Author
Number of institutions
Number cases
Complications
References
Murrell (1992)
Sydney, Australia 1
20
0
[33]
Van Niekerk (1990)
Utrecht, Netherlands 1
20
V 1
[146]
Bosenberg (1998, 2005)
South Africa 2
240,11,35
DP 1,C 1, V 1
ADARPEF (1994)
France, Belgium, Italy 38
43
0
[60]
Yamashita (1992–2002)
Japan 1
950
DP 7
[62]
Webster (1993)
Ontario, Canada1
18
V 2
[175]
Williams (1995)
Vermont 1
17 with spinal
0
[34]
Courreges (1996)
France 1
45
0
[67]
Tobias (1996)
Columbia, USA 1
25
0
[89]
Hasan (1994)
London 1
12
0
[65]
Vas (1999, 2001,2003)
Bombay 1
20
0
[66]
National UK audit (2010)
UK 21
529
C 1 DE 3
[58]
Frawley (2000)
Melbourne, Australia 1
50 with spinal
0
[233]
Somri (2007)
Israel 1
24 with spinal
0
[8]
Valairucha (2002)
Boston 1
115 caudal cath
A-1
[149]
Krishnan (2006)
Birmingham 1
20
0
[49]
Willschke (2007)
South Africa 1
85,20
0
[14]
Raghavan (2008)
Birmingham 1
22
0
[43]
Schenkman (2009)
Israel 1
44
V 5M1
[50]
Kost-Byerly (2002–2007)
Baltimore, USA 1
23
0
[152]
Bailey (2001–2002)
Philadelphia, USA 1
28 caudal cath
0
[183]
PRAN (2007–2010)
USA 8
72
DP 2
[5]
ADARPEF (2010)
France, Tunis, Quebec, Swiss, Belgium 45
46
DP 1
[60]
Willschke et al (2011)
Vienna 1
20
0
[7]
Total
~99 institutions
2594
DP11 DE 3 C2 V 9M 1
Risks and Benefits
Before implementing any new pain management strategy, the risks and benefits must be carefully evaluated to avoid putting the neonate at increased risk [1, 2]. Potential benefits of regional anesthesia must be weighed against the individual practitioner’s ability to perform the technique successfully as well as the ability of the healthcare staff on the ward or in the intensive care unit to manage continuous infusions of local anesthetics and/or opioids safely.
Benefits:
Surgically induced pain causes a spectrum of autonomic, hormonal metabolic, immunologic/inflammatory and neurobehavioral sequelae, many of which have associated detrimental effects [2, 16–22]. Acute pain can also have negative physiological consequences that include impairment of respiratory effort and systemic and pulmonary vasoconstriction that negatively influences compromised organ function [2, 15–19, 23].
In the late 1980s, Anand et al. first demonstrated that neonates, including preterm infants, are capable of mounting both hormonal and metabolic stresses in response to surgery [16]. They demonstrated that opioids inhibit the stress response to surgery. The stress response varies directly with the degree of surgical stress [2, 16], even after minor surgery. Moreover, severe stress may be pathological and could contribute to increased postoperative morbidity and mortality. Extreme catecholamine responses are associated with the worst outcome [2, 17]. Regional anesthesia appears to inhibit the hormonal stress response more effectively than opioids [18, 20, 21].
Neonates, and in particular preterm infants, exposed to the deleterious effects of pain are also at risk of impaired neurodevelopment and altered pain sensitivity [24–29]. Long-term effects may include emotional, behavioral and learning disabilities. In theory, regional anesthesia may avoid or, when used in combination with anesthesia, reduce the neurotoxicity associated with general anesthetics in neonates and young infants [26–30].
There are additional advantages to combining regional with general anesthesia [2, 4, 14, 31, 32]. Neonates, as a group, are at greater risk for adverse sequelae under general anesthesia given their immature organ systems (cardiovascular, central nervous, and respiratory) that are sensitive to the depressant effects of anesthetic agents. Neonatal myocardial function is particularly sensitive to both inhaled and intravenous anesthetics. When combined with general anesthesia, regional anesthesia provides profound analgesia with minimal hemodynamic effects, even in those with congenital heart disease [3, 32, 34–44]. A successful block allows reduced concentrations of inhalational agents to be used [3, 4, 32], thereby attenuating the severity of cardiovascular and respiratory depression [3] and may facilitate a faster recovery. Furthermore, inhalational agents have a reciprocal protective effect in that they increase the threshold of local anesthetic toxicity [45].
Regional anesthesia also reduces the requirement for muscle relaxants by providing motor relaxation. Neuraxial blockade facilitates the reduction of gastroschisis [43], omphalocele and diaphragmatic hernia [32, 41] by providing analgesia and relaxation of the abdominal musculature independent of the mode of ventilation [4, 6, 32, 34]. Caudal blocks have been used to reduce incarcerated inguinal herniae before surgery [40].
Neuraxial anesthesia may stimulate respiration and alter respiratory mechanics [46, 47]. The effects of neuraxial blockade on ventilation depend on the level and intensity of the block, as well as the clinical scenario. Neuraxial blockade may diminish abdominal and intercostal muscle activity, particularly in the compliant chest wall of neonates. On the other hand, it may improve diaphragmatic activity and excursion, thus offsetting a loss of accessory muscle function [33–35, 37]. The ventilatory response to CO2 is also improved, resulting in more efficient ventilation and maintenance of normocarbia [46, 47, 50, 51]. The pain relief provided by epidural analgesia improves ventilatory mechanics [34, 43, 47] and reduces the need for and duration of assisted or controlled ventilation after major abdominal or thoracic surgery [4, 34, 48–51]. As a consequence, ventilator-associated morbidity and mortality is reduced [41, 48–51].
Spinal anesthesia was reintroduced into pediatric anesthetic in the mid-1980s in an effort to reduce the respiratory complications, especially apnea, after surgery in preterm and ex-preterm infants. The impact on the outcomes from anesthesia was significant [6, 35, 52, 53]. As a result, spinal anesthesia and, more recently, caudal epidural analgesia have been advocated for high-risk neonates at risk for perioperative apnea after surgery [1, 3, 52]. The ex-preterm infants of today differ from those of the 1980s. Improvements in neonatal intensive care and ventilation strategies as well as surfactant have reduced the incidence and severity of bronchopulmonary dysplasia. Recent evidence investigating the perioperative risk of apnea in preterm infants anesthetized with the current inhalational agents (sevoflurane, desflurane) and remifentanil or receiving regional anesthesia for surgery failed to establish the superiority of one technique over the other [52, 54].
Regional anesthesia may have salutary effects on gastrointestinal function. It enhances the early return of gastrointestinal motility [44, 55, 56], particularly after gastroschisis repair [21, 43]. In necrotizing enterocolitis, the vasodilatory effects of autonomic blockade may improve splanchnic perfusion [32, 55], while opioids increase intestinal smooth muscle tone that may increase the risk of anastomotic leaks [55]. Lastly, oral feeding may resume earlier and speed recovery after minor surgery in the presence of a regional block [6, 34, 52, 53].
The immunosuppressive effect of regional anesthesia is attenuated compared with that reported with opioids [20, 21, 56]. Local anesthetics, but not opioids, stimulate natural killer cells, which play an important role in nonspecific cellular mediated and antitumor immunity [22, 56]. Local anesthetics (bupivacaine) also confer antimicrobial action and inhibit bacterial growth [56].
Lastly, the economic benefits of regional anesthesia include a reduction in the anesthetic costs, fewer days in the neonatal intensive care, earlier discharge, and more efficient use of the ward nurse’s time. However, to realize these benefits, the staff must be trained to care for neonates with epidural infusions and other regional blocks.
Risks:
The efficacy of regional anesthesia in neonates is not in dispute, but where opinions differ is in the ability to safely perform regional anesthesia in neonates [31]. Some consider the risks of regional anesthesia too great for routine use by individuals who do not have the requisite expertise [31, 44]. Although the risks associated with opioid and epidural analgesia in children are similar [57, 58], the risks associated with epidural analgesia and peripheral nerve blocks in neonates are less clear. The numbers of neonates in published surveys are relatively small in comparison with the numbers of children and adults [58–67]. The aggregate of published series from approximately 99 institutions yielded only one serious complication, meningitis, in the 2,594 published cases [50] (Table 15.1). Complications, as rare as they are, usually occur early “at the end of the needle,” i.e., when the anesthesiologist is still present. For example, the risk of a dural puncture is approximately 1:250, and convulsions 1:1,250 in neonates (Table 15.1). Every effort should be made to eliminate drug errors, a feature in the UK audit [58]. Anecdotal reports of spinal cord injuries bear testimony that these unfortunate disasters can occur, although infrequently [68]. It is generally recommended that neonatal epidural blocks should only be performed by those with the technical expertise despite the advent of ultrasound that may further reduce the risks [69].
Anatomical Considerations (Table 15.2)
Table 15.2
Important anatomical and physiological similarities and differences between neonate and adolescents (adults)
Anatomy | Neonate | Adolescents (adult) |
---|---|---|
Conus medullaris | L1–L2 | L1 |
Dural sac | S2–S4 | S2–S4 |
Intercristal line | L4 | L4 |
Vertebral column | Concave C1–L5 | Secondary curves |
Mainly cartilaginous | Ossified | |
Spinous processes | Lumbar more horizontal, parallel Orientation T10–T12 similar to lumbar Midline approach easy | Lumbar angled caudad All thoracic spines angled caudad |
Intervertebral space | Largest T12–L1; L5–S1 | |
Ligamentum flavum | Thinner, less dense | Thicker, fibrous |
Epidural space | 1–2 mm; less compliant Spongy gelatinous fat lobules | Compliant Densely packed lobules fibrous strands |
Sacrum | Flatter; less ossified | Fully ossified by 30 years |
Nerves | Thinner less myelination | |
CSF volume | 4 ml/kg | 2 ml/kg |
Physiology | ||
Blood pressure | Stable | Hypotension |
Pulse rate | Stable | Bradycardia |
Respiratory | Diaphragmatic function improved, ventilatory response CO2 enhanced | Similar |
CNS | Cortical arousal reduced Lower BIS | Similar |
Endocrine | Inhibition stress response | Similar |
GIT function | Earlier return | Similar |
Recent ultrasound studies have demonstrated that the conus medullaris (terminal end of the spinal cord) lies between L1 and L2 in the majority of neonates including preterm infants [14, 72]. The conus is not fixed but moves with changes in body position [73], although rarely does it extend beyond L3. A conus that extends caudally beyond L3 suggests a tethered cord [14, 72–75]. The dural sac usually terminates between S2 and S4, but maybe lie within millimeters of the sacral hiatus [14, 75].
The shape of the vertebral column develops over the first year of postnatal life. At birth, the vertebral column has a single shallow anteriorly concave curve extending from the C1 to L5. A secondary cervical curve appears when head control is achieved, usually by 6 months, and the lumbar curve develops with weight bearing, by ~1 year. In neonates, the spinous processes are parallel and horizontal facilitating a midline approach to the epidural space at all levels. The largest intervertebral spaces are found between T12–L1 and L5–S1, respectively.
The sacrum is narrower and flatter, ossification is incomplete, and the vertebrae are separate facilitating sacral intervertebral epidural blocks. Sacral dimples or pits may reflect an occult spina bifida, which should be excluded using ultrasound, CT or MRI before attempting a neuraxial block.
A posterior midline approach to the epidural space in neonates is regarded as the safest approach for several reasons. With a triangular spinal canal, the widest aspect of the epidural space is the midline where the epidural veins and arteries are less dense [75]. The epidural space is narrow (0.9–2.4 mm; median 1.5 mm) [14, 76] and less compliant, while the ligamentum flavum is thinner, is less dense and offers less resistance to the advancing epidural needle than in adults. Pressures generated during the passage of an epidural needle through the ligamentum flavum range from 35 to 105 mmHg (mean 70 mm) and the epidural pressures range from 1 to 10 mmHg [77].
Epidural fat consists of spongy gelatinous lobules with distinct spaces and offers minimal resistance to the passage of local anesthetic and an epidural catheter. The epidural veins have no valves and connect directly with intracranial veins. As a consequence, foreign material such as air or drugs inadvertently injected into the epidural veins can reach the brain without impediment.
The effective concentration of local anesthetics in neonates is less than in older children as the nerves in the former are thinner and less myelinated than those in the latter. The nerve trunks to the lower limbs are not fully myelinated until the second year of life. The degree of myelination influences the pharmacodynamic effects of local anesthetics.
The CSF volume in neonates <1.5 kg, 4 ml/kg, is relatively large compared with that in adults and older children, 2 ml/kg. CSF production in neonates, 0.35 ml/min, is also greater than that in adults. This explains, in part, why neonates require proportionately larger doses of local anesthetic for spinal block than older children.
In terms of peripheral nerve blocks in neonates, it is important to appreciate that the muscle layers of the thoracic and abdominal wall are thinner, less well defined, and more compliant than in older children. The sciatic nerve divides within the popliteal fossa [78], the ilioinguinal and iliohypogastric nerves lie 3–5 mm medial to the anterior superior iliac spine [79], and the musculocutaneous nerve is easily included in an axillary block because of its proximity to the divisions [80] (Fig. 15.1).


Fig. 15.1
Anatomical dissection of the axilla of a neonatal cadaver demonstrating the close relationship of the axillary artery and the cords and branches of the brachial plexus. The musculocutaneous branch is easily included even with small volumes of local anesthetic. Brachial plexus (j) and related structures within the axilla and at the root of the neck. Structures include the: (a) pectoralis major and (b) pectoralis minor muscles, (c) coracoid process, (d) coracobrachialis muscle, (e) axillary artery, (f) anterior and (g) middle scalene muscles, (h) common carotid artery, (i) vagus nerve, (k) clavicle
Pharmacological Differences
The pharmacological differences in neonates vary with gestational age-related changes in body fluid compartments, plasma protein concentrations, distribution of cardiac output and the functional maturity of liver and kidneys [81]. Other contributing factors include less body fat (15 % body weight) and skeletal mass (25 % body weight), a proportionally larger brain and liver and greater cardiac output and regional blood flow to vessel-rich organs resulting in more rapid uptake of drugs.
Neonates are at greater risk of drug toxicity than older children [2, 82]. Albumen and α1-acid glycoprotein concentrations in neonates are less than those in children [82–90], thus increasing the free fraction of the circulating drugs. Since local anesthetics are basic drugs, the reduced concentration of α1-acid glycoprotein increases the free fraction of local anesthetics in blood. However, α1-acid glycoprotein is an acute phase protein and, as such, increases during acute illnesses and with surgical stress [83, 86]. This latter effect offsets the reduced concentration of α1-acid glycoprotein in neonates, offering some protective effect by attenuating the free fraction of the local anesthetic. In addition, a greater fraction of local anesthetic is excreted unchanged in the urine because of the reduced hepatic blood flow and immature cytochrome P450 (CYP) enzyme system [83, 88]. Plasma cholinesterase activity in neonates is 50 % that in adults, slowing the clearance of ester local anesthetics such as chloroprocaine, from the blood. On balance, chloroprocaine is viewed as a safer local anesthetic in neonates than the amide anesthetics because its metabolism depends on only one enzyme [2, 35, 89].
Vascularity of the injection site and regional blood flow influence the rate of uptake of local anesthetics. The rate of absorption and therefore the peak blood concentration is reduced by the addition of a vasoconstrictor. Epinephrine prolongs the duration of action of local anesthetics [91, 92] by up to 50 % in neonates [6, 91–93]. In the author’s experience the duration of caudal bupivacaine is virtually doubled by the addition of epinephrine 1:400,000 (unpublished data). However, during continuous epidural infusions, the initial effect of epinephrine to prolong the duration of the block is minimal after 3 h [94]. Hence, epinephrine is recommended for one-shot caudal epidural blocks and not for continuous infusions.
Lung function also plays an important role in modulating the duration of action of local anesthetics. Approximately 60–80 % of an intravenous bolus of lidocaine is absorbed on the first pass through the lungs. However, in neonates with right to left intracardiac shunts, the reduced uptake of local anesthetic by the lungs may increase the peak blood concentration by 100 % and, with it, the risk of local anesthetic toxicity [95].
Little is known about the pharmacokinetics of longer-acting local anesthetics in neonates [2, 83–85, 88, 96]. Using anecdotal reports of toxicity after bupivacaine, the recommended caudal/epidural infusion rates for bupivacaine for postoperative analgesia is 0.2 mg/kg/ h for neonates and infants less than 6 months of age and up to 0.4 mg/kg/h for infants >6 months of age. The plasma concentrations recorded in neonates were greater than those in infants, although the concentrations in both age groups were <2–3 μg/ml the purported threshold for toxicity in humans [83, 88, 96]. The plasma concentrations of bound bupivacaine accumulate after a 48 h infusion in neonates and infants [96], whereas the concentrations of bound ropivacaine do not accumulate after infusions up to 72 h in duration [83]. Thus, ropivacaine appears to be the safer local anesthetic for epidural infusions lasting 48–72 h in neonates [88, 89, 96].
Neuraxial Blockade
Spinal
Bainbridge described the first spinal anesthetic performed on an infant in 1899, and early in the twentieth century, Lord H Tyrell Gray suggested that spinal anesthesia “would occupy an important place in the surgery of children in the future.” Although the popularity of spinal anesthesia waned as the safety of general anesthesia improved, these prophetic words may still be realized considering the current controversy regarding the neurotoxicity of general anesthetics in neonates. The popularity of spinal anesthesia was rekindled in the early 1980s when Abaijan proposed its use for ex-preterm infants undergoing inguinal hernia repair [6]. Currently, spinal anesthesia remains limited to selected high-risk infants in whom general anesthesia may pose a major risk [97].
Spinal anesthesia has been used alone or in combination with an epidural for a wide variety of surgeries including inguinal hernia repair, ligation of patent ductus arteriosus, pyloromyotomy, gastrostomy, gastroschisis [98], omphalocele, exploratory laparotomy, lower abdominal surgery (colostomy, anoplasty, rectal biopsy, circumcision) meningomyelocele repair or orthopedic surgery [6, 99, 100].
The duration of action of spinal anesthesia in neonates is much less than it is in adults, despite the relatively larger doses used in the former. The duration of action appears to depend directly on age [93, 101, 102]. For practical purposes, an effective plane of surgical anesthesia after spinal block lasts up to ~40–60 min with bupivacaine, levobupivacaine [103, 104], and ropivacaine [104, 105]; up to 1.5 h with tetracaine plain, or 2 h with tetracaine with epinephrine [6, 93]; and up to 1 h after lidocaine 3 mg/kg with epinephrine [93]. Spinal anesthesia can be used to facilitate placement of an epidural block [8] in order to prolong the duration [8, 9]. Intrathecal clonidine (1 μg/kg) may also be used to perform surgery, although it is associated with more sedation and apnea than the local anesthetics [101, 103].
Spinal anesthesia rarely produces significant changes in heart rate or blood pressure in neonates, even with blockade to thoracic levels [6, 100]. Reduced cortical arousal caused by peripheral deafferentation [44, 106] or a decrease in cerebral blood flow [107] should be born in mind when additional sedatives are used [106]. The incidence of postoperative apnea in preterm neonates who received spinal anesthesia was less than that after general anesthesia, provided sedatives (e.g., midazolam 0.2 mg/kg, propofol 1 mg/kg) were avoided [6, 100, 108, 109].
Complications:
Based on two large series [6, 100], the incidence of serious complications (nerve injury, meningitis, arachnoiditis) [110, 111] after spinal anesthesia is rare in neonates, but greater in neonates than older infants and children [109]. Failure rates for effective spinal anesthesia in neonates range from 5 % (in experienced hands) [6] to 17 % (trainees) [6, 31, 108] with a bloody tap rate of 10 % [101]. Bradycardia (<100 bpm) and apnea can be treated with tactile stimulation, atropine 0.1 mg/kg, or ventilatory support as indicated. The incidence of bradycardia ranges from 1.2 to 1.8 % [6, 100]. The incidence of high spinal blockade (0.1–0.6 %), heralded by apnea but usually not associated with hypotension or bradycardia, has been associated with the administration of large doses of local anesthetics and early elevation of the legs when applying the electrocautery pad to the back or “top-ups” when the level is inadequate. Unilateral spinal blockade in neonates has also been described [112]. Blood plasma concentrations of bupivacaine after spinal administration are small (0.2–0.3 mcg/ml) and unaffected by the addition of epinephrine [113].
Technique:
Using a sterile technique, a spinal anesthetic may be placed using a 25 ga or styletted 22 ga 1.5 in. spinal needle in the sitting or lateral decubitus position. Chlorhexidine in 70 % alcohol is currently recommended for skin preparation. The antiseptic should completely dry before inserting the spinal needle to preclude transfer of alcohol to the subarachnoid space. Spinal anesthesia is usually placed at L3–L4 or L4–L5. A prior ultrasound scan is useful to determine the exact location of the dural sac and to exclude any central nervous system or bony anomalies. Once free flow of CSF is obtained through the spinal needle, the local anesthetic can be administered using a 1 ml syringe. The onset of the block is reflected by profound motor block in the lower extremities within seconds of completing the delivery of local anesthetic. Care should be taken to avoid positioning the infant head down, i.e., when applying the electrocautery pad on the back, before the block height has been set to avoid a high spinal block. Instead, the neonate should be logrolled to apply monitors and other devices.
If the neonates requires placating gentle stroking, soothing or dextrose water on a pacifier is effective [114]. Intravenous sedation may be necessary in ~25 % of cases [6, 100], but it does increase the risk of perioperative apnea [100].
Dose Guidelines
Hyperbaric tetracaine 0.5 %; 0.6–1 mg/kg
Isobaric bupivacaine or ropivacaine 0.5 %; 0.6–1 mg/kg
Hyperbaric lidocaine 3 mg/kg
Adjuvants
Epinephrine 5–10 mcg/kg prolongs the duration of action.
Clonidine 1 mcg/kg prolongs analgesia [102].
Caudal Block
Caudal analgesia is frequently used to provide analgesia for surgery below the umbilicus [39, 70, 71, 115]. The popularity of caudal blockade stems from its simplicity, safety and efficacy and is usually performed in combination with general anesthesia [5]. Larger doses are required for upper abdominal surgery [116], but achieving this level of blockade is less predictable unless a caudal catheter is introduced. Caudal blocks are effective as the sole anesthetic, particularly for ex-preterm infants undergoing inguinal hernia repair [117]. They have also been used to reduce incarcerated inguinal herniae [40], to improve compromised perfusion after umbilical catheterization [118] and penile block [119] and to facilitate PICC line placement in extreme preterm infants [120].
Anatomy:
The sacral hiatus is between the sacral cornu, two prominences that represent the remnants of the fifth sacral arch, and extends to the fused arch of the fourth sacral vertebra. The sacrococcygeal membrane, an extension of the ligamentum flavum, covers the sacral hiatus separating the caudal space from the subcutaneous tissue. Considerable variation in the sacral hiatal anatomy exists mainly due to incomplete posterior fusion of other sacral vertebrae. However, a few important surface landmarks can be used to enhance successful placement in both normal and abnormal sacra. The sacral hiatus virtually always lies at the apex of an inverted equilateral triangle whose base is a line between the posterior superior iliac spines (Figs. 15.2 and 15.3). The intersection of a line drawn from the patella through the greater trochanter (with the hips flexed at 90°) with a line drawn down the vertebral column is another useful landmark (Fig. 15.3).



Fig. 15.2
Caudal block. The sacral hiatus lies at the apex of an equilateral triangle with the line drawn between the posterior superior iliac spines as the base

Fig. 15.3
Caudal block. The knee-chest position may be used to facilitate placement of the caudal in awake neonates
Technique:
Caudal block can be performed in the lateral decubitus position or prone knee-chest position—a useful position for an “awake” caudal block [121] (Fig. 15.2). Under sterile conditions a short-beveled needle, held between the thumb and index finger, is introduced at approximately 30–45° to the skin (i.e., with the bevel parallel to the skin) with the skin between the sacral cornua held taut by the thumb and index finger of the opposite hand. The needle is then advanced until it pierces the sacrococcygeal ligament [122] and a “give” is felt. The needle is now in the caudal space, which can be confirmed by a loss of resistance. If the needle tip is extremely sharp, a “give” may not be felt; hence, most prefer to use a needle that is not extremely sharp. Penetration of the sacrococcygeal membrane just above a line between the sacral cornua carries a smaller incidence of bloody tap in the author’s experience. The dural sac lies within 5–10 mm of the sacral hiatus in the majority of neonates [14], and thus changing the angle and advancing the needle, as described in adults, is unnecessary as it may result in a dural puncture or bloody tap [38]. Using a 22 g IV cannula is popular [38, 123], but in the author’s experience, it carries a greater incidence of failure (subcutaneous injection) and bloody taps (see below).
After negative aspiration for blood and CSF, the required volume of local anesthetic can be injected. Aspiration should be gentle since strong negative pressure may cause the low-pressure epidural vessels to collapse before a positive aspiration test can be elicited [38]. In the event of a “bloody tap,” the needle should be redirected or removed and reinserted more cephalad. Local anesthetic injection should proceed with caution after a bloody tap considering the greater risk of an intravascular injection under these circumstances [124]. Ultrasound can be used to “visualize” the spread within the caudal epidural space [125].
To provide a diverging opinion, the editor has always used a 22 g IV cannula for caudal block in neonates. In this approach, the skin is nicked with a “dull” needle down to the level of the subcutaneous tissue to introduce the IV catheter/needle into the subcutaneous tissue. This should minimize the risk of translocating epidermal tissue to the caudal space when the catheter/needle is advanced through an intact epidermis (see Complications below). The IV catheter/needle is then advanced through the nick. When the sacrococcygeal ligament is pierced, the catheter/needle is advanced 2–3 mm further, and the needle is transfixed while the catheter is slid off into the caudal canal. If the needle had inadvertently pierced the bone of the sacrum during insertion, the catheter would accordion rather than thread smoothly when it was advanced. This should ensure an inadvertent osseous cannulation did not occur. Once the needle is withdrawn, the remaining catheter is observed for either blood or clear CSF flashback. The editor does not aspirate the catheter at any time because if the catheter had pierced a vein, the vein would collapse when the catheter was aspirated and no blood will be collected. If the catheter were in the bone, again nothing will be returned during aspiration. Lastly, if the catheter were in the subarachnoid space and the sac punctured, CSF will flow back immediately upon withdrawal of the needle, before the catheter was aspirated. To ensure local anesthetic is injected into the caudal space and not subcutaneously, as the operator begins to inject local anesthetic into the catheter, the index finger of the free hand is gently placed over the sacrococcygeal ligament to detect a subcutaneous injection [99]. Although much debate has occurred with regard to the use of a test dose of drug and the determination of the location of the tip of the catheter, the editor treats the entire dose of local anesthetic to be administered (usually 1 mL/kg of 0.125 % bupivacaine) as a test dose, infusing 1 mL at a time while monitoring the ECG for 30–60 s before continuing.
Complications:
The incidence of all complications after caudal/epidural blocks in neonates is threefold greater than in infants and eightfold greater than in children [126]. The two most common complications reported were a local skin infection and drug error [127]. Dural puncture and subsequent injection may lead to a total spinal and respiratory arrest (apnea). Systemic toxicity may be heralded by EKG (ST segment elevation and peaked T waves) changes, arrhythmia, cardiovascular collapse or convulsions after accidental intravascular or sacral intraosseous injection (incidence 0.4 %) [128]. Intrapelvic, intraosseous, and intravascular injections [129, 130] are very rare with proper technique. Urinary retention is not a substantive concern in neonates. Nerve injury and neurological deficits have not been reported in neonates. Inclusion dermoid tumors have been reported, but only anecdotally. The risk of introducing nucleated epidermal cells from stratum spinosum during caudal block is small and is similar with 22 g hollow needles and styletted 22 g caudal block needles [131–133].
Dosage:
Many formulae have been proposed for the volume of local anesthetic required for a caudal block based on the neonate’s weight, age, and length [115, 124, 134–137]. The most practical is that suggested by Armitage [124]:
0.5 ml/kg of local anesthetic for sacrolumbar dermatomes
1.0 ml/kg for lumbar thoracic dermatomes (subumbilical)
1.25 ml/kg for mid-thoracic dermatomes (upper abdominal)
Bupivacaine 0.125–0.25 % [138], ropivacaine 0.1–0.2 % [139–143], levobupivacaine 0.25 % [142], and chloroprocaine 3 % [35, 89] are effective. The duration of analgesia depends upon the dose and specific local anesthetic administered, the use of epinephrine, the site of surgery and whether a continuous catheter is used [35, 71]. Increasing the concentration of local anesthetic does not offer additional advantage but may increase the incidence of side effects (e.g., motor blockade) and/or complications. Clonidine (1 μg/kg) has been used to prolong the duration of analgesia about several hours [144] but is associated with an increased risk of sedation and apnea, particularly with a dose of 2 μg/kg [145].
Caudal Catheter Techniques
A catheter can be introduced via the sacral hiatus in neonates to prolong the duration of caudal block [9, 35] and to access the sacral, lumbar, or thoracic nerve roots [4, 146–160]. This technique was developed before the introduction of pediatric epidural needles. Specialized equipment is not required [4], and the risk of dural puncture or spinal cord injury may be less than with lumbar epidural placement in less-experienced hands [4, 147].
Technique:
An 18 or 20G IV cannula, Crawford needle or specifically designed kits [147, 148] can be used to access the caudal space. A 20–24G epidural catheter that passes easily through the cannula is measured against the neonate’s back to the dermatome level of the planned surgical incision. This predetermined length can then be introduced gently into the caudal/epidural space (Fig. 15.4). Flexion or extension of the infant’s spine [4, 50], flushing the catheter with saline [4, 147] or twisting/rolling the catheter in the operator’s fingers [4, 50] can be used to advance the catheter. Thin (24G) flexible catheters may curl in the sacrolumbar epidural space and fail to reach their target dermatome. This problem can be overcome by using styletted catheters, although they can be expensive [152, 154, 155]. Attempts to feed the catheter against resistance are potentially harmful. It is important not to force the catheter should resistance be encountered, as the catheter tip may impinge on a nerve root or puncture a blood vessel rather than advance. It may also puncture the dura and pass up the subdural or subarachnoid space. Instead of forcing a catheter to advance, it may be necessary to administer larger volumes of local anesthetic to achieve the desired level of blockade. The choice between single- and multi-orifice catheters is moot, since the latter function as single orifice catheters (perfusing the proximal orifice in all instances) at the small infusion rates used in epidural infusions [156].


Fig. 15.4
A predetermined length of catheter can be introduced into the epidural space to the desired level via the sacral hiatus. An epidurogram or ultrasound can be used to confirm placement. In this epidurogram, contrast filled the caudal catheter, which was introduced at the sacral hiatus (lower arrow), and threaded up to the L1 level (upper arrow)
Accurate placement of epidural catheters depends on the facilities available. Several techniques including epidurography [4, 146, 149, 161], fluoroscopy, electrocardiography [154, 155, 162], nerve stimulation [159, 160], and ultrasonography [125, 150, 157, 163] may be used. Electrical stimulation with wired epidural catheters allows real-time adjustment of the catheter level but requires specifically designed equipment. In the author’s experience of more than 500 infants, 20 g nylon catheters (Portex®) may be threaded to within one vertebral body of the preselected level in the epidural space. Difficulties have been described reaching the desired dermatome level in preterm infants and when a Tuohy needle is used [9, 146]. The curve of a Tuohy needle may cause the catheter to curl if the lumen is not properly aligned within the caudal space.
Complications:
These include failure to position the catheter at the desired level, dural puncture, intravascular placement [50, 153], bacterial colonization of the catheter tip (20–30 %) [99, 164, 165], and one reported case of meningitis [50]. Careful aseptic technique with chlorhexidine, rather than Proviodine, for all catheter techniques carries a lower risk of colonization [166]. However, colonization has not been shown to progress to abscess formation in the central nervous system. Tunneling catheters subcutaneously away from the diaper area may further reduce the risk of prolonged infusions [164, 165].
Dosage:
For single dosing, 0.5–0.75 ml/kg 0.25 % bupivacaine or 0.2 % ropivacaine depending on the number of dermatomes required to be blocked [4].
Since neonates are not ambulatory, these concentrations will not impair discharge because of lower extremity weakness. Previous studies in children demonstrated that for 0.175 % bupivacaine with epinephrine, 0.7–1.3 ml/kg provided similar analgesia and discharge times after inguinal hernia surgery [167]. For ropivacaine, 1 ml/kg of a 0.25 % solution achieved a block to T11 with a time to first analgesia of 6 h. However, a 0.15 % solution of ropivacaine in a volume of 1.5 ml/kg achieved a block to T7 and time to first analgesia of 9 h [168].
For continuous epidural infusions for postoperative pain, 0.2–0.25 mg/kg/h bupivacaine is recommended to prevent toxic blood concentrations for 48 h [96]. This may be administered as 0.2 ml/kg/h of a 0.1 % bupivacaine solution. Ropivacaine dosing should be 0.2 mg/kg/h of a 0.1 % solution. In contrast to bupivacaine, ropivacaine does not accumulate as the duration of infusion increases [23].
Lumbar and Thoracic Epidural
Lumbar epidural is indicated for lower abdominal, pelvic and lower limb surgery, whereas a thoracic epidural is indicated for upper abdominal or thoracic surgery [169], particularly in poor-risk patients with respiratory disabilities [170]. Experience with these epidural techniques in neonates is limited [32, 50, 51, 58, 83, 171]. Few dermatomes are involved in the transverse abdominal incision favored by pediatric surgeons and thus can be easily covered by an accurately placed epidural. Epidural placement is usually performed in an anesthetized child, although it can also be performed with sedation [7] or after initial spinal blockade when indicated [8]. In view of the potential risk of spinal cord trauma, thoracic epidurals should only be performed by experienced providers familiar with epidurals in neonates.
Technique:
Using a sterile technique, the skin should be punctured to facilitate smooth insertion of a 19 or 20 g Tuohy needle. A midline approach is preferred since the epidural space is widest at this point and the epidural vessels less dense. The interspace chosen should be as close to the dermatome of the surgical incision as possible. The needle angulation depends on the level of epidural puncture and is greatest in the mid-thoracic region. Below this level, a more perpendicular approach can be used since the spinous processes in the lumbar region are almost horizontal when the back is flexed [172, 173] (Fig. 15.5). T12–L1 and L1–L2 interspaces are the largest and most easily palpable. The skin-epidural distance ranges from 5 to 12 mm depending on the gestational age and weight [32]. Ultrasound can be used to measure this distance [14].


Fig. 15.5
The epidural needle can be introduced almost vertically to the skin in neonates when the back is flexed in the lateral decubitus position. Loss of resistance to air is considered more sensitive for detecting the 1–2 mm wide epidural space
Both air and saline have been advocated for the loss of resistance test to identify the epidural space [173, 174]. Saline is more popular according to a recent survey [174], although air is perhaps more sensitive [32, 172]. Using air for loss of resistance often results in the injection of air into the epidural space, and this maneuvre could cause an air embolus or rarely intra-arterial air embolus, specifically in the artery of Adamkiewicz, leading to paralysis. A “drip and tube” method has also been used successfully [62].
A catheter should be introduced at least 2 cm into the epidural space for continuous infusion or intermittent “top-ups” depending on whether an open tip or closed tip catheter is used [156]. This is best done after the “test dose” has “opened” the epidural space to facilitate the passage of the catheter [4, 147]. The length of catheter introduced into the epidural space is important: too much length increases the risk of a unilateral blockade, whereas too little could cause a failed block or increased local anesthetic leakage. Ultrasound can confirm correct catheter placement [157].

Full access? Get Clinical Tree
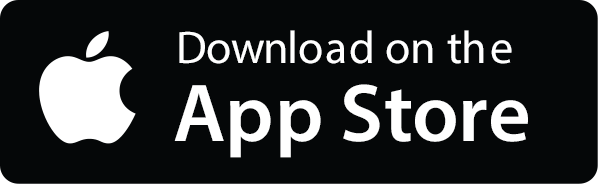
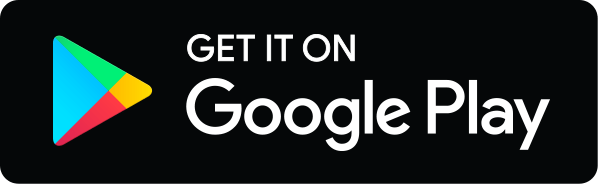