Ionizing radiation is more dangerous than nonionizing radiation because such reactions lead to breaks in both DNA and RNA.
There are two categories of radiation injuries with which the emergency physician should be familiar:
Exposure injury, which generally represents no threat to emergency care providers.
Contamination, which may represent a risk to emergency personnel.
Acute radiation syndrome may develop following a whole-body exposure of 100 rad or more that occurs over a relatively short period of time.
Total-body irradiation with >1000 rad results in a neurovascular syndrome.
In the presence of contamination, if the patient’s condition permits, decontamination should begin in the prehospital setting.
Radiation accidents involving discarded medical and industrial sources get little attention compared to problems at nuclear power plants or weapon facilities. Unfortunately, these accidents occur around the world with surprising regularity, and in some instances prove deadly.
According to the Radiation Emergency Assistance Center/Training Site (REAC/TS) Radiation Accident Registry, between 1944 and March 2017, there have been 462 accidents worldwide. These accidents resulted in significant radiation exposure to 2985 individuals and in 129 deaths (Table 143-1).
Despite the relatively rare incidence of medically significant pediatric radiation accidents, our dependence on nuclear energy makes it necessary for today’s emergency physician to understand its potential for disaster. Most obvious are the threats of sophisticated nuclear weaponry to individuals of all ages. However, the near disaster of Three Mile Island in Pennsylvania in 1979, the Chernobyl Nuclear Power Station catastrophe in the former Soviet Union in 1986, and the Fukushima Dai-ichi Nuclear Disaster as a result of the March 2011 tsunami in Japan serve as potent reminders of the severe underlying hazards to children and adults, even in peaceful nuclear energy utilization. A more probable predicament, however, is an isolated or limited exposure in a medical, industrial, or research accident, or during the transport of radionucleotides. Basic preparation for radiation emergencies is not difficult, but a thorough understanding of the pathophysiology and clinical presentation is necessary in order to successfully handle all aspects of these complex problems and minimize the risk to providers.
All individuals are susceptible to radiation injury if the exposure is of significant dose and duration. Children, however, may suffer greater short-, and long-term consequences of a significant radiation exposure for several reasons. Children are more susceptible to relatively greater internal exposure to inhaled radioactive gases given their disproportionately higher minute ventilation.1 They are also at greater risk for developing subsequent malignancies when they are exposed to radiation.2,3 In the event of nuclear fallout, the short stature of children increases their exposure to a higher concentration of radioactive material on the ground.1 In addition, children are more likely than adults to experience psychological trauma leading to enduring psychological injury after a radiation disaster.1,4
Radiation is a general term used to describe energy that is emitted from a source and results in the transfer of energy through space. The term encompasses the broad-wavelength microwave and extends through the ultrahigh-frequency γ-rays. A radioactive substance, referred to as a radioisotope or radionucleotide, gives off radiation. A person exposed to external or remote radiation has been irradiated but does not become radioactive. The victim may give off radiation only if there was external or internal contamination caused by the presence of radioactive particles (α and β).
Radiation can be classified as either ionizing or nonionizing. In addition, radiation can be described either as nonparticulate (electromagnetic) or particulate. Electromagnetic radiation has no mass and no charge. It occurs in waveforms and is described by wavelengths. Examples of this nonparticulate/electromagnetic radiation can be found in both the ionizing and nonionizing radiation classifications. In contrast, particulate radiation has mass and can either be charged or uncharged ionizing radiation.
Nonionizing radiation is relatively low energy in nature and does NOT result in acute radiation injuries or contamination. The adverse effects to humans are limited to local heat production. A classic example of the effects of nonionizing radiation in humans is sunburn. In order of decreasing energy content, the nonionizing forms of radiation include ultraviolet rays, visible light, infrared radiation, microwaves, and radio waves. These types of radiation are easily shielded by protective barriers (e.g., sunscreen, clothing, etc.) and do not result in contamination or penetration of human tissue. These forms of nonionizing radiation also represent many of the electromagnetic radiations. Their energy content is less than that of ionizing radiation, making them a less threatening form of radiation (Table 143-2).
Nonionizing Nonparticulate and electromagnetica Ultraviolet rays Visible rays Infrared rays Microwaves Radio waves Ionizing Nonparticulate and electromagnetic No mass No charge Penetrating Examplesa γ-Rays X-Rays Particulate Has mass Uncharged and penetrating Neutrons More damaging than x-rays or γ-rays No electrical charge Charged and nonpenetrating α-Rays 2 Protons 2 Neutrons 2+ Charge β-Rays 1 Electron 1 Charge |
Ionizing radiation is named for its ability to interact with matter. Types of ionizing radiation include α-particles, β-particles, and neutrons, which represent particulate radiation (has mass); and x-rays and γ-rays, which are nonparticulate forms of radiation (has no mass).
Ionizing radiation is more dangerous than nonionizing radiation because such reactions lead to breaks in DNA, RNA, and proteins, damaging important biologic functions at the cellular metabolic level. Anomalies may be passed on to subsequent offspring or they may result in cell death or the inability to replicate. Ionized radiation has a high frequency, a short wavelength, and a billion times more energy than nonionizing radiation. Common sources of ionizing radiation are nuclear reactors, nuclear weapons, radioactive material, and radiography equipment. Material identified by the label radioactive produces ionizing radiation.
γ-Rays have the highest energy content of the nonparticulate and massless radiations. Their photon radiation originates in the atomic nucleus. They can penetrate deep into the tissue, depositing energy and interacting with the various layers they penetrate. Constant low-level exposure through cosmic radiation is a usual source. γ-Radiation is a common cause of acute radiation syndrome due to radioisotope decay and radiation from linear accelerators. A lead shield, 1 to 2 cm in depth or thick concrete, would provide satisfactory protection from γ-rays.
X-rays have the next highest energy content of the nonparticulate, massless radiations. Unlike the γ-ray, which is produced within the nucleus of the atom, x-rays originate from outside the nucleus and are emitted by excited electrons. Like the γ-ray, x-rays can also penetrate tissue and deposit energy deep within the cells, are shielded by lead, and can be detected with a Geiger counter. Their usual source is medical or industrial in nature.
α-Particles are composed of two protons and two neutrons and possess a 2+ electrical charge. They originate from the nucleus of the atom, and being relatively heavy radioactive emissions, can travel only inches from their source. In general, they cannot penetrate paper or epidermis because of their mass and size and are rarely harmful externally. Examples include plutonium, uranium, and radium. α-Particles can emit significant amounts of radiation and cannot be detected with standard Geiger counters.
β-Particles have a small mass, composed of a single electron emitted from the atom’s nucleus, and possess a 1 charge. They can disperse only a few feet from their source and penetrate tissues only a small amount (up to 8 mm), primarily causing thermal injuries. Children have less keratinized epithelium and are at greater risk than adults. Clothing alone can often provide adequate protection from β-particles. Despite their inability to penetrate the skin to any significant depth, both α- and β-particles can be harmful if they are ingested or inhaled or if wounds are contaminated by these particles. The common research isotope tritium is an example, as is carbon14 and phosphorous.
Neutrons are the third type of particulate radiation. Without an electrical charge, they ionize by colliding with atomic nuclei within cells and tissues. They possess strong power to penetrate, and represent the only form of radiation that can make previously stable atoms within the body radioactive. They can be more damaging than x-rays or γ-rays and are responsible for radioactive fallout. Emitted only after nuclear detonation, nuclear reactors, weapons, and accelerators are common sources of neutron radiation. Specialized concrete is necessary to provide shielding from neutron radiation.
Although radiation cannot be sensed by the human body, it can be detected and quantified by dosimeters or Geiger–Mueller tubes at levels far below those that result in any biologic significance. There are several units of measurement used in relation to radiation: roentgen is the unit of measurement used during the production of x-rays that measure the ion pairs produced in a given volume of air; dose represents the amount of energy deposited by radiation per unit of mass; and the rad (roentgen absorbed dose) is the basic unit of measurement. A rad can be defined as a unit of absorbed dose of radiant energy that is equal to 100 erg of energy deposited per gram of absorbing material. The gray (Gy) represents the standard international (SI) unit for dose:
Units of rem (roentgen equivalent in man) represent a calculated radiation unit of dose equivalent. The absorbed dose (rad) is multiplied by a factor to account for the relative biologic effectiveness (RBE) of the various types of radiation:
β-particles, x-rays, and γ-rays have an RBE of 1 and for these sources of radiation 1 rad = 1 rem. The RBE for α-particles and neutrons is 20, and therefore 1 rad = 20 rem for these sources of radiation.
The sievert (Sv) is the SI unit for dose equivalent, where
Therefore, 1 Gy = 100 rad = 100 rem = 1 Sv. Generally, the terms rem and mrem (millirem) are used when they refer to the exposure of biologic systems.
The clinical impact of radiation exposure depends on several factors. These factors are also important to properly coordinate the safety of both prehospital and hospital providers who may respond to a radiation incident.
The clinical effects of radiation exposure are related to the type of radiation involved, the amount of radiation, and the nature of the exposure (continuous or intermittent). In addition, the harmful effects of ionizing radiation may be affected by the total time of the exposure, the distance from the radiation source, and the presence of any shielding (amount and type).
A radiation exposure over a prolonged period of time is less likely to be harmful to an individual than the same dose over a shorter time period. For example, an exposure of 100 rem (1 Sv) in 1 second will be more harmful than an exposure of 100 rem over 1 year.
An inverse square relationship exists between distance from a radiation source and the resultant exposure, making increased distance an effective means to reduce the amount of exposure. An exposure can be reduced by a factor of four simply by doubling the distance from the radiation source. Tripling the distance will decrease the exposure by a factor of nine.
Shielding may be an effective method to reduce radiation exposure when one is dealing with low-energy radiation (x-rays). When dealing with medium- or high-energy radiation, shielding may become impractical due to the amount of lead or concrete that would be necessary.
In the United States, there are natural and technological radiation sources to which children and adults are commonly exposed (Fig. 143-1). Americans were exposed to more than two times as much ionizing radiation in 2006 compared to the early 1980s.5 Most categories had not changed significantly, but diagnostic medical procedures had increased more than seven times.5 With an annual projected exposure of 1,870,000 Sv for each individual, background exposure was estimated to account for 50% of the total, and medical exposure 48%. The remaining 2% was from consumer products as well as industrial, security, educational, research, and occupational exposures.5
Technological sources of radiation may represent a wide range of exposures to individuals. Color television may result in an exposure of 1 mrem/y; a round-trip, coast-to-coast jet flight may result in a 2- to 5-mrem exposure; and a chest x-ray causes an exposure between 5 and 10 mrem. The common radiation exposure to a patient during angiography may be 1000 mrem. As technology changes and new technologies are developed, we are likely to encounter additional sources of radiation in varying amounts.
There are two categories of radiation injuries with which the emergency physician should be familiar. The first type is an exposure injury, which generally represents no threat to emergency care providers. Contamination, the second type of radiation injury, may represent a potential risk to emergency personnel.
Exposure radiation injuries can be classified into two categories. A person may be the victim of a localized radiation injury or may have suffered a whole-body exposure.
A large dose of radiation exposure to a small part of the body will result in a local (partial-body) radiation injury. These injuries often occur over months or even years, but they may occur over a shorter amount of time.6
Localized radiation injuries most commonly affect the hand/upper extremities, with the buttocks and thighs representing the next most common sites. Adults and children may unknowingly come into contact with a radiation source by handling an unknown object and putting it into their pockets. Typically, these injuries occur in the occupational setting. Localized radiation accidents may also result from an inadvertent exposure to an intense radiation beam.
The dose of radiation that can result in a local radiation injury varies greatly. Larger doses are often better tolerated than a whole-body exposure. Accidental exposures from radioactive sources with a surface dose of nearly 20,000 rad/min have been reported to have caused localized radiation injuries.
The initial clinical picture of a localized radiation injury depicts a thermal injury to the skin. While thermal burns develop soon after an exposure, erythema from a local radiation injury is delayed.7 Radiation injury should be considered in the differential diagnosis for any patient who presents with a painless “burn” but who does not remember a thermal or chemical insult.
Prolonged radiation exposure causes blood vessel fibrosis, leading to tissue necrosis. The outcome will be determined by the degree of blood vessel and tissue damage. Classification of these localized injuries can be divided into four types, differentiated by increasing epidermal and dermal injury. They are summarized in Table 143-3.
Type | Presentation | Exposure | Comments |
---|---|---|---|
Type I | Erythema only | 600–1000 rad | Similar to a first-degree thermal burn. Erythema may be delayed up to 2–3 wk. A dose of 300 rad can result in a delayed hair loss (epilation). Dry desquamation or scaling may occur. |
Type II | Transepidermal injury or wet desquamation | 1000–2000 rad | Similar in severity to a second-degree partial-thickness thermal burn. |
Type III | Dermal radionecrosis | >2000 rad | Severe pain with or without paresthesia. Resembles a severe chemical or scalding burn. Skin grafting may be necessary. |
Type IV | Chronic radiation dermatitis | Recurrent exposure over several years | Can result in an eczematous appearance of the skin. Ulcerations and carcinoma are not uncommon. |
Pediatric gonads and eyes are very sensitive to radiation exposure. Depression of spermatogenesis and destruction of oocytes may occur. In addition, low doses of radiation may induce premature cataract formation.8
Acute radiation syndrome may develop following a whole-body exposure of 100 rad or more that occurs over a relatively short period of time. Even at doses that do not cause immediate injury, children are at an increased risk for leukemia, thyroid cancer, ocular cataracts, growth retardation, and infertility. This is due to the fact that sensitivity to radiation varies directly with the rate of cell production and the number of future cell divisions. Children have increased cell propagation, greater surface-area-to-mass ratio, and smaller reserve than adults. Organ systems with rapidly dividing cells (bone marrow and gastrointestinal tract) are the most vulnerable to radiation injury. With greater doses of radiation, however, all organ systems may become involved, including the central nervous system.9

Full access? Get Clinical Tree
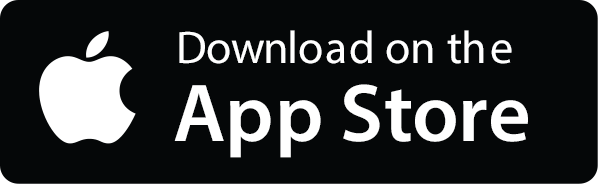
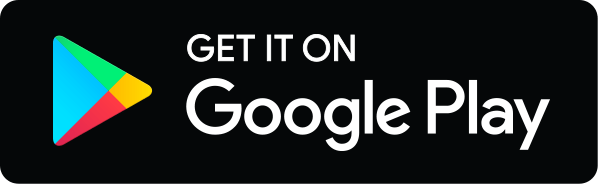