Pulmonary System
A-A Gradient
The A-a gradient indicates whether gas transfer is normal and gives an idea of how well oxygen is moving from the alveoli to the arterial blood. It helps to distinguish hypoventilation from other causes such as ![]() ![]() |

The more exact formula, using the alveolar gas equation, is the following:
An easier and less complicated method to predict shunting is the PaO2/FiO2 (P/F) ratio. It is calculated thus:

Using the criteria from the previous example:
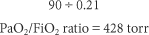
Normal is 286 torr; lower indicates a shunt.
Abg Interpretation
(see also Base Excess/Deficit p. 174; ABG’s in Part 9, p. 340)
ABG Interpretation
pH 7.35–7.45 | PaCO2 35–45 | HCO3 22–26 | |
---|---|---|---|
Respiratory acidosis | ↓ | ↑ | — |
Respiratory alkalosis | ↑ | ↓ | — |
Metabolic acidosis | ↓ | — | ↓ |
Metabolic alkalosis | ↑ | — | ↑ |
Uncompensated | Abnormal | Either abnormal | |
No indication that the opposite system has tried to correct for the other | |||
Partially compensated | Abnormal | Abnormal | Abnormal |
One system has attempted to correct for the other but has not had success | |||
Fully compensated | Normal | Abnormal | Abnormal |
Normal pH indicates that one system has been able to compensate for the other |
Steps to ABG Interpretation
1. Check the pH | >7.45 = Alkalosis <7.35 = Acidosis Normal = no imbalance or compensated |
2. Check the PaCO2 | >45 = CO2 retention related to
<35 = CO2 blown off related to:
Normal = No imbalance of respiratory component |
3. Check the HCO3 | >26 = Nonvolatile acid lost (HCO3 gained). Indicates
<22 = Nonvolatile acid is added (HCO3 lost). Indicates
Normal = No imbalance of metabolic component |
4. Determine the imbalance | |
5. Determine if compensation exists |
Remember:
pH, PaCO2 opposite direction = respiratory problem
pH, HCO–3 same direction = metabolic problem (“M” for “married”)
HCO–3, PaCO2 same direction = compensation for abnormal pH
HCO–3, PaCO2 opposite direction = two mixed imbalances present
Respiratory Acidosis (↓pH, ↑PaCO2)
Respiratory acidosis is related to stroke, drug overdose, aspiration, pneumonia, acute respiratory distress syndrome (ARDS), cardiac arrest, chronic obstructive pulmonary disease (COPD), hypoventilation, and neuromuscular disorders.
Treatment: Aggressive chest PT, suction. Increase respiratory rate, increase tidal volume.
Respiratory Alkalosis (↑pH, ↓PaCO2)
Respiratory alkalosis is related to anxiety, fear, head trauma, brain tumor, hepatic insufficiency, fever, mechanical overventilation, pulmonary embolism, and thyrotoxicosis.
Treatment: Sedation, support, breathe in paper bag for attack of hyperventilation. Decrease respiratory rate, decrease tidal volume.
Metabolic Acidosis (↓pH, ↑HCO–3)
Metabolic acidosis is related to renal failure, diarrhea, TPN, acetazolamide (Diamox) (diuretic that prevents carbonic acid formation), ketoacidosis, and lactic acidosis (caused by bicarbonate loss or excess acids in extracellular fluid).
Treatment: Treat underlying cause, monitor intake/output and dysrhythmias, protect against infection.
Metabolic Alkalosis (↑pH, ↑HCO-3)
Metabolic alkalosis is related to volume depletion (loss of H+, Cl–, K+ from vomiting or diarrhea, gastric suction, or diuretic therapy).
Remember:
al-K+–low-sis means potassium value is low when patient is alkalotic.
Treatment: Treat underlying cause, monitor intake/output, potassium replacement therapy.
Abgs, Mixed Venous
(see Mixed Venous ABGs, p. 191)
Acute Respiratory Failure
Acute respiratory failure is the inability of the respiratory system for adequate gas exchange, namely, sufficient oxygenation, carbon dioxide elimination, or both. Thus, it can be classified as either hypoxemic or hypercapnic.
In practice, it is defined by exhibiting any two of the following four:
Tachypnea (>35 breaths/min)
PaO2 <50 mm Hg on room air or <70 mm Hg on 50% O2 mask
PaCO2 >50 mm Hg
pH <7.3 (showing significant respiratory acidosis)
Additional criteria include:
Altered mental status
Vital capacity <15 mL/kg
Inspiratory force <25 cm H2O
PaO2/FiO2 ratio <250
Albuterol
(see Respiratory Inhalation Medications, p. 202)
Alupent
(see Respiratory Inhalation Medications, p. 203)
Aminophylline
(see Part 8, p. 301)
Anion Gap
(see Part 5, p. 250)
Ards
(see also Prone Positioning, p. 198)
Acute respiratory distress syndrome (ARDS) (formerly known as adult respiratory distress syndrome until 1994, when it was recognized that the malady is not confined to adults) is a form of severe respiratory failure associated with pulmonary infiltrates originating from several insults involving damage to the alveolocapillary membrane with subsequent fluid accumulation within the air spaces of the lungs. In effect, ARDS is pulmonary edema without volume overload and without depressed left ventricular function.
Cause is either a direct or an indirect injury to the lungs:
Sepsis and systemic inflammatory response (SIRS)—most common reason
Multiple fractures or long bone fractures (trauma)—related to fat embolism
Severe head injury—discharge of sympathetic nervous system, resulting in acute pulmonary hypertension and injury to the capillary bed
Pulmonary contusion—due to direct trauma to the lung
Multiple transfusions—potential increases with the number of units transfused
Near drowning, aspiration, and pneumonia—direct insult to lung tissue
Smoke inhalation—damage from particulate matter inhaled into the lower lung. (These patients should be monitored closely, even if symptoms of ARDS are initially absent.)
Drug overdoses—tricyclic antidepressants are the most common. (This risk is independent of aspiration.)
Hallmarks of ARDS:
Bilateral infiltrates on chest x-ray film
PAWP <18 mm Hg with no evidence of left atrial hypertension
Impaired oxygenation (regardless of PEEP) with a PaO2/FiO2 ratio of <200 torr
The signs and symptoms are usually subtle:
Chest x-ray film appearance may be normal early in the disease but will digress to show bilateral pulmonary infiltrates (a “ground-glass” appearance) and ultimately a complete whiteout of both lungs.
ABGs initially show hypoxia with respiratory alkalosis early in the disease and respiratory acidosis as the disease progresses.
High peak inspiratory pressures
Increase in the A-a gradient
Decreased compliance
Decreased FRC
P/F ratio <200 torr
The treatment is mainly supportive because there are no specific measures to correct increased vascular permeability or correct the inflammatory process. Mechanical ventilation is generally employed to keep O2 saturation above 90%. PEEP may be added in small increments to reverse compression atelectasis. Routine or prophylactic use of antibiotics or corticosteroids is not beneficial; in fact, administration of multiple antibiotics may lead to the development of multiple drug-resistant infections. Despite years of research, mortality remains as high as 40% to 60%.
Arterial Blood Gases
(see ABG Interpretation, p. 168)
Arterial O2 Content
Arterial oxygen content (CaO2) is primarily determined by the amount of hemoglobin in arterial blood that is saturated with oxygen:

Remember:
A quicker way to estimate this value: Hgb × 1.39 × SaO2
Normal value: 15 to 24 volume percentage
Assist/Control Ventilation
(see Ventilator Modes, p. 212)
Asthma
Asthma is from the Greek for “to pant.” Hallmarks are tenacious sputum and reversible airway obstruction. Patient is dehydrated (related to tachypnea) and has decreased
vesicular breath sounds with hyperexpansion of chest and decreased chest wall excursion. There is severe airway obstruction and respiratory acidosis. (In less severe attacks, there may be a decrease in the PCO2 related to hyperventilation and respiratory alkalosis.)
vesicular breath sounds with hyperexpansion of chest and decreased chest wall excursion. There is severe airway obstruction and respiratory acidosis. (In less severe attacks, there may be a decrease in the PCO2 related to hyperventilation and respiratory alkalosis.)
Status asthmaticus is an acute exacerbation of asthma refractory to initial treatment with bronchodilators and is a medical emergency. It can lead to respiratory failure, unconsciousness, and cardiopulmonary arrest. Advanced symptoms include extreme dyspnea, coughing, and wheezing. There are little or no breath sounds, inability to speak, cyanosis, and diaphoresis.
Treatment of choice: Bronchodilators (inhaled or nebulizers), oral or IV steroids, and hydration. Oxygenation with mechanical ventilation may need to be considered. Aminophylline is rarely used, but it may be considered if other therapy fails or if the patient had been using it previously.
Atelectasis
Atelectasis refers to the absence of gas in a lung segment or an entire lobe, resulting in lung collapse. Since the right middle lobe is the most narrow and is surrounded by lymphoid tissue, it is the most common area to be atelectatic. While almost always a secondary phenomenon, atelectasis has a direct morbidity linked to it because of hypoxemia. There are four primary causes:
Airways have intrinsic obstruction.
Airways have extrinsic obstruction (compressed).
Lung tissue is compressed.
Alveoli incompletely expand and eventually collapse.
Absorption atelectasis is a hazard of high FiO2 and will most likely occur when there is an obstruction (e.g., a mucous plug) causing blockage of the airways leading to the alveoli. Nitrogen makes up about 80% of the air we breathe but is not absorbed at all by the lung under normal atmospheric conditions. Therefore, nitrogen remains in the lung even after the oxygen has been absorbed, to help keep the alveoli from collapsing. However, if a high concentration of FiO2 is delivered, the nitrogen is “washed out” (and replaced by the delivered gas.) When this gas is absorbed completely, the alveoli collapse, and atelectasis results.
Atropine
(see Respiratory Inhalation Medications, p. 202)
Autopeep
Normally, end-expiration is a “no-flow” state, and the pressure in the alveoli equals the pressure at the mouth. In conditions of autoPEEP (intrinsic PEEP), however, the pleural and alveolar pressures remain positive at end-expiration, compared with mouth pressures, related to air “trapped” in the alveoli. Subsequently, when the next breath comes, airway pressure increases, and the breaths are “stacked.” (This is frequently seen when the respiratory rate and/or minute volume are high, but it can also develop because of a narrow ET tube, water clogging in the exhalation part of the circuit, or a stiff exhalation valve.) AutoPEEP adds to the work of breathing because the patient must generate a negative pleural pressure to initiate inspiration.
How do you know if autoPEEP is present? You can tell this easily in a mechanically ventilated patient by looking at the expiratory airflow. Using the ventilator graphics, if
expiratory flow continues until the onset of the next inspiratory cycle, a pressure gradient must exist during the flow, and, by definition, autoPEEP exists.
expiratory flow continues until the onset of the next inspiratory cycle, a pressure gradient must exist during the flow, and, by definition, autoPEEP exists.
Management of the condition is done by adding extrinsic PEEP, thus removing the additional work of breathing required to overcome the positive pressure gradient from the alveolus to the mouth. Expiratory time also needs to be increased. This can be accomplished by decreasing the respiratory rate, setting the I:E ratio in favor of expiration, or increasing peak flow, which will decrease inspiratory time.
Base Excess/Deficit
The base excess/deficit is a calculated number that represents the amount of base that must be added to restore the blood to a normal pH of 7.4 (Normal range = -2 to +2 mEq/L).
Base excess is a positive number and signifies metabolic alkalosis.
Base deficit is a negative number and signifies metabolic acidosis.
Remember:
Base deficit (acidosis) ← pH 7.4 → Base excess (alkalosis)
The parameter is confusing, in that the terms excess and deficit are sometimes used interchangeably. To be correct, excess should be reported as a positive number, and deficit should be reported as a negative number.
Bipap Ventilator Support
Bilevel positive airway pressure (BiPAP) is a noninvasive, positive pressure ventilation system, usually delivered via face mask (thus eliminating the need for intubation or tracheostomy) and is used to augment breathing for patients who feel that continuous positive airway pressure (CPAP) is unbearable because it is too difficult to exhale against the back pressure. The patient breathes spontaneously, and the circuit is maintained at one pressure during exhalation and a higher pressure during inhalation. It is helpful for patients who have difficulty breathing (often at night) related to COPD, congestive heart failure (CHF), cystic fibrosis, sleep apnea syndrome, and neuromuscular diseases resulting in respiratory insufficiency. It does not provide total ventilation requirements and should never be used as a life-support device.
Bleb
(see also Bulla, p. 176)
A bleb is a small bulla, usually found in the subpleural zone of the lung abutting the pleura.
Boyle’s Law
When the pressure of a gas is doubled and the temperature remains constant, the volume of the gas is reduced by one half. At a constant temperature (T), the volume of a gas (V) is inversely proportional to the pressure (P) of the volume. That pressure remains constant if the temperature is unchanged.

Example: Think of an inflatable pool raft. When you get on it to relax, initially it will compress under your weight. But at a certain point, the compression ceases and the pressure inside the raft is maintained to keep you afloat.
Breath Sounds, Normal
Bronchial breath sounds are normal over the upper third of the sternum and trachea. If they are heard anywhere else, they are abnormal. The sound is produced by the air movement through the larynx, setting up vibrations, not by the air leaving the alveoli. The sound is loud and high pitched.
Bronchovesicular breath sounds are heard over the two lower portions of the sternum, just to either side. They are heard posteriorly between the scapulae. If they are heard anywhere else, the sound is due to partial atelectasis or pulmonary consolidation. The sound is soft with a lower pitch.
Vesicular breath sounds (inspiration) are produced by air entering the alveoli directly, separating the alveoli. They can be heard anywhere anteriorly. Posteriorly, they can be heard anywhere except between the scapulae. The sound is soft and breezy, with the lowest pitch.
Breath Sounds, Abnormal
Rales (crackles) can usually be heard only on inspiration and are found wherever the underlying pathologic condition is. The sound is similar to rubbing strands of hair together, next to the ear. They correlate with early left-sided failure, and the degree to which they are audible corresponds to the degree of the failure. They may also be caused by interstitial lung disease and pneumonias. If the rales disappear with three or four breaths or with a cough, they are not due to fluid but to the actual separating of the alveolar walls. They are then known as dry rales, atelectatic rales, or hypostatic rales. Rales are common after the patient has been at bed rest for 24 hours.
Rhonchi are heard predominantly on expiration and are related to secretions in larger airways. The sound is of a continuous snoring quality, similar to rubbing two inflated balloons together.
Wheezes can be heard on inspiration or expiration. When breathing is partially constricted (as in asthma), the sound can be heard primarily on expiration. It has a musical quality, or is likened to creaking or groaning.
A rub is caused by parietal and visceral pleura rubbing caused by inflammation. The sound stops if the patient holds the breath. A rub is usually heard on inspiration and expiration and is a superficial squeaking or grating sound, similar to rubbing two pieces of leather together.
Breathing Patterns
(see Respiratory Patterns, p. 203)
Brethine
(see Respiratory Inhalation Medications, p. 202)
Bronchitis
The patient with bronchitis has recurrent, excessive bronchial mucous secretion, resulting in a productive cough. The increased secretions often render the patient a perfect host for bacteria. The description “blue bloater” is often given because of the presence of hypoxemia resulting in cyanosis and the tendency toward edema. Treatment is aimed at improving alveolar ventilation and relieving the hypoxemia through vigorous pulmonary toilet, postural drainage, intermittent positive-pressure breathing (IPPB) therapy, bronchodilators, and mucolytic agents.
Bronkosol
(see Respiratory Inhalation Medications, p. 202)
Bulla
A bulla is a thin-walled, localized pocket of air in lung tissue. Bullae vary in size from being barely visible on x-ray film to several centimeters in diameter. They may occur in otherwise normal lungs or may be a result of primary emphysema. Secondary infections often develop.
Capnography
Measured by monitoring samples of expired gas from a circuit attached to an endotracheal tube, CO2 measured at end-exhalation (PETCO2) can be used to approximate the level of alveolar CO2, because the percentage of arterial carbon dioxide dissolved in the blood (PaCO2) closely approximates the percentage of alveolar CO2. As a rule of thumb, PETCO2 values are usually just slightly lower than PaCO2 levels. In fact, the two values tend to move in opposite directions about 22% of the time. A normal capnogram will show a slow rise in CO2, with a plateau at end-expiration and a steep drop at the beginning of inspiration.
![]() Figure. Characteristics of a normal capnogram. (Courtesy of Agilent Technologies and Tom Ahrens, St. Louis, MO.) |
Measuring the PETCO2 enables many types of pulmonary assessments to be made:
Monitoring patients during weaning from the ventilator. Because PETCO2 provides continuous estimates of alveolar ventilation, monitoring the values is useful in determining readiness for extubation.
Predicting survival during cardiopulmonary resuscitation. PETCO2 is a noninvasive indicator of cardiac output, with both values moving in the same direction. A PETCO2 <10 mm Hg after 20 minutes of resuscitation is generally considered a reliable indicator that resuscitation will be unsuccessful.
Detecting incorrect placement of endotracheal tubes.
When proper tracheal placement occurs, the capnogram will show a waveform. If inadvertent esophageal placement occurs, the waveform will be flat because no CO2 is eliminated from the esophagus.
An alternative device to confirm correct ETT placement is a CO2 detector. These devices respond quickly to exhaled CO2 with a simple color change (device specific) from purple/blue to yellow. REMEMBER: Yellow is mellow!
Disconnection of the patient from the ventilator. Because capnography directly monitors the patient and not the equipment, disconnection from the ventilator will show an immediate flat line on the capnogram.
Identifying patient’s attempts to breathe while receiving paralytic agents. There should be no movement of the diaphragm during paralysis; therefore, incomplete paralysis can be detected by a dip in the capnogram waveform, indicating an attempt at a spontaneous breath.
Detecting a potential diagnosis of pulmonary embolism. When there is pulmonary dead space, the relationship between the PaCO2 and the PETCO2 is lost. Thus, in a condition such as a pulmonary embolism, the exhaled air from a poorly perfused part of the lung contains only a small amount of CO2 and results in a reduced PETCO2.
Two Patients with Shortness of Breath and a Potential Diagnosis of Pulmonary Emboli (PE)
Patient 1 | Patient 2 | |
---|---|---|
PaCO2 | 36 mm Hg | 39 mm Hg |
PETCO2 | 32 mm Hg | 21 mm Hg |
Interpretation | No significant PE Normal PaCO2–PETCO2 gradient | PE should be considered Widened PaCO2–PETCO2 gradient |
CO2 detection during nasal cannula oxygen delivery is possible via flow through mainstream or sidestream capnometry, in nonintubated patients. Normal EtCO2 values are 35 to 45 mm Hg, with some experts stating that as low as 30 mm Hg can be normal. Accurate measurements are often difficult in patients who are mouth breathers or who have obstructed nares, and a special sampling cannula should be utilized in this situation.
Carbon Monoxide Poisoning
(see also Hyperbaric Oxygen, p. 186; Oxyhemoglobin Dissociation Curve, p. 194)
Carbon monoxide, with affinity for hemoglobin 210 times greater than oxygen, combines with hemoglobin (Hgb) to form carboxyhemoglobin (COHb). Carbon monoxide impairs oxygen unloading at the tissue level, and the oxyhemoglobin dissociation
curve shifts to the left. This results in decreased O2 available to tissues, creating cerebral hypoxia. The patient has a characteristic cherry-red skin color and flu-like symptoms, such as headache, nausea, vomiting, fatigue, and weakness. Treatment is with 100% oxygen until all signs and symptoms resolve. Hyperbaric oxygen (HBO) therapy can be used to decrease the half-life, but because of the poor availability of HBO chambers, its use is limited.
curve shifts to the left. This results in decreased O2 available to tissues, creating cerebral hypoxia. The patient has a characteristic cherry-red skin color and flu-like symptoms, such as headache, nausea, vomiting, fatigue, and weakness. Treatment is with 100% oxygen until all signs and symptoms resolve. Hyperbaric oxygen (HBO) therapy can be used to decrease the half-life, but because of the poor availability of HBO chambers, its use is limited.
Carboxyhemoglobin Levels
Carboxyhemoglobin Level | Symptoms |
---|---|
0–5% | Normal |
<15% | Often in smokers, truck drivers |
15–40% | Headache, some degree of confusion |
40–60% | Loss of consciousness, Cheyne-Stokes respirations |
50–70% | Mortality >50% |
Charles’s Law
Known as the law of volumes, Charles’s law states that at a constant pressure, the volume of a given mass of an ideal gas will expand as the temperature rises, and likewise, the volume of the gas will contract as the temperature decreases.

Example: Think of a warm can of soda. When opened, there is usually enough pressure built up within the container to cause the contents to spew all over. (Some containers will spontaneously explode if exposed to heat or direct sunlight.) Conversely, if the container were cold, very little pressure would be released.
Chest Tubes
(see also Effusion, p. 182; Heimlich Valve, p. 184; Pneumothorax, p. 197)
Chest tubes are used in patients who require removal of air, blood, or fluid from the pleural or mediastinal space. Tube sizes are in French (F). The smaller ones (16F–20F) are used for removing air, whereas the larger ones (22F–40F) are used for removing blood and thick drainage. The proximal end of the chest tube has eyelets to drain the air or fluid, and the distal end connects to the drainage unit. There are five basic components of the system:
Collection chamber
Capacity 2,000 to 2,500 cc depending on model used. More than 200 mL/hr drainage usually signals a bleeding complication. Drainage fluid will overflow from one compartment to the next. When reading, note that there may be a decrease in original volume of the first compartment after fluids spill over into the next, attributed to surface tension “buildup.” The actual volume of the previous compartment should be checked for accuracy if the total output is critical.
Suction chamber
Suction is applied to the chest tube unit as ordered, usually -20 cm of water. The suction source needs to be set at typically 80 mm Hg to achieve -20 cm of water suction. If multiple chest tubes are pulling from one suction canister, increased amounts
of wall suction may be required to achieve the ordered suction amount on all chest tube units.
Dry suction system set-up
This set up does NOT require water to be added to establish suction. Suction is achieved by dialing a suction control knob to the desired amount and applying wall suction as described above. Various chest tube suction indicators, such as floats, bellows, or displays are available on differing models, but they will all “activate” when suction is applied, confirming that the system is operational.
Wet suction system set-up
Older systems DO require the addition of water to the suction chamber to establish the prescribed suction level. Increments on the suction chamber indicate the water fill level for different amounts of suction. Apply wall suction and when gentle bubbling is noted in the suction chamber, the correct amount of suction has been applied. Excessive suction/bubbling will not achieve greater amounts of suction and will evaporate the water more quickly, requiring replacement of water more often to maintain the ordered suction level. On some systems, like the dry suction, various indicators such as floats will confirm activation of suction.
Water seal chamber or one-way valve
Chest tubes of varying models will require either water to be added to establish a water seal, or have a one-way valve that allows air to leave the pleural cavity, but preventing it from returning and thereby maintaining a negative pressure system. If the model requires water to be added, it must be filled to a minimum of the 2-cm mark.
Air leak meter
Some drainage units provide an air leak meter (not to be confused with a water seal) that permits air leak detection. The air leak chamber must be filled with water, usually 30 mL, to the level indicated on the unit. The meter is labeled from low (1) to high (7) on most models. The higher the numbered column through which the bubbling occurs, the greater the degree of air leak. The column beside the air leak meter usually fluctuates with breathing, which represents normal tidaling. Negative pressure can build up in this column, which may be reflected by a rising fluid level. Continuous bubbling may indicate a leak in the system (check connections), whereas intermittent bubbling that fluctuates with respirations is mostly likely caused by the lung.
High negativity relief valve
This valve is provided to vent excessive negativity. If pressed, filtered air will enter the unit. Use caution! If suction is not operative (gravity drainage), depressing this valve can reduce the negative pressure within the collection chamber to zero (atmosphere) and result in a possible pneumothorax. The need for use of this valve is rare. Stripping or milking thoracic chest tubes can cause excessive negativity, and using this valve will restore prescribed levels. Some units feature an indicator window with a picture of a palm tree or the word “yes” when proper negativity exists. This icon should appear continuously while the patient is to suction but may appear intermittently during gravity drainage.
Troubleshooting
Specimen collection
Using a standard luer lock syringe, specimens should be taken from the needleless self-sealing sampling port found in the connector on the chest tube. The connector and the syringe can be inverted to facilitate sample collection. (Important: DO NOT take the
specimen from the tubing itself, as tubing is NOT self-sealing, and a needle puncture could cause an air leak.)
Accidental removal of chest tube, from insert site
Cover the site with a dry, sterile dressing. If you hear air leaking from the site, tape the dressing on only three sides to allow the escape of air and to prevent a tension pneumothorax. Call the physician, and prepare for a new chest tube insertion.
Inadvertent disconnection from drainage unit
Submerge the distal end of the chest tube in about 1 inch of sterile 0.9% NaCl to create a liquid seal until a new drainage unit can be prepped and attached. DO NOT CLAMP.
Drainage unit is full and needs to be changed
After prepping a new drainage unit, remove the current one from suction. Clamp the chest tube with a rubber-tipped hemostat, or the clamp provided on the tubing, and disconnect from the old unit. (Caution: Be sure that suction is OFF before you clamp the tube! Clamping while suction is still on could cause a pneumothorax.) Quickly connect to the new unit, unclamp the tube, and resume suction.
Unit has tipped; drainage spilled into other compartments of collection chamber
There are two alternatives. One is to change the drainage unit to a new one, another is to mark each chamber appropriately and add all the amounts together to determine total drainage.
Chest X-Ray Film
(see Part 10, p. 360)
Chronic Obstructive Pulmonary Disease
Chronic obstructive pulmonary disease (COPD) is a group of respiratory diseases that obstruct the pathway of normal alveolar ventilation, by either spasm of the airways, mucous secretions, or morphopathologic changes of airways and/or alveoli. The three most common are (1) chronic bronchitis, (2) pulmonary emphysema, and (3) bronchial asthma.
Signs typically include elevated pulmonary artery pressures, peaked P on electrocardiograph (EKG) tracing (pulmonary hypertension causes right atrial enlargement and position change), incomplete right bundle branch block, increased functional residual capacity (and barrel chest), multifocal atrial tachycardia (due to hypoxia and right ventricular strain), and compensatory polycythemia (increase in red blood cells [RBCs] to increase the hematocrit).
Treatment is to first treat the hypoxia with vigorous pulmonary toilet and postural toilet, then give IPPB therapy (to aerate alveoli and remove CO2), bronchodilator therapy, mucolytic agents, antibiotics (usually ampicillin), or steroids. The Venturi mask delivers most controlled O2 for patient.
Closed Pneumothorax
(see Pneumothorax, p. 197)
Co2 Monitoring
(see Capnography, p. 176)
Combitube
The combitube is a unique device used to facilitate emergency or difficult intubation of a patient. It does not require visualization of the larynx and is a double lumen tube, with
one blind end. Once placed, inflation of the cuff allows the device to function as an endotracheal tube, and closes off the esophagus allowing for ventilation and preventing reflux of gastric contents.
one blind end. Once placed, inflation of the cuff allows the device to function as an endotracheal tube, and closes off the esophagus allowing for ventilation and preventing reflux of gastric contents.
Compliance
Static compliance is a measurement of the elastic properties of the lung, usually measured while patient is using the ventilator. Reduction in compliance also tends to reduce patient’s tidal volume and increase breathing frequency to overcome the work of moving large volumes of air. To calculate:

Dynamic compliance is a measurement to evaluate the work of breathing or the amount of force needed to overcome airway resistance. To calculate:

Studies have shown that as ratios of dead space to tidal volume are increased, compliance falls, which indicates that the patient’s status is deteriorating.
Controlled Mandatory Ventilation
(see Ventilator Modes, p. 212)
Copd
(see Chronic Obstructive Pulmonary Disease, p. 180)
Cpap
(see Ventilator Modes, p. 212; BiPAP Ventilator Support, p. 174)
Cuff Measurement
(see Endotracheal Tube Placement, p. 183)
Dalton’s Law
Known as the law of partial pressures, Dalton’s law states that the total pressure of a mixture of gasses is equal to the sum of the partial pressures of each individual component of the gas mixture.
Example: Think of a bag filled with an equal amount of marbles and popcorn. You squeeze the bag tightly, over and over again. Eventually, the popcorn will compress more than the marbles, and the proportion of marbles to popcorn kernels will change. Dalton’s law says this will NOT happen with gases. Mixed gases will always stay in the same proportion under pressure, and none will compress any more than others.

Dead Space
Dead space is a measurement to determine total wasted ventilation, or that which is ineffective in gas exchange. It is normally one third of tidal volume, but this may increase markedly in the late phase of ARDS or in pulmonary embolism.
Deep Vein Thrombosis
(see Pulmonary Embolism, p. 200)
Dynamic Compliance
(see Compliance, p. 181)
Ebus (Endobronchial Ultrasound)
(see Part 10, p. 363)
Effusion
(see also Pneumothorax, p. 197; Thoracentesis in Part 4, p. 241)
Also known as hydrothorax, effusion is clinically the same as pneumothorax, but an effusion has fluid infiltrates instead of air. An empyema has pus-like fluid. On x-ray film, a pleural effusion shows blunting of the costophrenic angle, silhouetting of the diaphragm, and meniscus sign (a crescent-shaped inclusion of air surrounded by consolidated lung tissue).
Embolism
(see Pulmonary Embolism, p. 200)
Emphysema
(see also Chronic Obstructive Pulmonary Disease, p. 180)
From the Greek for “inflation,” emphysema is an obstructive respiratory disorder characterized by air trapped in overdistended alveoli and by collapse of bronchioles on expiration, causing prolonged expiratory outflow. The lungs are hyperresonant on chest percussion. Even if disease is severe, patient maintains a normal gas exchange and often has an elevated hematocrit; thus, the term “pink puffer” is often applied.
Empyema
(see Effusion, p. 182)
End Tidal Co2 Monitoring
(see Capnography, p. 176)
Endobronchial Ultrasound
(see Part 10, p. 363)
Endotracheal Tube Placement
Nasotracheal tube size: Usual 7.5 internal diameter (ID)
Endotracheal tube size: Adult female: Usual 7.0 to 8.0 ID (internal diameter)
Adult male: Usual 7.5 to 8.5 ID (internal diameter)

Full access? Get Clinical Tree
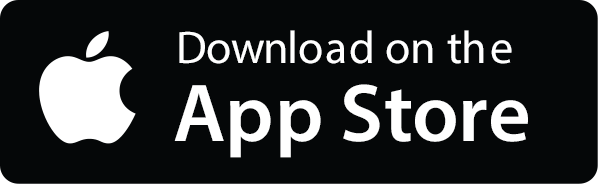
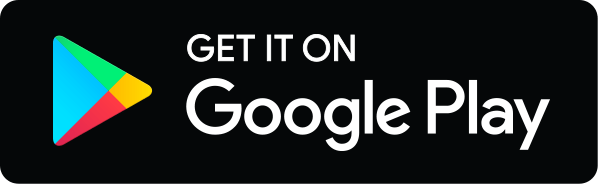