Pulmonary Toxins
BACKGROUND
The pulmonary system has multiple important physiologic functions. The most essential—oxygenation of hemoglobin—occurs across a layer of endothelial cells in the pulmonary alveoli. Toxins that displace oxygen are termed simple asphyxiants. This group of gases includes hydrocarbons, noble gases, nitrogen, and carbon dioxide. Inhalation of simple asphyxiants reduces the fraction of inspired oxygen (FiO2) below 21% and lowers the partial pressure of oxygen. These gases leave the pulmonary parenchyma largely intact allowing normal gas exchange to resume once the patient is removed from the toxic environment.
Inhaled toxins that injure or inflame the pulmonary alveoli and result in lung injury are termed pulmonary irritants. These gases disrupt the alveolar epithelial integrity by different mechanisms. Some gases such as chlorine (Cl2) and ammonia (NH3) form acids or bases that cause inflammation and disruption of surfactant. Other gases such as ozone (O3) and oxides of nitrogen (NOx) produce reactive free radicals or intermediates that result in inflammatory injury, sometimes hours after exposure. These gases are a major contributor to air pollution and high rates of asthma and symptomatic airway disease.
SIMPLE ASPHYXIANTS
Sources of simple asphyxiants include workplace releases of noble gases and agricultural sources such as methane. Iatrogenic asphyxiation may occur in hospitals when nitrous oxide is affixed to a patient’s face for prolonged periods, or when a nitrogen line is inadvertently confused for supplemental oxygen. Some simple asphyxiant sources exist in a nongaseous phase (e.g., dry ice [CO2] in a solid form, or nitrogen [N2] in liquid form).1 Asphyxiation from these sources usually occurs in a confined space, requiring phase transition to a gas.2–4 Occasionally, simple asphyxiants are associated with mass casualties: the large-scale emission of carbon dioxide gas from Lake Nyos, a carbonated volcanic lake in Cameroon, West Africa, asphyxiated more than 1,700 residents and thousands of livestock within a radius of 10 km.5,6
Management Guidelines
Because simple asphyxiants reduce the effective fraction of inspired oxygen (FiO2), the signs and symptoms of toxicity are similar to those of hypoxia; they include altered sensorium, syncope, coma, seizures, and cardiac arrest. Depending on the severity of the global tissue hypoxia, end-organ injury may develop, leading to significant morbidity and mortality. Simple asphyxiants typically do not interfere with ventilation, and with the exception of asphyxia by carbon dioxide, hypercarbia is absent until respiratory depression or arrest occurs. The primary therapeutic intervention in simple asphyxiant toxicity is patient removal from the exposure and restoration of adequate oxygenation and ventilation. In the setting of multiorgan failure from prolonged tissue hypoxia, therapy is limited to supportive care. Given that simple asphyxiants do not cause primary lung injury, recovery is complete provided end-organ damage from tissue hypoxia has not occurred.
PULMONARY IRRITANT GASES
Inhaled pulmonary irritants can destroy the alveolar epithelium, resulting in impaired alveolar gas exchange. The initial disruption of respiratory tract integrity is attributed to one or both of two causes: the acid or base produced when pulmonary irritant gases dissolve in the physiologic alveolar fluid and the generation of free radicals, specifically reactive oxygen species.7–9 Although the exact mechanism of injury is unknown, the toxic end products of pulmonary irritants are believed to cause direct cellular damage and initiate an inflammatory cascade that can lead to the development of acute respiratory distress syndrome (ARDS). Highly water-soluble toxic gasses such as concentrated ammonia quickly irritate mucous membranes, often prompting rapid retreat from the irritant source. Less water-soluble agents, such as chlorine gas, phosgene, and oxides of nitrogen, do not cause as rapid a reaction and are more likely to result in prolonged exposures.
Two well-known pulmonary irritant gases are chlorine gas [Cl2] and phosgene [COCl2]. During World War I, both Axis and Allied forces used these agents as chemical weapons; today, they are used in the production of pharmaceuticals, plastics, textiles, and pesticides.8,10 A majority of exposures to these pulmonary irritants result from mass casualty occupational, industrial, or transportation accidents.8–14 Domestic exposures—most notably to chlorine gas—do occur occasionally and typically result from unsafe mixing of cleaning agents or from inappropriate use and storage of swimming pool chlorinating solutions (see below).8,12,15,16
Chlorine [Cl2]
While the majority of chlorine gas exposures result from occupational- and industrial-related incidents, nonindustrial exposure can occur when acidic cleaning agents such as hydrochloric acid [HCl] are mixed with sodium hypochlorite [NaOCl], or ble- ach.12,15,17,18 Chlorine is a yellowish green gas that has a distinct and readily recognized odor with a density that is twice as heavy as air. It tends to settle near the ground and is dispersed by air movements. The mechanism of injury is the formation of hydrochloric acid, hypochlorous acid, and nascent oxygen [O−] (oxygen liberated from a chemical reaction) when the chlorine gas dissolves in the alveolar fluid.8 Complicating this process is nascent oxygen’s ability to induce the formation of free radicals, which can cause additional pulmonary injury.
The severity of the toxic effects of chlorine gas inhalation varies from minor respiratory tract irritation to death, depending on ambient concentration of the gas and the duration of exposure.19 From available data, exposure to 1 to 15 parts per million (ppm) will produce mild to moderate mucous membrane and conjunctival irritation. Inhalation of >30 ppm of chlorine gas results in chest pain, cough, and shortness of breath; chemical pneumonitis and acute pulmonary edema are observed at concentrations between 40 and 60 ppm. Exposure to concentrations above 400 ppm results in death, usually over a period of 30 minutes, while exposure to concentrations >1,000 ppm may be fatal within minutes.7,8
The intermediate water solubility of chlorine gas often results in mild or delayed initial symptoms, including conjunctival and nasal irritation, that can contribute to an unintentionally prolonged duration of exposure.8 A majority of exposures, however, remain brief, with inhalation of low to moderate concentrations resulting in transient symptoms such as cough, shortness of breath, and wheeze.12,17,19–21 Prolonged exposure can result in severe pulmonary sequelae—often not observed until 4 to 8 hours following inhalation—including pneumonitis, pulmonary edema, and ARDS.7,9,21
Chloramines [NH2Cl, NHCl2, and NCl3]
Chloramine is produced when chlorine interacts with nitrogen-containing compounds.22–24 This typically occurs during chlorination of indoor swimming pools where chlorine, in the form of bleach, is used to disinfect both organic (urea and creatinine) and inorganic (ammonia) nitrogen-containing compounds.23–25 In households, chloramine exposures are reported after two common household cleaning products, bleach and ammonia, are mixed.23–28 Three different types of chloramines can be generated, depending on the degree of chlorination: monochloramine [NH2Cl], dichloramine [NHCl2], and trichloramine [NCl3].23 The dissolution of chloramine in physiologic fluid (e.g., in the alveoli epithelial layer or mucous membrane) generates hypochlorous acid [HOCl], ammonia, and oxygen radicals—toxic end products that cause irritation of the eyes, respiratory tract, and mucosal membrane. Because chloramines are highly water-soluble, symptom onset is typically immediate, prompting the exposed person to escape to fresh air and minimize prolonged exposure. Significant morbidity, including pneumonitis, has been reported when exposure occurs in a confined space with limited ventilation.26–28
Phosgene [COCl2]
Similar to chlorine gas, phosgene has become an important compound in the chemical industry after its debut during World War I. Phosgene is used in the production of organic solvents, dyes, pesticides, and pharmaceuticals.10,29 The majority of phosgene exposures also occur in the industrial setting.10,30,31 Phosgene gas may also be emitted by combustion of chlorinated hydrocarbons such as plastic or polyvinyl chloride (e.g., house or vehicle fire).30
Contact with phosgene causes tissue injury by two distinct chemical mechanisms: acylation and hydrolysis.29,32 Acylation (the process of adding an acyl group to a compound) is believed to be the primary mechanism, occurs when phosgene reacts with nucleophilic components of macromolecules (amino, hydroxyl, thiol, and sulfhydryl groups), and produces injury by permanent denaturation of proteins and lipoproteins.29,32 The second mechanism is the hydrolysis of phosgene in the physiologic fluid of the alveoli, which results in the production of hydrochloric acid [HCl] and carbon dioxide [CO2] responsible for the initial mucous membrane irritation.32,33
The characteristic odor of phosgene gas is described as “fresh hay” (odor threshold 0.4 to 1.5 ppm).29 This property may contribute to prolonged exposure by inciting deep breathing rather than alarm at the presence of a toxic gas. Moreover, due to phosgene’s intermediate water solubility, early symptoms of mucosal irritation are frequently minor, further prolonging exposure and allowing deeper penetration into the lower respiratory tract.34
Phosgene toxicity produces three clinical phases: reflex, latent, and delayed pulmonary edema.35,36 During the initial reflex phase, symptom severity is related to gas concentration.29,32 Phosgene concentrations of >3 to 5 ppm result in immediate mucous membrane irritation, cough, and chest tightness.10,29,31,32 Initial exposure stimulates a vagal reflex, which results in decreased vital capacity from rapid shallow breathing, bradycardia, and hypotension.32,33 In the subsequent latent phase, patients may be asymptomatic for up to 48 hours; in the final, delayed phase, they may develop noncardiogenic pulmonary edema and respiratory distress.10,29–31,35,37 While the severity of the initial mucosal irritation is dependent on gas concentration, the delayed development of pulmonary edema is correlated with the total dose exposure and reflects a dose–response relationship.29,32,38,39 Clinically latent pulmonary interstitial inflammation occurs at exposures to 30 to 150 ppm/min, while overt pulmonary edema occurs at exposures to >150 ppm/min. The estimated lethal dose for mortality of 1%, 50%, and 100% is 300 ppm/min, 500 ppm/min, and 1,300 ppm/min, respectively.32,33
Management Guidelines
The principle management strategy for pulmonary irritant gas exposure is to remove the patient from the exposure and provide supportive care, including ensuring adequate oxygenation and ventilation. Following removal, patients should be undressed and examined for other potential toxic exposures and traumatic injuries. There is no antidote for pulmonary irritant exposure and no indication for decontamination in setting of acute chlorine, chloramine, and phosgene gas exposure. Decontamination protocols should be considered if dermal or ocular exposure to liquid agents has occurred. Irrigation of the eye and other mucosal surfaces may help reduce irritation, and if respiratory tract irritation is evident, supplemental oxygen and bronchodilators (β-agonists) should be administered.
Several studies have investigated the role of inhaled nebulized sodium bicarbonate in neutralizing the acidic end products of pulmonary irritants and in reducing pulmonary injury.40–44 In general, chemical neutralization is contraindicated, especially in ingestion of acid or alkali agents, due to its potential to exacerbate the initial caustic injury by producing an exothermic reaction and gas formation. Some practitioners have attempted chemical neutralization of pulmonary irritants, considered safe because the large surface area of the lung and the low concentration of pulmonary irritant end products would allow for the dissipation of the heat and gas produced. To date, however, no convincing evidence exists to support the routine use of inhaled nebulized sodium bicarbonate in pulmonary irritant exposures.40–44 One case report (n = 3) showed immediate relief of symptoms (cough and dyspnea) after 3.75% NaHCO3 nebulized solution was administered following chlorine exposure.40 However, a small prospective trial (n = 22) of 4.2% NaHCO3 nebulized solution for patients with chlorine and chloramine exposures failed to demonstrate any clinical benefit.42 Chemical neutralization using NaHCO3 has not been studied in gas exposures other than chlorine. Since definitive evidence is lacking, a brief trial of nebulized sodium bicarbonate <4.2% may be attempted in awake patients if tolerated, and continued only if subjective relief occurs, but is unlikely to affect outcome.
The most serious complication of pulmonary irritant exposure is the development of ARDS. In chlorine and phosgene exposure, physical exam findings (rales or crackles) and radiologic signs of pneumonitis or pulmonary edema may not be evident for up to 8 and 48 hours, respectively.7,9,21,30,31,33,35,45 Although the etiology of ARDS caused by pulmonary irritants is different from the etiology of injury caused by sepsis or trauma, the similarities of the underlying inflammatory response suggest that similar management principles apply. Specifically, targeting tidal volumes of 6 mL/kg and plateau pressure of <30 cm H2O decreases inflammatory markers and improves survival.46,47
In animal model studies, corticosteroids have been shown to improve oxygen delivery and lung compliance and possibly decrease pulmonary injury risk in chlorine gas-induced ARDS and pneumonitis.48–52 To date, no trials have investigated the role of corticosteroids in countering the inflammatory process during human pulmonary irritant exposure.
Patients exposed to chloramine and chlorine gas who remain asymptomatic after 8 hours of observation may be safely be discharged with adequate follow-up in place. Asymptomatic patients exposed to phosgene should be observed for 24 hours to monitor for delayed onset of pulmonary edema and pneumonitis.
Other Pulmonary Toxins
Although beyond the scope of this chapter, other chemicals, including salicylate, opioids, cocaine, carbon monoxide, and negative inotropic agents (beta-blockers and calcium channel blockers), have also been implicated in pulmonary toxicity/ARDS.6,53–58 Treatment of lung injury from these agents generally requires supportive care and, in some cases, such as salicylates, treatment to enhance elimination of toxin.
CONCLUSION
Exposure to simple asphyxiants and pulmonary irritants is potentially fatal. Patients exposed to simple asphyxiants who survive to reach medical care generally require only short-term supportive care. Patients surviving pulmonary irritant exposure may present with early and/or late mucosal and respiratory symptoms; for these patients, the specific irritant, duration of exposure, and severity of symptoms will determine the extent of supportive care needed. Although pulmonary toxins are less commonly treated in the ED than many other emergencies, a familiarity with the information presented in this chapter is indispensable for the emergency physician committed to managing the full spectrum of critically ill patients.
LITERATURE TABLE

Full access? Get Clinical Tree
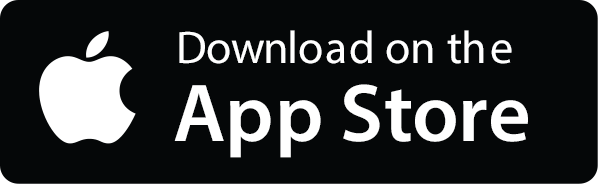
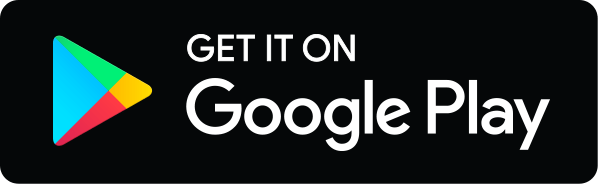