© Springer International Publishing AG 2017
Giovanni Landoni, Laura Ruggeri and Alberto Zangrillo (eds.)Reducing Mortality in the Perioperative Period10.1007/978-3-319-46696-5_1515. Reducing Mortality in the Perioperative Period: Remote Ischemic Preconditioning
(1)
Department of Critical Care Medicine, University of Pittsburgh, School of Medicine, Pittsburgh, PA, USA
15.1 Introduction
Despite the upsurge of publications on ischemic preconditioning in recent years, the concept of preconditioning an organ with ischemia is not new. In 1986, Murry et al. demonstrated short periods of regional ischemia and reperfusion resulting in protection against necrosis to a later longer period of ischemia in the canine myocardium [1]. In reperfusion injury following a brief period of ischemia, tissues begin to adapt to anaerobic metabolism. Restoration of blood flow can lead to an oxygen supply that exceeds tissue requirements, the activation of macrophages, and the generation of reactive oxygen species [2]. This can ultimately result in endothelial injury and further release of pro-inflammatory cytokines [3]. Ischemic preconditioning occurs when a tissue undergoes brief periods of ischemia to later protect against longer ischemic events and reperfusion injury.
The protection conferred by brief episodes of ischemia and reperfusion to a later more sustained episode of ischemia occurs in organs other than the heart, such as the kidneys and the brain. In 1985, Zager et al. reported that rats exposed to 15 min of bilateral renal artery occlusion had improved renal function when compared to a control group of rats after exposure to a second ischemic insult 30 min later [4]. In mice Joo et al. performed right nephrectomies and ischemic preconditioning by 5-min episodes of left-sided renal ischemia followed by reperfusion [5]. When the mice were later subjected to a more prolonged ischemic event, serum creatinine levels in the mice that underwent ischemic preconditioning were significantly lower when compared to a control group of mice who had just received unilateral nephrectomy [5]. Kitagawa and colleagues introduced the concept of “ischemic tolerance” in the brain when they introduced cerebral ischemia in gerbils by occluding both common carotid arteries [6]. Two-minute ischemic treatments performed daily for 2 days leading up to a 5-min cerebral ischemic period provided protection against neuronal death [6].
Remote ischemic preconditioning (RIPC) was first described in the literature in 1993 with an experiment in dogs where occlusion of the circumflex artery protected the myocardium supplied by the left anterior descending artery (LAD) [7]. When infarct sizes of the LAD were evaluated after 1 h of sustained LAD occlusion by triphenyltetrazolium staining, the infarct size of the preconditioned group was significantly less than the control group [7]. Since that time, numerous studies have been published on the clinical use of RIPC whereby a brief ischemic insult is provided to one area of the body to induce protection to a longer ischemic insult at a remote site. This chapter will review the most commonly discussed mechanisms for RIPC as well as the more recent clinical studies done using RIPC as they pertain to reducing morbidity and mortality in the perioperative period [8, 9].
15.2 Proposed Mechanisms of Remote Ischemic Preconditioning
15.2.1 Humoral Mechanism
The process by which RIPC occurs is complex and not fully understood. There have been numerous proposed mechanisms in the literature. The hypothesis that the RIPC event is triggered by a humoral mediator has been investigated. Dickson et al. provides evidence of the involvement of humoral mediators for eliciting RIPC by showing that a rabbit could be preconditioned by transfer of coronary effluent [10]. Effluent was collected during normal perfusion from donor hearts and during ischemia-reperfusion from donor preconditioned hearts. The effluent was then transferred to acceptor control and acceptor preconditioned hearts. All hearts were subject to 40 min of ischemia [10]. The resulting mean infarct size was smaller in the donor and acceptor preconditioned hearts [10]. There was an increase in adenosine and norepinephrine in the effluent from the preconditioned animals [10]. These results support the release of a hormonal trigger signal that is given off from the preconditioned myocardium and that when delivered to an acceptor heart evokes a cardioprotective effect. Some of the common mediators that have been studied include adenosine, catecholamine, bradykinin, and opioids [11–14].
In a recent review, Zarbock and Kellum discuss that kidney protection with RIPC occurs through the release of damage-associated molecular patterns (DAMPs) [15]. Increased levels of high-mobility group box 1 (HMGB-1), a prototypical DAMP, after RIPC were associated with a lower risk of AKI in an investigation discussed in more detail later in this chapter (OR 0.75, CI 0.35–0.94, p = 0.03) [16]. It is possible that DAMPs released from an initial location of ischemia-reperfusion travel to a target organ. In this case DAMPs may be filtered by the kidney and, through pattern-recognition receptors in the proximal tubular epithelia, signal renal protective mechanisms [15].
15.2.2 Neural Pathway
The potential for a neural pathway of communication to a target organ has been shown. Pretreatment with hexamethonium, a ganglion blocker, negated remote cardioprotection in rats receiving 15 min of mesenteric artery occlusion [17]. In humans endothelial injury caused by arm ischemia and reperfusion was measured with a reduction in flow-mediated dilation. The protective effect of RIPC prior to injury was reduced with the infusion of trimetaphan, another ganglion blocker [18]. In rabbits, vagal nerve ligation and atropine administration negated RIPC-induced reduction in myocardial infarct size [19].
15.2.3 The Final Common Event
The final common event in the protection induced by RIPC most commonly cited in the literature involves intracellular kinases acting on the mitochondria causing a closure of the mitochondrial transition pore, preventing the influx of ions [20]. Three main pathways acting on the mitochondrion have been proposed: (i) the reperfusion injury salvage pathway [21], (ii) the cyclic guanosine monophosphate/CGMP-dependent protein kinase pathway [22], and (iii) the survivor activating factor enhancement pathway [23]. The potassium-dependent adenosine triphosphate (ATP) channel blocker glibenclamide was shown to block the benefit of RIPC indicating that the protection may depend on potassium-dependent ATP channel activation [24]. Thus, it is proposed that in RIPC the potassium-dependent ATP channel is activated, leading to closure of the mitochondrial transition pore, reducing mitochondrial permeability in a target organ, and slowing the rate of ATP depletion [25].
15.3 Clinical Studies on Remote Ischemic Preconditioning
The majority of clinical studies describe the application of a blood pressure to the arm or leg to induce RIPC. Generally the cuff is inflated to 200 mmHg or 50 mmHg greater than the systolic atrial pressure and then deflated. This procedure is then repeated three to five times. The majority of clinical studies using RIPC have been done on the cardiothoracic patient population prior to cardiopulmonary bypass. Most studies report the effect of cardiac biomarkers in patients who receive RIPC when compared to a control group of patients [26–39]. For example, one of the initial studies to demonstrate the effect of RIPC on troponin T levels randomized 57 adult patients prior to coronary bypass grafting to receive RIPC through the use of timed arm blood pressure cuff inflations or to a control group [27]. When troponin T was measured prior to surgery and at time points after surgery, RIPC decreased the total area under the curve of troponin T by 43 % when compared to controls [27]. In 37 children undergoing congenital heart defect repair, Cheung et al. reported lower troponin I levels, airway resistance, and postoperative need for ionotropic medications for patients who received preoperative RIPC when compared to children who did not receive RIPC [26]. Regarding cardiac outcomes in both children and adults in the perioperative period, there have been discrepant findings with some studies showing a benefit to RIPC [26–29, 33] and other showing no benefit [31, 34, 36–39].
Additionally, the effect of RIPC on kidney outcomes has been studied in both the adult and pediatric cardiac and vascular surgery populations. The association of surgical procedures and AKI has been consistently shown [40–42]. When 82 adult patients were randomized to receive abdominal aortic aneurysm repair with either RIPC by intermittent cross clamping of the common iliac artery for 10 min followed by 10 min of reperfusion or no RIPC prior to surgery, RIPC was found to reduce the incidence of myocardial injury by 27 % and renal impairment by 23 % [43]. When AKI was defined as a rise in serum creatinine ≥0.3 mg/dL or ≥50 % within 48 h after cardiac surgery where cardiopulmonary bypass was expected, a 27 % absolute risk reduction in AKI was found when comparing a randomized group of patients who received RIPC to those who received no intervention prior to surgery [44]. However, there have been investigations that have not reported a protective effect of RIPC for AKI [45–48].
Given the differences in study results, it may be that different patient characteristics make an individual more or less likely to respond to RIPC. For example, it may be those patients at a greater risk for AKI that will be more likely show a beneficial effect of the intervention. In a recent study, 240 adult patients at very high risk for AKI (Cleveland Clinic Foundation scores ≥6 [49]) undergoing cardiac surgery were randomized to RIPC with upper arm blood pressure cuff inflation compared to a control group [16]. There was a 15 % absolute risk reduction (95 % CI 2.56–27.44 %, p = 0.02) for those who received RIPC when compared to those who did not [16]. A unique feature of this study was the use of urinary biomarkers of AKI, tissue inhibitor of metalloproteinase-2 (TIMP-2), and insulin-like growth factor-binding protein 7 (IGFBP7), which increased in the majority of patients who are receiving RIPC [16]. Furthermore, in those who experienced an increase in TIMP-2 and IGFBP7 after RIPC and prior to cardiopulmonary bypass, the incidence of AKI was reduced when compared to those who did not [16]. Also, higher levels of HMGB-1 after RIPC, discussed earlier in this chapter, were associated with a reduction in AKI [16].
The use of RIPC for neurologic as well was pulmonary protection prior to surgical procedures has been explored. Patients undergoing elective carotid endarterectomy (CEA) were randomized to receive either RIPC with 10 min of lower limb ischemia followed by reperfusion or no RIPC prior to CEA [50]. There were less saccadic latency deteriorations in the patients who received RIPC; however, this did not reach statistical significance (32 % versus 53 %, p = 0.11) [50]. Patients undergoing elective thoracic pulmonary resection (N = 216) were randomized to either RIPC or a sham procedure [51]. Compared to the control group, the patients who received RIPC had a significantly increased PaO2/FiO2 at 30 and 60 min after one-lung ventilation, 30 min after lung reexpansion, and 6 h after surgery [51].
15.4 The Future of Remote Ischemic Preconditioning for Improving Surgical Outcomes
Over 15 clinical trials were published in 2015 on the clinical use of RIPC. The ease of administration of the RIPC procedure and lack of adverse events reported in clinical trials are likely contributing factors to the continued interest in this intervention. However, RIPC is not used in routine perioperative care. The differences between study results as discussed above make it difficult to identify the patients that may benefit from the intervention. The use of biomarkers to predict RIPC response shows great promise for this purpose.
There is a need to standardize the RIPC procedure. The timing of placement of the blood pressure cuff, location of the blood pressure cuff, and duration of cuff inflation/deflation varies between studies. Also, future studies controlling for medication administration around the time of the RIPC procedure are important. Medication exposure has been discussed as a potential reason for a lack of RIPC benefit in two recently published large multicenter trials. Mehbohm et al. randomly assigned 1,403 patients undergoing cardiopulmonary bypass from 14 centers to four 5-min cycles of RIPC or sham-RIPC [38]. No differences were seen in mortality, stroke, or stage 2–3 AKI [38]. Hausenloy and colleagues using 30 centers randomized 1,612 patients undergoing cardiopulmonary bypass to RIPC or sham-RIPC as well and also found no difference in their combined primary endpoint of nonfatal myocardial infarction, death from cardiovascular causes, coronary revascularization, or stroke when evaluated 12 months after randomization [39]. Propofol was used in the perioperative period in the majority of patients in both studies [38, 39]. Propofol as well as certain inhaled anesthetics have been thought to affect the RIPC response [52–54].

Full access? Get Clinical Tree
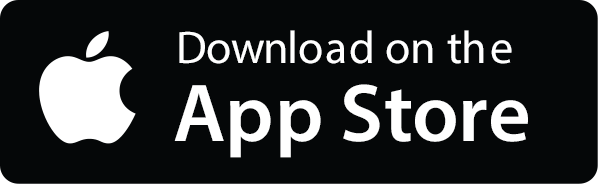
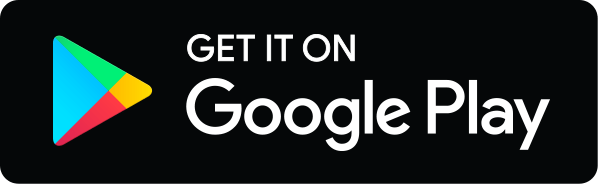