Overview of Radiation Safety
Pain practitioners have come to rely on fluoroscopy and, to a lesser but growing extent, on computed tomography (CT) to facilitate image-guided pain treatment techniques. Fluoroscopy and computed tomography employ ionizing radiation to produce the x-rays needed for imaging. Understanding the physics and biology underlying the biologic effects of ionizing radiation will help pain practitioners to minimize radiation exposure to their patients, other involved personnel, and themselves during image-guided injection. The basic elements of the fluoroscopy unit are illustrated in Figure 72.1 . X-rays emanate from an x-ray tube, typically positioned beneath the table and the patient to minimize radiation exposure. The x-rays pass through the table and the patient to strike the input phosphor of the image intensifier, where they are converted to visible light and, in turn, detected by an output phosphor that transfers the signal to a digital camera for visual display on a monitor or transfer to film. The size and shape of the x-ray beam can be adjusted after exiting the x-ray tube and before entering the patient, from side to side by an adjustable linear collimator or in a circular, concentric fashion by an iris collimator. The C-arm allows variation in the axis of the x-ray beam in numerous planes relative to the patient.

The use of CT has become more commonplace, particularly among radiologists. With the advent of fluoroscopy units that can rotate around the patient and acquire images at numerous angles and then reconstruct the images in multiple planes, the distinction between CT-fluoroscopy and traditional CT has become blurred. These CT-fluoroscopy units yield data that can be reformatted in multiple planes and produce final images rivaling the quality of conventional CT. However, acquiring images in multiple planes with either CT-fluoroscopy of conventional CT requires significantly greater radiation exposure than conventional fluoroscopy. In most instances, the superior anatomic information provided does not warrant the routine use of these advanced imaging modalities (see the further discussion of radiation dose later in this chapter). Patients undergoing high-risk procedures where small variations in anatomy can alter the risk/benefit ratio of a given technique may benefit from CT-fluoroscopy or conventional CT guidance.
Basic Radiation Physics
Radiation is energy radiated or transmitted as rays, waves, or in the form of particles. X-rays are one portion of the spectrum of electromagnetic radiation. As x-rays pass through matter, they impart enough energy to dislodge electrons (ionizing radiation), yielding free radicals that can lead to harmful biologic effects. In radiography, it is the x-rays that penetrate the body without effect that emerge to strike an image intensifier where they are converted to visible light and can be displayed on a monitor or transferred to film, producing an image based on x-ray penetrability of various tissues.
Several factors and definitions are central to any basic understanding of radiation safety. The biologic effects of ionizing radiation are proportional to the time of exposure, whereas radiation exposure is inversely proportional to the square of the distance from the radiation source. Radiation exposure is expressed as roentgen (R) or coulomb per kilogram, whereas the energy absorbed from radiation is expressed as radiation absorbed dose (rad) or as gray (Gy). Because different types of radiation can have different biologic effects, units of exposure are converted from rad to radiation equivalent in man (rem) or Sievert (Sv). The units used to express radiation exposure are listed in Table 72.1 . For x-rays, 1 R ∼ 1 rad ∼ 1 rem.
Term | Traditional Units | SI Units | Conversion |
---|---|---|---|
Exposure | Roentgen (R) | Coulomb/kg (C/kg) | 1 R = 2.5 × 10 −4 C/kg |
Radiation absorbed dose | rad | Gray (Gy) | 100 rad = 1 Gy |
Radiation equivalent in man | rem | Sievert (Sv) | 100 rem = 1 Sv |
The electrical input to the tube that generates the x-rays can be varied to produce x-rays that differ in number and energy. Increased current applied to the x-ray tube (expressed as milliamps or mA) produces more x-rays, and the more x-rays that strike the image intensifier, the darker the image. Lengthening the exposure time will also increase the number of x-rays reaching the image intensifier, thus variations in current and exposure time are expressed as mAs (mA × seconds). Increased voltage (expressed as kilovoltage peak or kVp) applied to the x-ray tube results in x-ray emission at higher energy levels (i.e., with greater ability to penetrate). In general, high kVp (75 to 125 kVp) and low mA (50 to 1,200 mA) are employed for fluoroscopy with short exposure times. This combination optimizes image quality while minimizing radiation exposure. High kVp/low mA combinations expose the patient to significantly less radiation than low kVp/high mA combinations. Modern fluoroscopy units typically employ automatic brightness control (ABC), which automatically adjusts kVp and mA to yield optimal brightness and contrast.
The x-rays generated during fluoroscopy are a form of ionizing radiation and have the potential to produce significant biologic effects. Small doses of ionizing radiation can produce molecular changes that take years to manifest in the form of cancerous transformation. Exposure to low doses of ionizing radiation is likely inconsequential because normal cellular mechanisms repair the damage. The International Committee on Radiation Safety Protection (ICRP) has produced estimates of the maximum permissible dose (MPD) of annual radiation to various organs ( Table 72.2 ). Exposure below these levels is unlikely to lead to any significant effects, but the ICRP recommends that workers should not receive more than 10% of the MPD.
Area/Organ | Annual Maximum Permissible Dose |
---|---|
Thyroid | 0.5 mSv (50 rem) |
Extremities | 0.5 mSv (50 rem) |
Lens of the eye | 0.15 mSv (15 rem) |
Gonads | 0.5 mSv (50 rem) |
Whole body | 0.05 mSv (5 rem) |
Pregnant women | 0.005 mSv to fetus (0.5 rem) |
Use of fluoroscopy for interventional procedures grew rapidly during the late 1980s, leading to increased concerns about radiation exposure. In 1994, the U.S. Food and Drug Administration (FDA) issued a public health advisory about serious radiation-related skin injuries resulting from some fluoroscopic procedures. Today’s equipment and techniques have reduced the risks of radiation exposure dramatically. Radiation exposure during a typical epidural steroid injection carried out with fluoroscopy and assuming the practitioner is at least 1 meter from the x-ray tube has been reported to be as low as 0.03 mR. In contrast, the typical entrance skin exposure during fluoroscopy ranges from 1 to 10 R per minute. A typical single chest radiograph leads to a skin entrance exposure of 15 mR. Thus, 1 minute of continuous fluoroscopy at 2 R per minute is equivalent to the exposure during 130 chest radiographs. Minimum target organ radiation doses that lead to pathologic effects are shown in Table 72.3 . Radiation dermatitis still occurs in fluoroscopists with unknown long-term consequences. Estimates of the relative radiation dose to the patient during use of fluoroscopy in comparison to other common diagnostic radiologic procedures are shown in Table 72.4 .
Organ | Dose (rad) | Dose (Gy) | Results |
---|---|---|---|
Eye lens | 200 | 2 | Cataract formation |
Skin | 500 | 5 | Erythema |
700 | 7 | Permanent alopecia | |
Whole body | 200-700 | 2-7 | Hematopoietic failure (4-6 weeks) |
700-5000 | 7-50 | Gastrointestinal failure (3-4 days) | |
5000-10,000 | 50-100 | Cerebral edema (1-2 days) |
X-ray—Chest | 0.1 mSv (10 mrem) |
X-ray—Mammography | 0.42 mSv (42 mrem) |
X-ray—Skull | 0.1 mSv (10 mrem) |
X-ray—Cervical spine | 0.2 mSv (20 mrem) |
X-ray—Lumbar spine | 6 mSv (600 mrem) |
X-ray—Upper GI | 6 mSv (600 mrem) |
X-ray—Abdomen (kidney/bladder) | 7 mSv (700 mrem) |
X-ray—Barium enema | 8 mSv (800 mrem) |
X-ray—Pelvis | 0.6 mSv (60 mrem) |
X-ray—Hip | 0.7 mSv (70 mrem) |
X-ray—Dental bitewing/image | 0.005 mSv (0.5 mrem) |
X-ray—Extremity (hand/foot) | 0.005 mSv (0.5 mrem) |
∗ Fluoroscopy, intermittent (e.g., for lumbar transforaminal or facet injection) | 0.007-0.03 mSv (0.7-3 mrem) |
∗ Fluoroscopy, high dose | (three- to sixfold the radiation exposure of standard dose) |
∗ Fluoroscopy, continuous, pulsed mode | 0.2-1 mSv/minute of exposure (20-100 mrem/minute of exposure) |
∗ Fluoroscopy, continuous | 2-10 mSv/minute of exposure (200-1000 mrem/minute of exposure) |
∗ Fluoroscopy, continuous, high dose | 10-20 mSv/minute of exposure (1000-2000 mrem/minute of exposure) |
∗ Fluoroscopy, continuous, digital subtraction | 20-40 mSv/minute of exposure (2000-4000 mrem/minute of exposure) |
Computed tomography—Head | 2 mSv (200 mrem) |
Computed tomography—Chest | 7 mSv (700 mrem) |
Computed tomography—Abdomen/pelvis | 10 mSv (1000 mrem) |
Computed tomography—Extremity | 0.1 mSv (10 mrem) |
Computed tomography—Angiography (heart) | 20 mSv (2000 mrem) |
Computed tomography—Angiography (head) | 5 mSv (500 mrem) |
Computed tomography—Spine | 10 mSv (1000 mrem) |
Computed tomography—Whole body | 10 mSv (1000 mrem) |
Computed tomography—Cardiac | 20 mSv (2000 mrem) |
∗ Fluoroscopy exposure values are approximate and vary widely based on the region of the body examined and the body habitus of each patient. The values presented are extrapolated from data provided by Philips Medical Systems for the Pulsera 9-inch mobile C-arm and the following references: Wagner AL. Selective lumbar nerve root blocks with ct fluoroscopic guidance: technique, results, procedure time, and radiation dose. AJNR Am J Neuroradiol . 2004;25:1592-1594; and Mahesh M. The AAPM/RSNA Physics Tutorial for Residents. Fluoroscopy: patient radiation exposure issues. RadioGraphics . 2001;21:1033-1045.
Basic Radiation Physics
Radiation is energy radiated or transmitted as rays, waves, or in the form of particles. X-rays are one portion of the spectrum of electromagnetic radiation. As x-rays pass through matter, they impart enough energy to dislodge electrons (ionizing radiation), yielding free radicals that can lead to harmful biologic effects. In radiography, it is the x-rays that penetrate the body without effect that emerge to strike an image intensifier where they are converted to visible light and can be displayed on a monitor or transferred to film, producing an image based on x-ray penetrability of various tissues.
Several factors and definitions are central to any basic understanding of radiation safety. The biologic effects of ionizing radiation are proportional to the time of exposure, whereas radiation exposure is inversely proportional to the square of the distance from the radiation source. Radiation exposure is expressed as roentgen (R) or coulomb per kilogram, whereas the energy absorbed from radiation is expressed as radiation absorbed dose (rad) or as gray (Gy). Because different types of radiation can have different biologic effects, units of exposure are converted from rad to radiation equivalent in man (rem) or Sievert (Sv). The units used to express radiation exposure are listed in Table 72.1 . For x-rays, 1 R ∼ 1 rad ∼ 1 rem.
Term | Traditional Units | SI Units | Conversion |
---|---|---|---|
Exposure | Roentgen (R) | Coulomb/kg (C/kg) | 1 R = 2.5 × 10 −4 C/kg |
Radiation absorbed dose | rad | Gray (Gy) | 100 rad = 1 Gy |
Radiation equivalent in man | rem | Sievert (Sv) | 100 rem = 1 Sv |
The electrical input to the tube that generates the x-rays can be varied to produce x-rays that differ in number and energy. Increased current applied to the x-ray tube (expressed as milliamps or mA) produces more x-rays, and the more x-rays that strike the image intensifier, the darker the image. Lengthening the exposure time will also increase the number of x-rays reaching the image intensifier, thus variations in current and exposure time are expressed as mAs (mA × seconds). Increased voltage (expressed as kilovoltage peak or kVp) applied to the x-ray tube results in x-ray emission at higher energy levels (i.e., with greater ability to penetrate). In general, high kVp (75 to 125 kVp) and low mA (50 to 1,200 mA) are employed for fluoroscopy with short exposure times. This combination optimizes image quality while minimizing radiation exposure. High kVp/low mA combinations expose the patient to significantly less radiation than low kVp/high mA combinations. Modern fluoroscopy units typically employ automatic brightness control (ABC), which automatically adjusts kVp and mA to yield optimal brightness and contrast.
The x-rays generated during fluoroscopy are a form of ionizing radiation and have the potential to produce significant biologic effects. Small doses of ionizing radiation can produce molecular changes that take years to manifest in the form of cancerous transformation. Exposure to low doses of ionizing radiation is likely inconsequential because normal cellular mechanisms repair the damage. The International Committee on Radiation Safety Protection (ICRP) has produced estimates of the maximum permissible dose (MPD) of annual radiation to various organs ( Table 72.2 ). Exposure below these levels is unlikely to lead to any significant effects, but the ICRP recommends that workers should not receive more than 10% of the MPD.
Area/Organ | Annual Maximum Permissible Dose |
---|---|
Thyroid | 0.5 mSv (50 rem) |
Extremities | 0.5 mSv (50 rem) |
Lens of the eye | 0.15 mSv (15 rem) |
Gonads | 0.5 mSv (50 rem) |
Whole body | 0.05 mSv (5 rem) |
Pregnant women | 0.005 mSv to fetus (0.5 rem) |
Use of fluoroscopy for interventional procedures grew rapidly during the late 1980s, leading to increased concerns about radiation exposure. In 1994, the U.S. Food and Drug Administration (FDA) issued a public health advisory about serious radiation-related skin injuries resulting from some fluoroscopic procedures. Today’s equipment and techniques have reduced the risks of radiation exposure dramatically. Radiation exposure during a typical epidural steroid injection carried out with fluoroscopy and assuming the practitioner is at least 1 meter from the x-ray tube has been reported to be as low as 0.03 mR. In contrast, the typical entrance skin exposure during fluoroscopy ranges from 1 to 10 R per minute. A typical single chest radiograph leads to a skin entrance exposure of 15 mR. Thus, 1 minute of continuous fluoroscopy at 2 R per minute is equivalent to the exposure during 130 chest radiographs. Minimum target organ radiation doses that lead to pathologic effects are shown in Table 72.3 . Radiation dermatitis still occurs in fluoroscopists with unknown long-term consequences. Estimates of the relative radiation dose to the patient during use of fluoroscopy in comparison to other common diagnostic radiologic procedures are shown in Table 72.4 .
Organ | Dose (rad) | Dose (Gy) | Results |
---|---|---|---|
Eye lens | 200 | 2 | Cataract formation |
Skin | 500 | 5 | Erythema |
700 | 7 | Permanent alopecia | |
Whole body | 200-700 | 2-7 | Hematopoietic failure (4-6 weeks) |
700-5000 | 7-50 | Gastrointestinal failure (3-4 days) | |
5000-10,000 | 50-100 | Cerebral edema (1-2 days) |
X-ray—Chest | 0.1 mSv (10 mrem) |
X-ray—Mammography | 0.42 mSv (42 mrem) |
X-ray—Skull | 0.1 mSv (10 mrem) |
X-ray—Cervical spine | 0.2 mSv (20 mrem) |
X-ray—Lumbar spine | 6 mSv (600 mrem) |
X-ray—Upper GI | 6 mSv (600 mrem) |
X-ray—Abdomen (kidney/bladder) | 7 mSv (700 mrem) |
X-ray—Barium enema | 8 mSv (800 mrem) |
X-ray—Pelvis | 0.6 mSv (60 mrem) |
X-ray—Hip | 0.7 mSv (70 mrem) |
X-ray—Dental bitewing/image | 0.005 mSv (0.5 mrem) |
X-ray—Extremity (hand/foot) | 0.005 mSv (0.5 mrem) |
∗ Fluoroscopy, intermittent (e.g., for lumbar transforaminal or facet injection) | 0.007-0.03 mSv (0.7-3 mrem) |
∗ Fluoroscopy, high dose | (three- to sixfold the radiation exposure of standard dose) |
∗ Fluoroscopy, continuous, pulsed mode | 0.2-1 mSv/minute of exposure (20-100 mrem/minute of exposure) |
∗ Fluoroscopy, continuous | 2-10 mSv/minute of exposure (200-1000 mrem/minute of exposure) |
∗ Fluoroscopy, continuous, high dose | 10-20 mSv/minute of exposure (1000-2000 mrem/minute of exposure) |
∗ Fluoroscopy, continuous, digital subtraction | 20-40 mSv/minute of exposure (2000-4000 mrem/minute of exposure) |
Computed tomography—Head | 2 mSv (200 mrem) |
Computed tomography—Chest | 7 mSv (700 mrem) |
Computed tomography—Abdomen/pelvis | 10 mSv (1000 mrem) |
Computed tomography—Extremity | 0.1 mSv (10 mrem) |
Computed tomography—Angiography (heart) | 20 mSv (2000 mrem) |
Computed tomography—Angiography (head) | 5 mSv (500 mrem) |
Computed tomography—Spine | 10 mSv (1000 mrem) |
Computed tomography—Whole body | 10 mSv (1000 mrem) |
Computed tomography—Cardiac | 20 mSv (2000 mrem) |

Full access? Get Clinical Tree
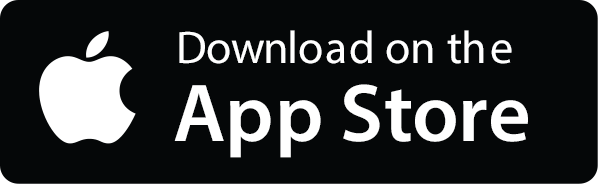
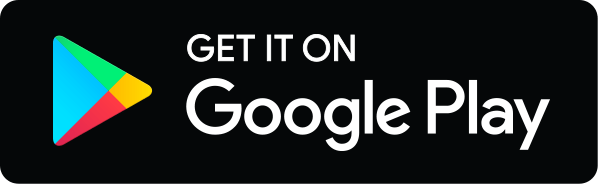
