Chapter 42 Principles of Meteorology and Weather Prediction
General Circulation and Atmospheric Profile
Climate Controls and Radiation Balance
The three-division structure results in surface wind distribution characterized by winds blowing from the east (easterly) (trade winds) out of the subtropical high-pressure zone to the low-pressure zone at the equator; winds from the west (westerly) out of the subtropical highs to the midlatitude low-pressure zone; and polar easterlies flowing from the polar high-pressure zone to the midlatitude low (Figure 42-1). Such an east–west wind direction, as opposed to a north–south wind direction, prevails because of the Coriolis effect, which acts on the pressure gradient force and deflects winds to the right in the northern hemisphere and to the left in the southern hemisphere.
Climatic Regions Controlled by Latitude: Tropics, Midlatitudes, and Poles
Midlatitude and Polar Climates
Cold and warm fronts are typically oriented around a cyclone as shown in Figure 42-2. The colder, typically polar air mass advances equatorward behind the cyclone at the cold front, while the warmer air mass advances poleward ahead of it behind the warm front. Typically, the faster-moving cold front catches up to the warm front as the cyclone matures and “occludes,” and the cyclone begins to lose its strength as the temperature differences from which it derives its energy dissipate. Occasionally neither air mass will be able to dislodge the other, and the front will become stationary. Stationary fronts can be conducive to prolonged periods of precipitation.
tropical cyclones
These are further categorized using the Saffir-Simpson scale, ranging from category 1 to category 5 (most severe) (Table 42-1).
Mountain Climates
The lifting of air because of the presence of mountains is known as orographic lift, which results in cooling of the air. If sufficient moisture is present, condensation takes place. Such convection can induce thunderstorm development on the windward side of the mountain (Figure 42-3).
Marine/Coastal Climates
The difference in heat capacity between land and ocean initiates a diurnal sea–land breeze circulation. During the day, land heats up much faster than does the ocean, establishing a land–ocean temperature difference that causes a pressure gradient. Wind blows toward the lower pressure over land to equalize the pressure difference. At night, land surfaces cool rapidly compared with adjacent oceans. The cycle is reversed at night, with winds blowing from the land toward the ocean to dispel the ocean–land temperature (and hence pressure) gradient (Figure 42-4).

Full access? Get Clinical Tree
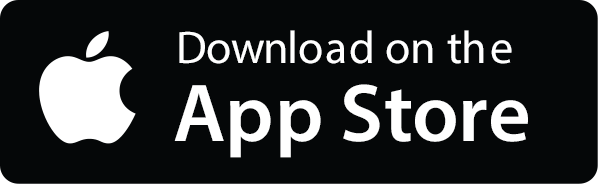
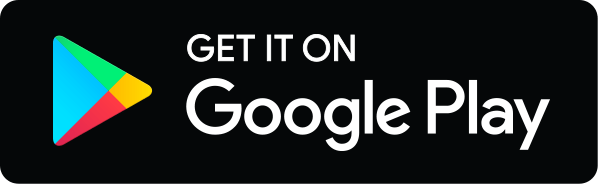