Renal Disease
Normal renal function is important for the excretion of anesthetics and medications, maintaining fluid and acid-base balance, and regulating hemoglobin levels in the perioperative period.
Renal disease is quite prevalent in patients presenting for surgery and is associated with increased likelihood of poor postoperative outcomes. Even mild renal dysfunction is associated with a more likely risk of postoperative complications.
Multiple preoperative risk factors have also been identified that predict renal dysfunction in the postoperative period ( Box 28.1 ).
- •
Preexisting chronic kidney disease
- •
Advanced age
- •
Emergent surgery
- •
Liver disease
- •
High-risk surgery
- •
Body mass index > 32
- •
Peripheral vascular occlusive disease
- •
Chronic obstructive pulmonary disease
Renal Blood Flow
Although the kidneys represent only 0.5% of total body weight, their blood flow is equivalent to about 20% of cardiac output. Approximately two thirds of renal blood flow is distributed to the renal cortex. Renal blood flow and the glomerular filtration rate (GFR) remain relatively constant at renal arterial blood pressures in the range of 80 to 180 mm Hg ( Fig. 28.1 ). Being able to maintain renal blood flow at a constant rate independent of changes in perfusion pressure is known as autoregulation. It is achieved by adjustment of afferent arteriolar tone, which alters the resistance to blood flow. Autoregulation protects the glomerular capillaries from hypertension during acute hypertensive episodes and maintains GFR and renal tubule function during modest decreases in arterial blood pressure. When mean arterial blood pressure is outside the autoregulatory range, renal blood flow becomes pressure dependent. Autoregulation is reset by chronic hypertension and may be abolished in the diabetic kidney.

Renal blood flow is also strongly influenced by the activity of the sympathetic nervous system and by release of renin and other hormones. Sympathetic nervous system stimulation can produce renal vasoconstriction and a marked decrease in renal blood flow even if systemic blood pressure is within the autoregulatory range. Any decrease in renal blood flow will initiate the release of renin, which can further decrease renal blood flow.
Glomerular Filtration Rate
GFR reflects glomerular function and is a measure of the ability of the glomerular membrane to allow filtration. About 90% of the fluid filtered at the glomeruli is reabsorbed from renal tubules into peritubular capillaries and thus returned to the circulation ( Fig. 28.2 ). Normal GFR is approximately 125 mL/min and is very dependent on glomerular filtration pressure (GFP). GFP, in turn, is a function of renal artery pressure, afferent and efferent arteriolar tone, and glomerular oncotic pressure. Hydrostatic pressure within the glomerular capillaries is about 50 mm Hg. This pressure forces water and other low-molecular-weight substances such as electrolytes through the glomerular capillaries into Bowman space. Plasma oncotic pressure is about 25 mm Hg at the afferent arteriole and with filtration increases to about 35 mm Hg at the efferent arteriole. Despite a relatively low net filtration pressure, the glomerular capillaries are able to filter plasma at a rate equivalent to about 125 mL/min. GFR is reduced by significantly decreased mean arterial pressure or renal blood flow. Afferent arteriolar constriction decreases GFR by decreasing glomerular flow. Conversely, afferent arteriolar dilation and mild efferent vasoconstriction increase GFP and GFR.

Humoral Mediators of Renal Function
Renin-Angiotensin-Aldosterone System
Renin is a proteolytic enzyme secreted by the juxtaglomerular apparatus of the kidneys in response to (1) sympathetic nervous system stimulation, (2) decreased renal perfusion pressure, and (3) decreases in the delivery of sodium to the distal convoluted renal tubules. Renin acts on angiotensinogen (a circulating globulin in plasma) to form angiotensin I. Angiotensin I is converted in the lungs by angiotensin-converting enzyme to angiotensin II. Angiotensin II, a potent vasoconstrictor, stimulates the release of aldosterone from the adrenal cortex. It selectively increases efferent renal arteriolar tone at low levels and causes afferent arteriolar constriction at higher levels. Aldosterone, in turn, stimulates reabsorption of sodium and water in the distal tubule and collecting ducts.
Prostaglandins
Prostaglandins are produced in the renal medulla via the enzymes phospholipase A 2 and cyclooxygenase and released in response to sympathetic nervous system stimulation, hypotension, and increased levels of angiotensin II. During periods of hemodynamic instability, prostaglandins act to modulate the effects of arginine vasopressin (AVP), the renin-angiotensin system, and norepinephrine by vasodilating juxtamedullary vessels and maintaining cortical blood flow.
Arginine Vasopressin
Previously known as antidiuretic hormone, AVP regulates osmolality and diuresis. Although secreted in the supraoptic and paraventricular nuclei in the hypothalamus, it exerts significant effects on the renal collecting system. AVP actions are concentrated on collecting duct V 2 receptors to increase membrane permeability and facilitate water reabsorption. The overall effect of AVP is to decrease serum osmolality and increase urine osmolality.
Atrial Natriuretic Peptide
Atrial natriuretic peptide (ANP) is secreted when stretch receptors in the atria of the heart, and other organs, are stimulated by increased intravascular volume. ANP acts by relaxing vascular smooth muscle to cause vasodilation, inhibiting the renin-angiotensin system, and stimulating diuresis and natriuresis. The net effect of ANP is to decrease systemic blood pressure and intravascular volume.
Drug Clearance
Excretion of drugs or their metabolites into urine depends on three mechanisms: (1) glomerular filtration, (2) active secretion by the renal tubules, and (3) passive reabsorption by the tubules. The glomerular filtration of small molecules characteristic of anesthetic drugs depends on the GFR and the fractional plasma protein binding. Drugs that are highly protein bound will be inefficiently filtered at the glomerulus. Nonionized acidic and basic compounds undergo passive reabsorption by backdiffusion in the proximal and distal renal tubules. Ionized forms of these weak acids and bases, on the other hand, are trapped within renal tubules, accounting for increased renal elimination by either alkalinization or acidification of urine. Conjugation of drugs in the liver to water-soluble metabolites is another mechanism by which renal excretion of substances is achieved.
Renal Function Tests
Renal function can be evaluated preoperatively by using several laboratory tests ( Table 28.1 ). These tests are not sensitive measurements, and significant renal disease (more than a 50% decrease in renal function) can exist while laboratory values remain normal. Furthermore, the normal values established in healthy individuals may not be adjusted for age or applicable during anesthesia. Trends are more useful for evaluating renal function than a single laboratory measurement.
Test | Normal Value | Factors That Influence Interpretation |
---|---|---|
Test of Glomerular Filtration | ||
Blood urea nitrogen | 8-20 mg/dL | Dehydration Variable protein intake Gastrointestinal bleeding Catabolism |
Serum creatinine | 0.5-1.2 mg/dL | Age Skeletal muscle mass Catabolism |
Creatinine clearance | 120 mL/min | Accurate urine volume measurement |
Tests of Tubular Function | ||
Urine specific gravity Urine osmolality Urine sodium | 1.003-1.030 350-500 mOsm 20-40 mEq | All are affected by dehydration, solutes, filtrates, proteins, diuretics, dehydration, drugs, and extremes of age |
Serum Creatinine
Serum creatinine concentration, which reflects the balance between creatinine production by muscle and its renal excretion, is often used as a marker of GFR. In contrast to blood urea nitrogen (BUN) concentration, serum creatinine level is not influenced by protein metabolism or the rate of fluid flow through renal tubules. It is, however, influenced by skeletal muscle mass. Furthermore, increases in serum creatinine are not typically noted until GFR has declined by at least 50%. Thus, increased creatinine level may serve as a late marker of renal injury. For example, elderly patients, with known decreases in GFR, frequently display normal serum creatinine concentrations because of decreased creatinine production as a consequence of the decrease in skeletal muscle mass. Indeed, mild increases in the serum creatinine concentration in elderly patients may suggest significant renal disease. Likewise, in patients with chronic renal failure, serum creatinine concentrations may not accurately reflect the GFR because of (1) decreased creatinine production, (2) the presence of decreased skeletal muscle mass, or (3) nonrenal (gastrointestinal tract) excretion of creatinine. GFR can be estimated from serum creatinine by a variety of methods including the following formula:
GFR=(140−age)×weight(inkg)/(serumcreatinine×72)
Blood Urea Nitrogen
BUN concentrations, which are normally 10 to 20 mg/dL, vary with changes in GFR. The relationship between serum creatinine and BUN levels is particularly useful in diagnosing the cause of renal failure. Like serum creatinine, increases in BUN level are frequently a late sign of renal injury and are affected by dietary intake, coexisting illnesses, and intravascular fluid volume. For example, high-protein diets or gastrointestinal bleeding can increase the production of urea and thereby result in increased BUN concentrations (azotemia) despite a normal GFR. Other causes of increased BUN concentrations in the presence of normal GFR are increased catabolism during febrile illnesses and dehydration. Conversely, BUN concentrations can remain normal in the presence of low-protein diets despite decreases in GFR.
Increased BUN concentrations relative to serum creatinine in the presence of dehydration likely reflect increased urea absorption due to decreased urinary flow through the renal tubules, which results in a BUN-creatinine ratio more than 20. Although BUN concentration is susceptible to multiple extraneous influences, values more than 50 mg/dL inevitably reflect a decreased GFR.
Creatinine Clearance
Creatinine clearance (normal, 110 to 150 mL/min) is a measurement of the ability of the glomeruli to excrete creatinine into urine for a given serum creatinine concentration. Because clearance does not depend on corrections for age or the presence of a steady state, measurement of GFR is more reliable than the serum BUN or creatinine values. The principal disadvantage of this test, however, is the need for timed (2 hours may be as acceptable as 24 hours) urine collections. Creatinine clearance (CrCl) and by proxy GFR can be calculated from the formula
GFR=CrCl=Ucr×V/Pcr
Proteinuria
Small amounts of protein are normally filtered through glomerular capillaries and then reabsorbed in the proximal convoluted tubules. Proteinuria (excretion of more than 150 mg of protein per day) is most likely due to abnormally high filtration rather than impaired reabsorption by the renal tubules. Intermittent proteinuria occasionally occurs in healthy individuals when standing and disappears when supine. Other nonrenal causes of proteinuria include exercise, fever, and congestive heart failure.
Urine Indices
Measurement of urine osmolality and urinary sodium and calculation of the fractional excretion of sodium can help differentiate between prerenal and renal tubular causes of azotemia.
Newer Tests of Renal Function
Several new markers of renal function have recently been identified. Serum cystatin C, a ubiquitous protein that is exclusively excreted by glomerular filtration, is less influenced by variations in muscle mass and nutrition than creatinine. It may better predict risk of death and end-stage renal disease (ESRD) across diverse populations.
Other biomarkers such as N -acetyl-β- d -glucosaminidase, kidney injury molecule-1, and interleukin 18 are promising in the early detection of kidney injury. These biomarkers may have a role in the future in reducing morbidity and mortality rates associated with kidney injury in the perioperative setting.
Pharmacology of Diuretics
Thiazide Diuretics
Thiazide diuretics (hydrochlorothiazide, chlorthalidone) are generally administered for the treatment of essential hypertension and for mobilization of the edema fluid that is associated with renal, hepatic, or cardiac dysfunction. Diuresis occurs as a result of the inhibition of reabsorption of sodium and chloride ions from the early distal renal tubules. Side effects associated with diuretic-induced hypokalemia may include (1) skeletal muscle weakness, (2) increased risk for digitalis toxicity, and (3) enhancement of nondepolarizing neuromuscular blocking drugs ( Table 28.2 ).
Diuretic Class | Hypokalemic, Hypochloremic Metabolic Alkalosis | Hyperkalemia | Hyperglycemia |
---|---|---|---|
Thiazide diuretics | Yes | No | Yes |
Loop diuretics | Yes | No | Minimal |
Osmotic diuretics | No | No | No |
Aldosterone antagonists | No | Yes | No |
Loop Diuretics
Loop diuretics (ethacrynic acid, furosemide, bumetanide) inhibit the reabsorption of sodium and chloride and augment the secretion of potassium, primarily in the loop of Henle. Intravenous administration of these drugs produces a diuretic response within minutes. Chronic administration of loop diuretics may result in hypochloremic, hypokalemic metabolic alkalosis and, in rare instances, deafness.
Osmotic Diuretics
The most frequently administered osmotic diuretic is the six-carbon sugar mannitol. Mannitol produces diuresis because it is filtered by the glomeruli and not reabsorbed within the renal tubules. This leads to increased osmolarity of the renal tubule fluid and associated excretion of water.
Mannitol increases fluid movement from intracellular spaces into extracellular spaces such that intravascular fluid volume expands acutely. This redistribution of fluid from intracellular to extracellular compartments decreases brain size and intracranial pressure (also see Chapter 30 ). Mannitol may further diminish intracranial pressure by decreasing the rate of cerebrospinal fluid formation.
Aldosterone Antagonists
Spironolactone blocks the renal tubular effects of aldosterone and offsets the loss of potassium from administration of thiazide diuretics. Ascites and peripheral edema secondary to cirrhosis of the liver is often treated with spironolactone. The most serious toxic effect of spironolactone is hyperkalemia. Serum potassium concentration should be monitored closely in patients taking spironolactone.
Dopamine and Fenoldopam
Dopamine dilates renal arterioles via its agonist action at the DA1 receptor, leading to increased renal blood flow and GFR. Treatment with low-dose dopamine (0.5 to 3 μg/kg/min) may increase urine output but yet not alter the course of renal failure. In addition, dose-dependent side effects of dopamine include tachydysrhythmias, pulmonary shunting, and tissue ischemia (gastrointestinal tract, digits).
Fenoldopam, a dopamine analog, also possesses DA1 agonist activity but lacks the adrenergic activity of dopamine. It also increases renal blood flow and GFR, which may help the treatment of acute kidney injury. Yet its role in the treatment of renal failure is unclear. It is currently approved for short-term parenteral treatment of severe hypertension.
Pathophysiology of End-Stage Renal Disease
ESRD causes profound physiologic changes that affect several organs ( Box 28.2 and Table 28.3 ).
- •
Anemia
- •
Decreased ejection fraction
- •
Decreased platelet adhesiveness
- •
Hyperkalemia
- •
Unpredictable intravascular fluid volume
- •
Metabolic acidosis
- •
Systemic hypertension
- •
Pericardial effusion
- •
Decreased sympathetic nervous system activity

Full access? Get Clinical Tree
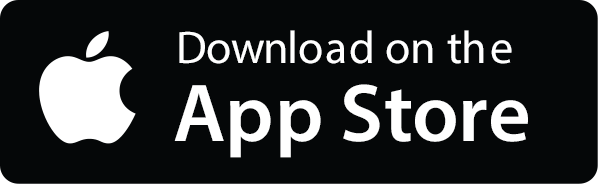
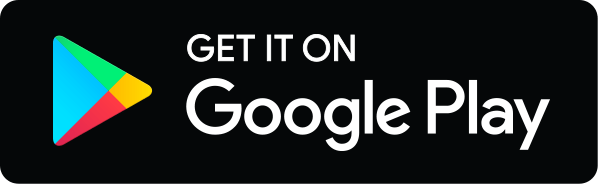
