Key Points
- ▪
The anesthesia preoperative evaluation, which is the clinical foundation for guiding perioperative patient management, reduces perioperative morbidity and enhances patient outcome.
- ▪
The fundamental purpose of preoperative evaluation is to obtain pertinent information regarding the patient’s medical history, formulate an assessment of the patient’s perioperative risk, and develop a plan for any requisite clinical optimization.
- ▪
The preanesthesia evaluation should include a focused clinical examination, documentation of comorbid illness, reduction of patients’ anxiety through education, assurance that preexisting medical conditions are optimally managed, selective referrals to medical specialists, ordering of preoperative investigations, initiation of interventions intended to decrease risk, discussion of aspects of perioperative care, arrangements for appropriate postoperative care, and recommendations to delay or cancel the surgical procedure, if deemed appropriate.
- ▪
Comorbid medical diseases can influence anesthesia and perioperative management, thus requiring the anesthesiologist to be knowledgeable in many aspects of internal medicine.
- ▪
Patients require appropriate preoperative diagnostic and laboratory studies consistent with their medical history and the proposed surgical procedure. Routine preoperative testing cannot be justified because it is costly and often clinically inappropriate.
- ▪
The anesthesiologist-led preoperative evaluation outpatient clinic can enhance operating room efficiency, decrease day-of-surgery cancellations or delays, reduce hospital costs, and enhance the quality of patient care.
- ▪
New and updated preoperative evaluation consensus and evidence-based guidelines published by multiple medical specialties have important influences on the preparation of patients for anesthesia and surgery.
- ▪
Anesthesiologists must be aware of, and be compliant with, increasing regulatory and reporting requirements involving preoperative issues by healthcare agencies.
- ▪
The anesthesiologist is the perioperative medical specialist and thus is uniquely positioned to evaluate the risks associated with anesthesia or surgery, discuss these risks with the patient, and manage them perioperatively in collaboration with the surgical team, referring physician, and other medical specialists.
Acknowledgment
The editors, publisher, and Dr. Duminda Wijeysundera would like to thank Dr. Bobbie-Jean Sweitzer for her contribution to this chapter in the prior edition of this work. It has served as the foundation for the current chapter.
Preoperative evaluation is required prior to the administration of any anesthetic. Its practice and scope have changed dramatically. Initial changes were driven by a rapid transformation in practice from hospital admission of patients the night before surgical procedures to admission on the morning of surgery. More recently, preanesthesia evaluation has become integral to the Perioperative Surgical Home model that aims to develop an integrated model for managing the entire perioperative episode of care. This evolution in practice has led to a new approach to preoperative evaluation, which is a vital role of the perioperative physician. Accordingly, many anesthesiologists have expanded their responsibilities from providers of intraoperative anesthesia care to perioperative medical specialists who use their unique knowledge and experience to manage medical complexities related to surgery. This expanded role entails that anesthesiologists assume the leadership role in the assessment and optimization of patients who are scheduled for surgery. This chapter provides a comprehensive discussion of the practice of preoperative evaluation, as well as a review of related concepts, current evidence, and consensus-based clinical practice guidelines.
Evolution of Preanesthesia Evaluation
All patients requiring anesthesia for surgery must undergo a preoperative evaluation by their anesthesia provider. The conduct of this evaluation has changed substantially. Historically, anesthesiologists assessed their patients for the first time either just before the surgical procedure or on the preceding day, with the remainder of preoperative evaluation and preparation being the responsibility of the surgeon, primary care provider, or other specialist. In some countries, this approach remains the usual clinical practice. Nonetheless, in many countries, anesthesiologists have increasingly taken on a leadership role in preoperative evaluation and preparation, well in advance of the scheduled procedure. This is particularly true for high-risk patients or those undergoing high-risk procedures.
Several factors explain this change. First, considerably fewer patients are admitted to the hospital prior to the day of surgery. In many countries, there is little financial justification for the previous model of admitting patients prior to the day of surgery. For example, most surgical procedures in the United States are performed on an outpatient or same-day admission basis, including major neurosurgical, cardiac, and cancer resection procedures. Second, surgical patients are increasingly likely to be older frail individuals with significant burdens of medical comorbidity. For such patients, sufficient time is needed between the preanesthesia evaluation and the planned surgery to facilitate testing, interventions, and medical optimization. In the case of patients with very high-risk medical comorbidity, preoperative consultation by an anesthesiologist can help inform a shared decision-making process for proceeding with surgery (see section on “Frailty, Geriatric Conditions, and the Older Surgical Patient”). Third, anesthesia care increasingly extends beyond the operating room alone. Preoperative evaluation is an integral component of the anesthesiologists’ role as perioperative physicians who are involved in integrated medical care before, during, and after surgery. The specialty is uniquely positioned for this role given its expertise in the management of medical complexities related to anesthesia and surgery. This view of preoperative evaluation as a key component of the integrated management of the entire perioperative episode of care is essential to the Perioperative Surgical Home model.
Outpatient preoperative assessment clinics have been instrumental in facilitating greater involvement of anesthesiologists in preoperative evaluation. These clinics also impose new clinical and organizational challenges. In an institution where most surgical patients are evaluated in the preoperative assessment clinic, anesthesiologists may have less time to evaluate medically complex patients. Consequently, anesthesiologists must achieve a high level of efficiency and accuracy in the assessment of a patient’s history, physical examination, and differential diagnosis, as well as in the planning of perioperative management. Conversely, in a hospital where only the highest-risk patients are referred for consultation at a preoperative assessment clinic, the anesthesia department must interact with surgical departments to establish general protocols that ensure capture of the information needed to perform anesthesia safely, as well as the appropriate selection of individuals who require preoperative anesthesia consultation. In addition to changes in the scope and timing of the preanesthesia evaluation, this assessment has increasingly been influenced and governed by practice guidelines. For example, in the United States, The Joint Commission mandates documentation of a history and physical examination for any surgical patient within 30 days before the planned procedure, as well as reassessment within the 48-hour period immediately preceding the surgical procedure. The American Society of Anesthesiologists (ASA) and European Society of Anaesthesiology (ESA) have published specific guidelines for preoperative anesthesia evaluation. In addition, several other specialty societies published practice guidelines specifically relating to the preoperative management of medical issues in surgical patients.
Goals and Benefits of Preanesthesia Evaluation
Preoperative evaluations help influence and improve perioperative care ( Fig. 31.1 ). Indeed, inadequate preoperative evaluation was a contributing factor in 3% of perioperative adverse events in the Australian Incident Monitoring Study database. The overarching goals of preanesthesia evaluation are to (1) ensure that the patient can safely tolerate anesthesia for the planned surgery; and (2) mitigate perioperative risks such as pulmonary or cardiovascular complications. In pursuit of these goals, preanesthesia evaluations offer opportunities to perform focused clinical examinations, better document comorbid illness, reduce the patient’s (and family’s) anxiety through education, optimize preexisting medical conditions, make selective referrals to medical specialists (e.g., cardiologists), order specialized investigations (e.g., cardiac stress tests), initiate interventions intended to decrease risk, discuss aspects of perioperative care (e.g., anticipated risks, fasting guidelines), and arrange appropriate levels of postoperative care (e.g., critical care unit). If a patient is deemed to be at very high risk for adverse perioperative outcomes, the anesthesiologist may also recommend consideration of a nonoperative or less invasive treatment. Such a recommendation helps inform shared decision making for surgery (see Clinical Examination During Preoperative Evaluation section). In some cases, preanesthesia evaluation can identify a previously unrecognized medical condition (e.g., hypertension) that may not significantly affect perioperative risks, but warrants longer-term follow-up by appropriate healthcare professionals.

Compared to preoperative evaluations performed by surgeons or primary care physicians alone, anesthesiologist-led preoperative evaluation is associated with more selective ordering of laboratory tests and referral to specialists, thereby leading to reduced healthcare costs. Within the context of an anesthesiologist-led preoperative evaluation clinic, preanesthesia evaluations also associated with reduced anxiety, improved acceptance of regional anesthesia, fewer day-of-surgery case cancellations, shorter hospital length-of-stay, and lower hospital costs.
Clinical Examination During Preoperative Evaluation
The clinical examination , consisting of the history and physical examination, is a fundamental component of preoperative evaluation by anesthesiologists. This information helps identify the underlying basis for the planned surgery, clarify the extent of comorbidities with specific perioperative relevance, identify opportunities for preoperative optimization, and select any appropriate preoperative testing. The consistency and quality of preoperative examinations are enhanced through standardization. Specifically, the baseline clinical examination of all surgical patients should include a consistent set of components, with opportunities for more detailed examination of one or more of these components (e.g., cardiovascular system) based on the findings from the standardized initial examination. Computer-based preoperative evaluation tools offer a mechanism for improving the standardization of high-quality preoperative assessments, and are recommended in the 2018 ESA guidelines on preoperative evaluation. The quality of the preoperative physical examination is further enhanced by appropriately considering evidence-based implications of different findings.
Components of The Medical History
The important components of the anesthesia history are presented in the sample preoperative history form in Fig. 31.2 . This information can be documented, either on paper or an electronic form, by anesthesia staff during an in-person or telephone interview with the patient. Alternatively, the patient can complete a form capturing the required information, either in person or remotely through a web-based program. The preanesthesia evaluation starts with the planned surgery and its indication. The development of the underlying condition necessitating surgery (e.g., cancer) and any prior related therapies need to be evident. Current known medical problems, past medical issues, previous surgeries, anesthesia types, and anesthesia-related complications must be noted. A simple notation of diseases or symptoms such as hypertension, diabetes mellitus, ischemic heart disease, shortness of breath, or chest pain is not sufficient. Rather, their associated severity, stability, associated activity limitations, exacerbations (current or recent), prior treatments, and planned interventions should be clearly documented. All related diagnostic test results, interventions, and names of treating physicians should be reviewed. The patient’s responses to these initial questions may elicit further inquiry to establish a complete history.


Prescription and over-the-counter medications (including supplements and herbal medications) should be documented, along with their dosages and schedules. This information should include any recently interrupted medications with perioperative implications (e.g., recent corticosteroid therapy). The anesthesiologist should list any allergies to medications and other substances (e.g., latex, radiographic dye), with special emphasis on the patient’s response to the exposure. Patients often claim an “allergy” to a substance when, in reality, the reaction was an expected side effect (e.g., nausea or vomiting with narcotics). Tobacco, alcohol, or illicit drug use must be documented. Tobacco exposure is best quantified using pack-years (number of packs of cigarettes smoked per day, multiplied by the number of years of smoking); as an example, an individual who smoked two packs of cigarettes daily for the prior 10 years is deemed to have a 20 pack-year history of tobacco use. A personal or family history of pseudocholinesterase deficiency and malignant hyperthermia (including a suggestive history such as hyperthermia or rigidity during anesthesia) must be clearly documented to facilitate appropriate planning before the day of surgery. Information from previous anesthetic records may clarify an uncertain history.
A standardized general review of all organ systems should then be performed. For example, patients should be asked whether they ever had problems with their heart, lungs, kidneys, liver, and nervous system. In addition, they should be asked about any history of cancer, anemia, bleeding problems, or prior hospitalization for any reason. The principal emphasis should be on airway abnormalities, anesthesia-related adverse events (personal or family history), as well as symptoms of cardiovascular, pulmonary, hepatic, renal, endocrine, or neurologic disease. For example, a report of a patient who has experienced excessive sore throat, dental injuries, or “the need to have a small tube” with previous anesthetic cases may be an indication of previous difficulty with airway management. A history of snoring and daytime somnolence may suggest undiagnosed sleep apnea (see later section on “Obstructive Sleep Apnea”). Any history of chest discomfort (including its duration, precipitating factors, associated symptoms, and relieving factors) could be important. Similarly, a list of previous surgical procedures should be obtained and can help complete the medical history. Finally, records from primary care physicians, specialists, or inpatient admissions may reveal any issues the patient did not recall.
Assessment of Functional Capacity
The assessment of the patient’s cardiopulmonary fitness or functional capacity is an integral component of the preoperative clinical examination. This information is typically used to estimate a patient’s risk for major postoperative morbidity or mortality, and to determine whether further preoperative testing is required. Importantly, suboptimal functional capacity is common in many economically advanced countries. For example, only one-fifth of American adults meet federal guidelines for recommended levels of aerobic and strengthening activity. Poor exercise capacity and cardiopulmonary disease have a bidirectional relationship. Specifically, lack of exercise may increase the risk of developing cardiopulmonary disease but preexisting cardiopulmonary disease can also prevent an individual from exercising. For example, patients with peripheral artery disease (PAD) may be limited by intermittent claudication, and patients with ischemic heart disease may be limited by exertional chest discomfort. There is a reasonable body of evidence demonstrating an association between poor preoperative functional capacity and increased perioperative risk. Most of these studies involved objective assessment of preoperative functional capacity using either exercise testing or cardiopulmonary exercise testing (CPET). Several other large studies have demonstrated association between very significant preoperative functional impairment (i.e., difficulty or inability to perform activities of daily living) and increased risks of postoperative mortality, cardiovascular complications, and pulmonary complications,
The challenge with assessment of preoperative functional capacity pertains to how best to assess it in usual clinical practice. Typically, the anesthesiologist will inquire about a patient’s general activity levels during the preoperative interview, and on that basis, make a subjective assessment of the patient’s functional capacity. Functional capacity is typically quantified in using the metabolic equivalent of task (MET), where one MET is approximately the rate of energy consumption at rest (3.5 mL/kg/min). A proposed scheme for estimating METs based on information from the preoperative interview is presented in Table 31.1 . There are important limitations to the usual clinical approach for this integral component of the preoperative evaluation. First, subjective assessment does not accurately estimate the patient’s true exercise capacity. In a multicenter prospective cohort study of 1401 patients undergoing major noncardiac surgery, anesthesiologists’ subjective assessment had only 19% sensitivity and 95% specificity for identifying patients’ inability to attain 4 or more METs during formal exercise testing. In addition, subjective assessment has poor correlation with standardized questionnaires that have been validated for measuring functional capacity. Second, subjective assessment has generally shown poor performance in predicting postoperative morbidity and mortality. In a single-center cohort study of 600 surgical patients, patients’ self-reported poor exercise capacity (defined as inability to walk four blocks and climb two flights of stairs) was associated with increased risk of serious perioperative complications, but the magnitude of the association was relatively weak (positive likelihood ratio of 1.3 and negative likelihood ratio of 0.6). For context, positive test results should have likelihood ratios greater than 2 to provide clinically meaningful information, whereas negative test results should have likelihood ratios of less than 0.5. Furthermore, in both a multicenter prospective cohort study and single-center retrospective cohort study, subjective assessment was a poor predictor of postoperative mortality and morbidity.
METs | Equivalent Level of Exercise |
---|---|
1 | Eating, working at computer, or dressing |
2 | Walking down stairs or in your house, or cooking |
3 | Walking 1 or 2 blocks on level ground |
4 | Raking leaves, gardening |
5 | Climbing 1 flight of stairs, dancing, or bicycling |
6 | Playing golf, or carrying clubs |
7 | Playing singles tennis |
8 | Rapidly climbing stairs, or jogging slowly |
9 | Jumping rope slowly, or moderate cycling |
10 | Swimming quickly, running or jogging briskly |
11 | Skiing cross country, or playing full-court basketball |
12 | Running rapidly for moderate to long distances |
∗ One metabolic equivalent of task (MET) is the amount of oxygen consumed while sitting at rest, and is equivalent to an oxygen consumption of 3.5 mL/min/kg body weight.
To improve preoperative evaluation of functional capacity, anesthesiologists should consider instead using structured questionnaires, such as the Duke Activity Status Index (DASI) ( Table 31.2 ). This 12-item self-administered questionnaire about activities of daily living has demonstrated correlation with gold-standard measures of functional capacity in surgical patients. Furthermore, DASI scores have been shown to improve prediction of postoperative cardiac complications following noncardiac surgery. While there is some varying opinion as to how DASI scores should be converted to METs, the original formula is presented below:
EstimatedMETS=(0.43×DASIscore)+9.63.5.
Can You | Points |
---|---|
|
|
|
|
|
|
|
|
|
|
|
|
|
|
|
|
|
|
|
|
|
|
|
|
Total score: |
Other alternatives for estimating functional capacity include simple exercise tests (e.g., 6-minute walk test, incremental shuttle walk test), exercise testing (e.g., electrocardiogram [ECG] exercise testing), or CPET. If using a standard exercise test (i.e., not CPET), extrapolation from the total treadmill time on a Bruce protocol exercise test results in overestimation of true exercise capacity. More importantly, resting left ventricular ejection fraction should not be used as a proxy measure of functional capacity.
Physical Examination
At a minimum, the preanesthetic examination should include vital signs (i.e., arterial blood pressure, heart rate, respiratory rate, oxygen saturation), height, and weight. Body mass index (BMI), which is calculated based on height and weight, is more informative than weight alone in establishing obesity. A scheme for classifying children and adults based on BMI is presented in Table 31.3 . Information pertaining to BMI can help identify individuals at risk for difficulties with airway management, and some chronic diseases (e.g., heart disease, diabetes mellitus, obstructive sleep apnea [OSA]). An ideal body weight should also be calculated, using available formulae such as the Devine equation. Information on ideal body weight can better inform dose selection for some anesthesia-related medications, and settings for positive pressure ventilation. Readily available online calculators can be used to quickly determine both BMI and ideal body weight. Patients often have increased arterial blood pressure during the preoperative visit, even without a prior history of hypertension. This finding may be caused by anxiety, or patients having forgotten to take their usual dose of antihypertensive medication. Thus, a single reading during the preoperative evaluation may not reflect the patient’s usual blood pressure control. Repeating the blood pressure measurement or obtaining previous readings, either by obtaining medical records (including prior ambulatory blood pressure testing) or asking patients about their “usual” blood pressure measurements are informative. Ideally, the referral documentation from the patient’s primary care physician or surgeon should include information on the patient’s usual blood pressure readings.
Body Mass Index | Weight Status |
---|---|
Adults Over 20 Years Old | |
BMI < 18.5 | Underweight |
BMI 18.5–24.9 | Normal |
BMI 25.0–29.9 | Overweight |
BMI 30.0 and above | Obese |
For Children and Teens | |
BMI-for-age < 5th percentile | Underweight |
BMI-for-age 5th percentile to < 85th percentile | Normal |
BMI-for-age 85th percentile to < 95th percentile | At risk of overweight |
BMI-for-age ≥ 95th percentile | Overweight |
From an anesthesiologist’s perspective, inspection of the airway may be the most important component of the physical examination (see Chapter 44 ). The components of the airway examination are presented in Box 31.1 . Documentation of an airway examination includes the Mallampati score ( Fig. 31.3 ), status of teeth, degree of neck mobility (especially extension), neck circumference (increased size predicts difficulty with laryngoscopy), thyromental distance, body habitus, and pertinent deformities. Because of the potential for dental injuries during anesthesia, any preexisting tooth abnormalities should be documented ( Fig. 31.4 ). In a French prospective cohort study of 1501 patients, the following characteristics were identified as independent predictors of difficult bag-mask ventilation: age more than 55 years, BMI more than 26 kg/m 2 , absence of teeth, presence of a beard, and a history of snoring. These risk factors are largely consistent with those identified in an American retrospective cohort study of 22,660 patients: age 57 years or more, BMI 30 kg/m 2 or greater, presence of a beard, Mallampati classification III or IV, severely limited mandibular protrusion, and a history of snoring. Other possible risk factors for difficult ventilation include an increased neck circumference, face and neck deformities (i.e., prior surgery, prior radiation, prior trauma, congenital abnormalities), rheumatoid arthritis, Trisomy 21 (Down syndrome), scleroderma, cervical spine disease, or previous cervical spine surgery. The physical examination must be supplemented by examination of previous anesthetic records, especially when there are indications of a potentially difficult airway. Patients with known difficult airways should be encouraged to obtain medical alert identification. When challenging airways are identified, advance planning ensures that necessary equipment and skilled personnel are available on the day of surgery. An evaluation of the heart, lungs, and skin is necessary, as well as further focus on organ systems involved with diseases reported by the patient. This evaluation should include cardiac auscultation, as well as inspection of arterial pulses, veins (peripheral and central), jugular venous distention, ascites, hepatomegaly, and peripheral edema. Inspection of peripheral veins can also help assess the ease of intravenous access. The auscultatory examination should assess for murmurs, abnormal heart sounds (e.g., third or fourth heart sounds), and pulmonary rales. If intravenous access sites are limited, possible central line placement can be discussed with the patient, or arrangements can be made for assistance from interventional radiology. Auscultation for carotid bruits is also important, especially in patients with a history of stroke, transient ischemic attacks (TIAs), or head and neck irradiation. The presence of a carotid bruit significantly increases the likelihood of an important lesion (i.e., 70%-99% stenosis) in both symptomatic or asymptomatic patients, but the absence of a bruit does not rule out carotid stenosis. Examination of the pulmonary system should include both auscultation (i.e., wheezing, decreased breath sounds, abnormal breath sounds) and inspection (i.e., cyanosis, clubbing, accessory muscle use, respiratory effort). A basic screening neurologic examination should document deficits in mental status, speech, gait, cranial nerve function, motor nerve function, and sensory nerve function. For selected patients (e.g., those with deficits, or scheduled to undergo neurosurgery), a more extensive or focused neurologic examination should document preexisting abnormalities that may aid in diagnosis or interfere with positioning. In addition, the definition of a baseline preoperative neurologic state helps determine whether any postoperative deficits represent new deficits versus preexisting abnormalities.
Length of upper incisors (concerning if relatively long)
Condition of the teeth
Relationship of maxillary incisors to mandibular incisors (concerning if there is prominent overbite)
Ability to advance mandibular incisors in front of maxillary incisors (concerning if unable to do this)
Interincisor or intergum (if edentulous) distance (concerning if < 3 cm)
Visibility of the uvula (concerning if Mallampati class is 3 or more)
Shape of uvula (concerning if highly arched or very narrow)
Presence of heavy facial hair
Compliance of the mandibular space (concerning if it is stiff, indurated, occupied by mass, or nonresilient)
Thyromental distance (concerning if < 6 cm)
Length of the neck
Thickness or circumference of the neck
Range of motion of the head and neck (concerning if unable to touch tip of chin to chest or cannot extend neck)


Frailty, Geriatric Conditions, and the Older Surgical Patient
As patients age, the accumulation of comorbid conditions puts them at increased risk for adverse outcomes after surgery. In addition, geriatric-specific risk factors such as functional and cognitive impairment are associated with poor postoperative outcomes. These clinical conditions, which do not fit into discrete disease categories, are often overlooked in routine preoperative assessments. In order to (1) accurately inform patients of their surgical risk and (2) identify targets for preoperative optimization, assessment of geriatric vulnerabilities is essential before surgery. The American College of Surgeons (ACS) and the American Geriatrics Society (AGS) have established best practice guidelines to guide the preoperative assessment of older surgical patients.
Geriatric-Focused Assessment in the Older Surgical Patient
Function and Mobility
Preoperative functional decline is associated with morbidity, mortality, and loss of function after surgery. Assessment of function prior to surgery is consequently essential for risk stratification and postdischarge planning in older surgical patients. Activities-of-daily-living (ADLs) assess the ability to perform basic tasks of selfcare, such as dressing, bathing, toileting, movement, continence, and eating. Instrumental ADLs determine an individual’s ability to live independently by the ability to complete shopping, laundry, transportation, finances, medications, food preparation, and housekeeping. To determine additional functional vulnerabilities, determination of vision deficits, hearing deficits, and swallowing difficulties should be routine. Mobility impairment can be efficiently screened by enquiring about a history of falls, determining fall risk, and performing a Timed-Up-And-Go test. The Timed-Up-And-Go test involves timing patients while they perform the following tasks in sequence:
- 1.
Stand up from a chair (without using arm rests if possible)
- 2.
Walk 10 feet
- 3.
Turn around and walk back to chair
- 4.
Sit down in chair
In a prospective cohort of older surgical patients, Timed-Up-And-Go test times were associated with risks of postoperative complications and 1-year mortality in a stepwise fashion.
Cognition
Preoperative cognitive impairment is strongly linked to delirium, complications, functional decline, and death after surgery. The Mini-Cog test (available at https://mini-cog.com ), which consists of a three-item recall test and clock draw test, is an efficient tool to screen for preoperative cognitive impairment. Additional information about potential cognitive deficits should be elicited from someone who knows the patient well. Importantly, mild cognitive impairment that is not clinically apparent may have a critical impact on decision-making capacity. Patients should be able to describe, in their own words, the essential elements of the consent discussion—including the surgical condition, indications for surgery, risks, benefits, and alternatives to surgery. It is important to note the four legal criteria for medical decision-making capacity, namely that the patient can (1) clearly indicate his/her treatment choice; (2) understand the relevant information communicated by the physician; (3) acknowledge his/her medical condition, treatment options, and expected outcomes; and (4) engage in a rational discussion about treatment options.
Nutrition
In general, most surgeons and anesthesiologists are keenly aware of the role nutritional status plays in recovery after surgery for all surgical patients. The most common adverse events related to poor nutritional status are infectious complications (i.e., surgical site infections, pneumonia, urinary tract infections), wound complications (i.e., dehiscence, anastomotic leaks), and increased length of stay. The ACS National Surgical Quality Improvement Program (NSQIP) and AGS Best Practice Guidelines recommend the following steps to screen for poor nutritional status (older adults with limited material resources are at particular risk for food insecurity).
- 1.
Document height, weight, and BMI
A BMI value less than 18.5 kg/m 2 places an individual at elevated risk and should prompt referral for nutritional assessment.
- 2.
Measure baseline serum albumin and prealbumin concentrations
Serum albumin concentration less than 30 g/L (in the absence of hepatic or renal dysfunction) should prompt referral for nutritional assessment.
- 3.
Inquire about unintentional weight loss in the last year
Unintentional weight loss exceeding 10% to 15% of baseline weight within the prior 6 months is associated with severe nutritional risk and should prompt referral for nutritional assessment.
Frailty
Frailty, which is defined as a state of increased vulnerability to physiologic stressors, is associated with adverse health outcomes after medical and surgical interventions and limited life expectancy. Numerous validated instruments measuring frailty are in current use in research and clinical practice. There are two primary models of frailty—the frailty phenotype and the deficit accumulation model. The frailty phenotype described by Fried and colleagues is based on the identification of traits associated with the occurrence of disease, hospitalization, falls, disability, and death in a large prospective cohort study. This study defined the determinants of frailty as weight loss, exhaustion, physical activity, walk time, and grip strength. The deficit accumulation model of aging (based on data from the Canadian Study of Health and Aging) identified 92 signs, symptoms, functional impairments, and laboratory abnormalities that are proportionally weighted into a frailty index for predicting mortality.
While numerous studies have demonstrated the association of patient frailty with adverse surgical outcomes, the incorporation of a patient’s level of frailty into routine clinical practice has not been broadly implemented. Many frailty instruments require special training, input of laboratory values or clinical data, and a fair amount of time to complete; hence, they are impractical in a busy clinical setting. A recent survey of surgical oncologists reported that although most surgeons expressed interest in preoperative optimization for older patients, only 6% currently perform geriatric assessments in their older patients. Factors contributing to the low level of adoption include perceptions regarding the amount of time required to assess for vulnerabilities, and lack of specific programs designed to address them within typical clinical practices. To address this gap, several investigators have validated efficient strategies to measure frailty in clinical practice. Robinson and colleagues developed two alternative frailty assessment definitions for surgical patients, which were
- ▪
Mini-Cog score of 3 or less, serum albumin concentration of 30 g/L or less, one or more falls in the prior 6 months, and hematocrit under 35%.
- ▪
Timed-Up-And-Go test 15 or more seconds, activity-of-daily-living dependence, and Charlson comorbidity index score 3 or greater.
Frailty identified with the Edmonton Frail Scale (EFS) has been shown to be associated with adverse outcomes after surgery. The EFS can be administered by an individual with no formal medical education, and has been validated in comparison to the geriatric specialist’s comprehensive geriatric assessment.
Additional Considerations
Anxiety, depression, substance abuse, and social isolation are common underdiagnosed conditions in older adults. Careful screening can identify these potential barriers to recovery, safe discharge after surgery, and maintenance of independence. Up to 11% of the population 71 years of age or more in the United States suffer from depression. The Patient Health Questionnaire-2 is an efficient instrument to screen for depression that includes only two questions: “ In the past 12 months, have you ever had a time when you felt sad, blue, depressed, or down for most of the time for at least two weeks?” and “ In the past 12 months, have you ever had a time, lasting at least two weeks, when you didn’t care about the things that you usually care about or when you didn’t enjoy the things that you usually enjoy?” Among individuals aged 65 years or more, 13% of males and 8% of females consume at least two alcoholic drinks per day. Alcohol and substance abuse are associated with increased rates of postoperative mortality and complications including pneumonia, sepsis, wound infection and disruption, and prolonged length of stay. Consequently, the ACS NSQIP and AGS recommend screening for alcohol and substance abuse among older individuals with the modified CAGE (acronym of four clinical interview questions: cutting down, annoyance by criticism, guilty feeling, and eye-openers) questionnaire (see section on “Patients With a History of Substance Abuse”).
Preoperative Optimization for Frail Geriatric Patients ( Table 31.4 )
One goal of the preoperative assessment of elderly surgical patients is to identify potentially modifiable risk factors—such as malnutrition, poor physical function, anxiety, and social isolation—in order to optimize surgical outcomes. Several recent geriatric prehabilitation models have emerged to help meet this need, with some demonstrating promising results. One of the first of such programs was the Proactive Care of Older People undergoing surgery (POPS) before-and-after study in United Kingdom. The overarching aim of this project was to decrease complications and hospital length of stay among at-risk older adults undergoing elective surgery. The authors performed a structured geriatric team intervention that identified at-risk patients, and then facilitated coordinated multidisciplinary optimization of geriatric vulnerabilities. The interventions included preoperative home visits by occupational and physical therapists, social worker inputs, nutrition education, and relaxation techniques. In addition to preoperative optimization, interdisciplinary teams participated in daily inpatient rounds, weekly multidisciplinary meetings, and bi-weekly ward rounds led by a consultant or clinical nurse specialist. Compared to historical controls, surgical patients who received the POPS intervention experienced fewer postoperative complications (e.g., pneumonia, delirium), improved pain control, lower rates of delayed mobilization, lower rates of inappropriate urinary catheter use, and shorter hospital length of stay.
Domain | Assessment | Preoperative Optimization |
---|---|---|
Cognition |
|
|
Function |
|
|
Nutrition |
|
|
Similar findings were observed in the more recently reported Perioperative Optimization of Senior Health study, which was another before-and-after study. This preoperative interdisciplinary clinic evaluated at-risk patients (either aged age ≥ 80 years, or aged ≥ 65 years with concurrent geriatric vulnerability) and designed targeted optimization. Compared to historical controls, the combination of geriatric prehabilitation and inpatient geriatric comanagement were associated with reduced hospital length of stay, reduced readmission rates, and increased rates of discharge to home with self-care. Another example of a promising prehabilitation program is the Michigan Surgical Home and Optimization Program, which is a structured home-based preoperative training program with physical, nutritional, and psychological interventions. The intervention included (1) a home-based walking program with daily reminders and feedback; (2) incentive spirometry instructions starting 1 week prior to surgery; (3) education on nutrition, stress management, and care planning; and (4) resources for smoking cessation, when appropriate. Compared to matched historical controls, patients enrolled in the program experienced reductions in both hospital length of stay and healthcare costs. While promising, these studies do have methodological weakness as before-and-after studies, as opposed to methodologically more robust parallel arm randomized trials, cluster randomized trials, or stepped-wedge trials (see Chapter 90 ). Nonetheless, the consistency in findings across the studies is supportive of the notion that attention to preoperative and perioperative assessment in the older population can result in improved postoperative outcomes that benefit patients, hospitals, and healthcare systems.
Decision Making for Surgery in Older Adults
The critical initial step in surgical decision making for the older patient is an assessment of the patient’s decision-making capacity. Among older adults, only about 3% lack medical decision-making capacity. However, among older adults with mild cognitive impairment, this proportion is as high as 20%. A Mini-Cog exam (see section on “Cognition”) is an efficient way to screen for cognitive impairment in the surgical setting. If the patient does not have capacity, treatment goals and choices should be discussed with the patient’s surrogate, with patient involvement as appropriate. Even cognitively intact patients may have difficulty grasping the risks and tradeoffs involved in decisions for surgery. The “teach-back” method (i.e., asking patients to communicate back information about their diagnosis, treatment plan, and potential risks of treatment) may be useful to confirm that the risks, expected benefits, and alternatives to surgery are fully understood. Various resources are available to aid in using the teach-back method (e.g., http://www.teachbacktraining.org ).
Collaborative decision making is essential in older adults with limited life expectancy. Rather than a traditional conversation that focuses on the process of correcting the underlying surgical condition, the conversation should be driven by a focus on the older adult’s overarching health goals. Using open-ended questions, physicians elicit the patient’s global healthcare goals that may prioritize (1) life prolongation; (2) function and independence; (3) maintenance of cognition; or (4) comfort. Further discussion should be framed by the patient’s most important health goal. A frank, realistic, and granular conversation about the ability of surgery to satisfy healthcare goals and priorities is essential. Invasive treatments should only be considered if there is a realistic possibility that overarching health goals can be achieved. These goals, however, are often dynamic and must be revisited as conditions change. Often, primary physicians who have longitudinal relationships with patients can provide important insight into their patient’s health goals and should be included in this important deliberation. To assist in high-risk surgical decision making, preoperative palliative care consultation should be considered in individuals with poor prognoses who are electing to undergo surgery, particularly if they have a life expectancy of less than 6 months.
Preoperative Evaluation of Patients With Coexisting Disease
For some conditions commonly seen in the preanesthetic assessment clinic, preoperative optimization, testing, and intervention may be important (see also Chapter 32). Identification of these comorbid conditions might be an opportunity for the anesthesiologist to intervene to decrease perioperative risk. These conditions are best managed before the day of surgery, thus allowing ample time for thoughtful evaluation, consultation, and planning.
Cardiovascular Disease
Cardiovascular complications are serious perioperative adverse events that account for about 45% of all deaths within 30 days after major noncardiac surgery. These events occur relatively frequently. For example, the multicenter Vascular Events in Noncardiac Surgery Patients Cohort Evaluation prospective cohort study found a 4% risk of myocardial infarction, 17% risk of prognostically important myocardial injury, and 0.7% risk of acute heart failure during the 30 days following major noncardiac surgery. Evidence-based preoperative cardiovascular assessment guidelines have now been published in several countries, including the 2014 American College of Cardiology (ACC) and American Heart Association (AHA) guidelines, 2014 European Society of Cardiology (ESC) and ESA guidelines, 2017 Canadian Cardiovascular Society (CCS) guidelines, 2017 Brazilian Society of Cardiology guidelines, and 2014 Japanese Circulation Society guidelines. While the guidelines provide generally similar guidance, there are some important differences that will be highlighted in the sections below.
Hypertension
Based on revised 2017 ACC/AHA guidelines, hypertension is defined as a blood pressure greater than 130/80 based on appropriately measured arterial blood pressure. About 45% of adults in the United States have hypertension, with a similar prevalence in other countries. While most individuals with hypertension have primary (or essential) hypertension, there are several important causes of secondary hypertension, including primary renal disease, OSA, pheochromocytoma, renovascular hypertension, Cushing syndrome, hyperthyroidism, and coarctation of the aorta. Hypertension leads to significantly increased risks of left ventricular hypertrophy (LVH), heart failure, ischemic heart disease, chronic kidney disease (CKD), ischemic stroke, intracerebral hemorrhage, and PAD. The duration and severity of hypertension are highly correlated with subsequent end-organ damage, morbidity, and mortality. These risks appear to increase once blood pressure exceeds 117/75, with each subsequent 20 mm Hg increase in systolic blood pressure and 10 mm Hg increase in diastolic blood pressure being associated with a two-fold increase in the risk of stroke and cardiovascular death. In the perioperative setting, hypertension is associated with increased risks of postoperative death and myocardial infarction, but the magnitude of this association is relatively weak (odds ratio 1.35; 95% confidence limits, 1.17-1.56).
In patients with hypertension, the goals of preoperative evaluation are to identify any secondary causes of hypertension, presence of other cardiovascular risk factors (e.g., smoking, diabetes mellitus), and evidence of end-organ damage. For example, paroxysmal hypertension or hypertension in young individuals should prompt a search for hyperthyroidism, illicit drug use (e.g., cocaine, anabolic steroids), and coarctation of the aorta. Similarly, a history of paroxysmal hypertension associated with episodic tachycardia and palpitations should raise suspicions about an underlying pheochromocytoma (see next section). The physical examination should focus on vital signs (including blood pressure measured in both arms), thyroid gland, peripheral pulses, and cardiovascular system (including bruits and signs of intravascular volume overload). Additional specialized testing is directed by the initial clinical evaluation. For example, patients with long-standing, severe, or poorly controlled hypertension should undergo an ECG and blood sampling to measure creatinine concentration. Individuals on diuretic antihypertensives (e.g., chlorthalidone, hydrochlorothiazide) may require evaluation of electrolytes. Patients with suspected hyperthyroidism will require thyroid function tests.
While preoperative hypertension is associated with an increased risk of cardiovascular complication, this association is generally not evident for systolic blood pressure values less than 180 mm Hg or diastolic blood pressure values less than 110 mm Hg. Additionally, there is no compelling data that delaying surgery to optimize blood pressure control will result in improved outcomes. Accordingly, some international practice guidelines support proceeding with surgery if the systolic blood pressure is less than 180 mm Hg and diastolic blood pressure is less than 110 mm Hg. Usual antihypertensive medication treatment should be continued in these patients during the perioperative period. For patients with severe hypertension (i.e., diastolic blood pressure > 110 mm Hg or systolic blood pressure > 180 mm Hg), anesthesiologists should weigh the potential benefits of delaying surgery to optimize antihypertensive treatment against the risks of delaying the procedure. In general, all long-term antihypertensive treatment should be continued up to the day of surgery, with the possible exception of angiotensin-converting enzyme inhibitors (ACEIs) and angiotensin receptor blockers (ARBs). Administration of these medications within 24 hours before surgery is consistently associated with increased risks of intraoperative hypotension, and possibly associated with elevated risks of postoperative myocardial injury. Accordingly, it is reasonable to withhold these medications for 24 hours before surgery, provided that they are restarted postoperatively once patients are hemodynamically stable. Importantly, failure to resume ACEI and ARB therapy postoperatively is itself associated with adverse outcomes.
Even if surgery is not necessarily delayed to facilitate improved blood pressure control, the preanesthesia evaluation should also be viewed as an excellent opportunity to alter long-term consequences of diseases. Thus, appropriate postsurgery referrals should be made to facilitate improved long-term management of hypertension.
Ischemic Heart Disease
Ischemic heart disease (IHD) afflicts about 16.5 million adults in the United States, and 111 million people worldwide. It also accounts for about 13% of all deaths, both in the United States and worldwide ( http://www.who.int.easyaccess2.lib.cuhk.edu.hk/mediacentre/factsheets/fs317/en/ ). While death rates attributable to IHD are declining in many high-income countries, other regions continue to experience high or increasing rates of IHD mortality. IHD mediates adverse effects because of its immediate impact (e.g., myocardial infarction, sudden cardiac death) and related diseases (e.g., heart failure, atrial fibrillation). Therapy for IHD includes antiplatelet therapy (e.g., aspirin, adenosine diphosphate receptor [P2Y 12 ] inhibitor), renin angiotensin system inhibitors (e.g., ACEI, ARB), β-adrenergic blockers, other antianginal therapies (e.g., calcium channel blocker, nitrates), lipid reducing agents (e.g., statins), and coronary revascularization with either coronary artery bypass grafting (CABG) or percutaneous coronary intervention (PCI). Based on recent randomized controlled trials, several newer types of medical therapy for IHD may be increasingly used, including novel antiinflammatory therapy (e.g., canakinumab), low-dose direct oral anticoagulant (DOAC) therapy, and antibody-based lipid-lowering agents. Coronary revascularization—specifically with CABG—improves survival compared to medical therapy (pooled relative risk 0.80, 95% limits 0.70-0.91) in several high-risk IHD states, namely left main coronary artery stenosis, triple-vessel coronary artery disease, and two-vessel coronary artery disease with proximal left anterior descending artery stenosis. When PCI is used instead for these high-risk states, PCI with a newer generation drug eluting stent (DES) may also confer a survival benefit, albeit with marginal statistical significance. In patients who meet indications for revascularization, CABG improves survival more than PCI in multivessel disease that is associated with either diabetes mellitus or higher coronary artery lesion complexity. Notably, aside from these high-risk states (e.g., triple vessel coronary artery disease), PCI has not been shown to convincingly improve survival in stable IHD.
In the perioperative setting, IHD is a risk factor for myocardial infarction and a prognostically important myocardial injury after surgery. It is also associated with elevated risks of 30-day postoperative mortality, especially if a patient had experienced a myocardial infarction, acute coronary syndrome, or severe angina (i.e., occurring at effort levels less than walking one to two blocks or climbing one flight of stairs) within the 6 months preceding surgery. Surgical patients with IHD may also have important comorbidities with important perioperative implications, such as heart failure and atrial fibrillation (see sections of “Heart Failure” and “Atrial Fibrillation”). The goals of preoperative evaluation are to (1) ascertain whether the patient has previously undiagnosed significant IHD; (2) characterize any known IHD with respect to severity, functional limitations, therapy, and prior investigations; (3) determine whether additional preoperative specialized testing or consultations are warranted; and (4) identity opportunities for reducing perioperative risk related to IHD. In the case of patients without known IHD, evaluation of traditional risk factors for IHD (i.e., smoking, hypertension, increased age, male sex, hyperlipidemia, family history) is important when significance of suspicious symptoms (e.g., chest discomfort, dyspnea) or abnormal ECG. In patients with known IHD, the anesthesiologist should characterize any history of chest discomfort (i.e., pain, pressure, tightness) with respect to its duration, precipitating factors, associated symptoms, and relieving factors. Exertional dyspnea may represent an angina equivalent but is also a nonspecific finding that might be related to physical deconditioning, pulmonary disease, or heart failure. It is useful to classify any angina based on the CCS grading scale:
- ▪
CCS class I: Ordinary physical activity (e.g., walking, climbing stairs) does not cause angina. Angina with strenuous or rapid or prolonged exertion at work or recreation
- ▪
CCS class II: Slight limitation of ordinary activity. No angina is precipitated by walking more than two blocks on level ground or climbing more than one flight of stairs at a normal pace and in normal conditions. Angina is only precipitated by walking or climbing stairs rapidly, walking uphill, and walking or stair climbing under challenging conditions (e.g., after meals, in cold, in wind, under emotional stress, during the few hours after awakening).
- ▪
CCS class III: Marked limitation of ordinary physical activity. Angina precipitated by walking one or two blocks on level ground and climbing one flight of stairs in normal conditions and at normal pace.
- ▪
CCS class IV: Inability to carry on any physical activity without discomfort. Angina may be present at rest.
Patients with risk factors for IHD or suspicious symptoms may require an ECG, especially before intermediate-risk or high-risk surgical procedures. Routine preoperative ECGs are not indicated ( Box 31.2 ), especially in asymptomatic patients without known cardiovascular disease or risk factors. While specific preoperative abnormalities are associated with increased perioperative cardiac risk (e.g., bundle branch blocks [BBBs]), these abnormalities do not enable clinicians to identify patients with increased perioperative cardiac risk more accurately when considered in combination with known clinical risk factors. Establishing a baseline for postoperative comparison is often the most important reason to obtain a preoperative ECG; however, this decision should be based on the patient’s likely risk of postoperative cardiovascular complications. Thus, a baseline ECG is unlikely to be helpful in an individual at very low risk for postoperative cardiac events. If a previous ECG is available from the previous 3 months and there has been no intervening change in clinical status, a repeat ECG is likely not needed. Other typical preoperative laboratory tests that may be considered for patients with known or suspected IHD include creatinine and hemoglobin concentrations. Both chronic renal insufficiency and anemia are risk factors for perioperative cardiac complications. In addition, anemia can modify the effects of β-adrenergic blockade in surgical patients, with evidence of increased harm when used in patients with perioperative anemia or significant bleeding.
Class IIa Recommendation: It Is Reasonable to Perform the Procedure
Preoperative resting 12-lead ECG is reasonable for patients with known IHD, significant arrhythmia, PAD, CVD, or other significant structural heart disease (except if undergoing low-risk surgical procedures).
Class IIb Recommendation: The Procedure May Be Considered
Preoperative resting 12-lead ECG may be considered for asymptomatic patients without known coronary heart disease, except for those undergoing low-risk surgical procedures.
Class III Recommendation: The Procedure Should Not Be Performed Because It Is Not Helpful
Routine preoperative resting 12-lead ECG is not useful for asymptomatic patients undergoing low-risk surgical procedures.
CVD, Cerebrovascular disease; ECG, electrocardiogram; IHD, ischemic heart disease; PAD, peripheral artery disease.
Preoperative cardiac risk assessment algorithms have been proposed by several practice guidelines, including the ACC/AHA guidelines ( Fig. 31.5 ) and the CCS guidelines ( Fig. 31.6 ). These algorithms have somewhat differing target populations. The ACC/AHA algorithm encompasses patients with known IHD or associated risk factors who are having noncardiac surgery. The CCS guidelines focus on adults (≥18 years old) having inpatient surgery who are either 45 years or older, or who have significant cardiovascular disease (i.e., IHD, cerebrovascular disease [CVD], PAD, heart failure, severe pulmonary hypertension, severe obstructive cardiac valvular disease). While the algorithms have many fundamental similarities, they also have several key differences, which will be discussed below. Importantly, the algorithms should always be viewed as flexible guidance frameworks that should be tailored, as needed, to individual patients. The initial step in these risk assessment algorithms is consideration of the urgency of the planned surgery. The 2014 ACC/AHA guidelines define an emergency procedure as one where life or limb would be threatened if surgery did not proceed within 6 hours or less; an urgent procedure as one where life or limb would be threatened if surgery did not proceed within 6 to 24 hours; and a time-sensitive procedure as one where delays exceeding 1 to 6 weeks would adversely affect outcomes (e.g., most oncology surgery). Based on this classification scheme, patients should proceed directly to any required emergency surgery without further preoperative cardiac assessment. For these individuals, the focus should be on surveillance (e.g., serial cardiac enzymes, hemodynamic monitoring, serial ECGs) and early treatment of any postoperative cardiovascular complications.


In the second step, active cardiac conditions—such as acute coronary syndromes, decompensated heart failure, severe valvular disease (e.g., critical aortic stenosis), suspected significant pulmonary hypertension, or significant arrhythmias (e.g., atrial fibrillation with rapid ventricular rate, sustained ventricular tachycardia)—should be ruled out in patients not requiring emergency surgery. If any of these conditions are present, they must be initially treated, after which the original planned surgery can be reconsidered based on its risk-benefit balance. When assessing whether a patient has an active cardiac condition, the anesthesiologist should inquire about any recent myocardial infarction. In a study of about 560,000 patients who underwent major noncardiac surgery in the United States, the risk of 30-day postoperative myocardial infarction or death was significantly elevated when surgery was performed within 60 days after a prior myocardial infarction. Thus, the 2014 ACC/AHA guidelines recommend deferring nonurgent surgery until 60 days after a recent myocardial infarction.
In the third step, perioperative cardiac risk should be estimated based on readily available clinical information that encompasses both patient-level (e.g., comorbidities) and surgery-level (e.g., procedure type) characteristics. The 2014 ACC/AHA guidelines and 2014 ESC/ESA guidelines recommend using clinical risk indices, namely the Revised Cardiac Risk Index (RCRI) ( Table 31.5 ), ACS NSQIP surgical risk calculator ( https://riskcalculator.facs.org/RiskCalculator ), or NSQIP Myocardial Infarction and Cardiac Arrest risk calculator. If these indices find the estimated risk of postoperative myocardial infarction or death to be less than 1% (consistent with RCRI ≤ 1), the ACC/AHA guidelines recommend that patients simply proceed directly to surgery. The 2017 CCS guidelines suggest using the RCRI, as opposed to the NSQIP risk calculators, largely because the NSQIP-risk models were developed using a database without routine postoperative troponin surveillance, implying that the predicted absolute myocardial infarction rate might underestimate the true rate by three-fold, and have not been externally validated. In addition, the NSQIP risk calculators have largely not been externally validated. By comparison, the RCRI was derived in a cohort study with standardized cardiac biomarker surveillance, and also has undergone extensive external validation. Nonetheless, the RCRI has limitations, especially with respect to inadequate consideration of variations in cardiac risk across different surgical procedures. The CCS guidelines recommend that patients proceed directly to surgery if they meet all the following criteria: age over 65 years, RCRI score of 1 or greater, and no history of significant cardiovascular disease (i.e., coronary artery disease, CVD, PAD, heart failure, pulmonary hypertension, or severe obstructive cardiac valvular disease).
Components of Revised Cardiac Risk Index ∗ | Points Assigned |
---|---|
High-risk surgery (intraperitoneal, intrathoracic, or suprainguinal vascular procedure) | 1 |
Ischemic heart disease (by any diagnostic criteria) | 1 |
History of congestive heart failure | 1 |
History of cerebrovascular disease | 1 |
Diabetes mellitus requiring insulin | 1 |
Creatinine > 2.0 mg/dL (176 μmol/L) | 1 |
∗ Data from Lee TH, Marcantonio ER, Mangione CM, et al. Derivation and prospective validation of a simple index for prediction of cardiac risk of major noncardiac surgery. Circulation . 1999;100:1043–1049.
† Data from Devereaux OJ, Goldman L, Cook DJ, et al. Perioperative cardiac events in patients undergoing noncardiac surgery: a review of the magnitude of the problem, the pathophysiology of the events and methods to estimate and communicate risk. CMAJ . 2005;173:627–634.
‡ Defined as cardiac death, nonfatal myocardial infarction, or nonfatal cardiac arrest.
In the fourth and subsequent steps, there are substantial differences between the American versus Canadian preoperative risk assessment algorithms. The ACC/AHA algorithm recommends that a patient with a functional capacity of 4 or more METs should proceed directly to surgery. This guidance is largely based on older studies of preoperative exercise testing, and more recent studies of preoperative CPET, which found an association between poor functional capacity and elevated perioperative cardiac risk. The major clinical challenge with this recommendation pertains to how best to estimate patients’ preoperative functional capacity in clinical practice (see earlier section on “Assessment of Functional Capacity”). Simple subjective assessment of functional capacity based on the usual preoperative history does not accurately estimate true exercise capacity, and does not accurately predict postoperative cardiovascular complications. Thus, in clinical practice, anesthesiologists should generally use a structured questionnaire, especially the DASI (see Table 31.2 ), with consideration of formal exercise testing if the results would alter management. Notably, DASI scores improve the accuracy of prediction of cardiac complications following noncardiac surgery beyond that achieved with the RCRI alone.
If a patient is deemed to have either low (i.e., < 4 METs) or uncertain functional capacity, the AHA/ACC guidelines recommend consideration of exercise or pharmacologic cardiac stress testing, if the results could plausibly inform decision making or clinical care. Patients with high-risk abnormal test results can be considered for subsequent coronary angiography, and possibly revascularization (if usual nonoperative indications for coronary revascularization are met). Importantly, the ACC/AHA guidelines emphasize the importance of considering alternative less invasive or nonsurgical treatment for the underlying condition, especially in patients found to be at very high cardiac risk.
As opposed to assessing functional capacity, the CCS guidelines recommend preoperative risk assessment with cardiac biomarkers, specifically brain natriuretic peptide (BNP) or N-terminal-pro-BNP (NT pro-BNP). These neurohormones are secreted by the cardiac ventricles in response to stretch or ischemia of the atrial and ventricular walls. In the nonoperative setting, elevated natriuretic peptide concentrations are powerful markers of cardiovascular risk in individuals with IHD or associated risk factors, as well as in individuals with heart failure. Interestingly, there is only slight-to-fair correlation between plasma levels of these biomarkers and measures of exercise capacity, suggesting that natriuretic peptides measure a different patient characteristic. An individual patient data meta-analysis of 18 studies in noncardiac surgery found that preoperative natriuretic peptide concentrations can differentiate patients based on their perioperative cardiac risk ( Table 31.6 ). In general, BNP concentrations less than 100 ng/L or NT pro-BNP concentrations less than 300 ng/L are indicative of a patient at very low perioperative cardiac risk. Conversely, BNP concentrations above 300 ng/L or NT pro-BNP concentrations above 900 ng/L are indicative of a patient at high cardiac risk. Importantly, natriuretic peptides also improve the accuracy of risk estimation beyond that achieved with traditional clinical risk factors alone. The CCS guidelines recommend that preoperative BNP or NT pro-BNP testing be used to inform the required level of postoperative surveillance. Specifically, they recommend routine troponin surveillance for 48 to 72 hours after surgery in patients with elevated preoperative BNP (≥92 ng/L) or NT pro-BNP (≥300 ng/L) concentrations. No other preoperative testing or intervention is recommended based on the results of preoperative cardiac biomarker testing.
Preoperative BNP Concentration (ng/L) | Likelihood Ratio ∗ for Death or MI | Preoperative NT pro-BNP Concentration (ng/L) | Likelihood Ratio ∗ for Death or MI |
---|---|---|---|
0–99 | 0.6 | 0–300 | 0.4 |
100–250 | 1.4 | 301–900 | 1.5 |
>250 | 3.9 | 901–3000 | 2.7 |
>3000 | 5.0 |
∗ Positive test results should have likelihood ratios > 2 to provide clinically meaningful information, whereas negative test results should have likelihood ratios of < 0.5.
Although current evidence pertaining to risk assessment with preoperative natriuretic peptides is promising, there are also some important limitations. First, the event rate for death or myocardial infarction in this individual patient data meta-analysis was about 11%, which is considerably higher than would be expected in usual clinical practice. This high event rate is explained, in part, by the intermediate-to-high risk characteristics of the study sample, and by two large included studies that defined cardiovascular complications based on elevated troponin concentrations alone (as opposed to less frequent myocardial infarction events). Therefore, further research remains needed to determine biomarker threshold levels and prognostic accuracy in generalizable study samples. Second, while elevated natriuretic peptide concentrations are indicative of elevated perioperative cardiac risk, they do not indicate underlying pathophysiological mechanism. Aside from ischemia and heart failure, other prognostically important conditions can cause elevated natriuretic peptide concentrations, including right ventricular dysfunction, cardiac valvular disease, and atrial fibrillation. Thus, further specialized testing (e.g., echocardiography) may be helpful in some patients with high preoperative BNP or NT pro-BNP concentrations, if the results might inform clinical care or decision making. Third, nonoperative data suggest natriuretic peptides have limitations as prognostic biomarkers in certain disease states, including obesity and chronic renal kidney.
The anesthesiologist has several other avenues for further investigating or optimizing known or suspected IHD before surgery—including consultations, biomarkers, stress testing, coronary angiography, coronary revascularization, and medical therapy. When considering the need for additional consultations before surgery, an initial phone call to the primary care physician or cardiologist often yields important information that obviates the need for further consultation. Any specialist consultation (e.g., cardiologist) initiated by the anesthesiologist should seek specific advice regarding the diagnosis, treatment, or further optimization of the patient’s condition. It is preferable to ask specific questions, such as “ Does this patient have IHD ?” or “ Is this patient optimized for planned radical nephrectomy?, ” to avoid unhelpful consultation reports that simply state that a patient is “ cleared for surgery. ”
Aside from BNP and NT pro-BNP, several other preoperative biomarkers have shown promise, with the most compelling data pertaining to high-sensitivity troponin assays. In the nonoperative setting, these high-sensitivity assays have demonstrated subtle resting elevations in cardiac troponin concentrations in an important segment of the population without any acute coronary syndrome. These individuals have significantly elevated risks of death, as well as progression to IHD or heart failure. Perhaps unsurprisingly, many otherwise stable surgical patients may have elevated troponin concentrations even before surgery. For example, in a cohort study of 325 patients undergoing major inpatient noncardiac surgery, about 20% had a preoperative high-sensitivity troponin T concentration exceeding the 99th percentile for the assay. Thus, especially in patients for whom postoperative troponin surveillance is planned, a preoperative troponin measurement is integral to determining whether any elevated postoperative concentration reflects acute injury versus chronic long-term elevation. Two large cohort studies have also shown that these preoperative troponin elevations may aid in risk stratification for noncardiac surgery. Specifically, a preoperative high-sensitivity troponin T concentration above 14 ng/L is associated with increased risks of death and cardiovascular complications after major noncardiac surgery. Furthermore, addition of preoperative high-sensitivity troponin T appears to improve the accuracy of risk estimation beyond that achieved with traditional clinical risk factors and natriuretic peptides. Further research remains needed to confirm these initial results, establish ideal screening thresholds, and replicate these analyses using other high-sensitivity troponin assays. Importantly, prognostically important conditions other than myocardial ischemia can cause elevated troponin concentrations, including right ventricular dysfunction, cardiac valvular disease, and atrial fibrillation.
When supplementing preoperative evaluation, cardiac stress testing can help diagnose IHD, assess its severity, and estimate perioperative cardiac risk. The tests therefore address both diagnosis and prognosis . Several stress tests are available that differ based on the stress modality (i.e., exercise, pharmacologic) and ischemia monitoring method (i.e., ECG, perfusion imaging, echocardiography). An exercise stress test is a preferred option for a patient who is capable of exercising and likely to achieve an adequate heart rate response during exercise. Exercise stress testing also allows for an objective measurement of functional capacity. An adequate test result is obtained when patients can exercise to 85% of their target heart rate (i.e., 220 minus age). Pharmacologic stress modalities (e.g., dobutamine, dipyridamole, adenosine, regadenoson) are indicated for an individual who cannot exercise or is unlikely to achieve an adequate heart rate response because of pacemakers, significant bradycardia, or high-dose negative chronotropic drugs (e.g., β-adrenergic blockers). The choice of pharmacologic stress modality is generally immaterial, but there are some exceptions. For example, since dobutamine uncovers ischemia by increasing contractility, heart rate, and blood pressure, it may not be the best choice in patients with pacemakers, significant bradycardia, aortic aneurysms, cerebral aneurysms, or poorly controlled hypertension. While adenosine and dipyridamole rely on their vasodilatory properties and do not depend on a heart rate response, they may exacerbate bronchospasm in patients taking theophylline. In addition, these drugs may cause dangerous reductions in preload in the presence of severe stenotic valvular heart disease.
An exercise ECG stress test is a reasonable option for a patient with a relatively normal baseline ECG who is capable of exercising with an adequate heart rate response. An imaging monitoring method (i.e., echocardiography, myocardial perfusion imaging) can be used instead if a patient has significant ECG abnormalities (e.g., left bundle branch block [LBBB], LVH with strain pattern) that may interfere with electrocardiographic detection of ischemia. Nonetheless, exercise myocardial perfusion imaging may still be problematic in patients with LBBB, because of false-positive results related to septal perfusion defects. Stress echocardiography assesses for wall motion abnormalities both at rest and under stress conditions (i.e., exercise, dobutamine). Resting abnormalities indicate scar tissue from a prior infarction, while new abnormalities under stress conditions (i.e., inducible wall motion abnormalities) indicate limited blood due to stenotic coronary lesions. Nuclear myocardial perfusion imaging detects ischemia by comparing radioisotope uptake by viable myocardium under resting and stress conditions. Perfusion defects at rest are indicative of a prior infarction. Since normal coronary arteries vasodilate with exercise or specific pharmacologic stressors (i.e., adenosine, dipyridamole), normal myocardium maintains normal radioisotope uptake in stress conditions. By comparison, stenotic vessels are maximally vasodilated at rest, and unable to further vasodilate under stress conditions. Thus, myocardium with flow-limiting lesions has normal radioisotope update at rest, but decreased uptake under stress conditions (i.e., reversible perfusion defects). In general, the choice of test should be informed by patient factors (e.g., ability to exercise) and local expertise in cardiac stress testing.
Cardiac stress tests can also help predict whether patients are likely to experience perioperative cardiac complications. Since these events occur relatively infrequently, the prognostic performance of these tests should not be evaluated based on positive or negative predictive values. It is preferable to use positive likelihood ratio and negative likelihood ratio values, which can be readily calculated using sensitivity and specificity values.
Positivelikelihoodratio=sensitivity1−specificity
Negativelikelihoodratio=1−sensitivityspecificity
There are relatively few data on the prognostic performance of preoperative exercise ECG stress testing. In a metaanalysis of studies in vascular surgery, exercise ECG stress testing had a positive likelihood ratio of 2.4 and negative likelihood ratio of 0.4 for predicting postoperative cardiac death or myocardial infarction. Conversely, ECG stress testing had diminished predictive performance (positive likelihood ratio 1.8 and negative likelihood ratio 0.8) in a cohort study of 200 patients undergoing mixed noncardiac surgery. Considerably more research has been published pertaining to the prognostic accuracy of preoperative stress testing based on myocardial perfusion imaging or echocardiography. In a meta-analysis in noncardiac surgery, stress echocardiography has a positive likelihood ratio of 4.1 and a negative likelihood ratio of 0.2 for predicting postoperative death or myocardial infarction. In the case of myocardial perfusion imaging, the positive likelihood ratio was 1.8 and negative likelihood ratio was 0.4. The prognostic value of myocardial perfusion imaging might be further improved by considering the extent of reversible defects. In another meta-analysis in vascular surgery, reversible defects on preoperative myocardial perfusion imaging were associated with significantly increased cardiac risk only when the extent of reversibility exceeded 20% of the myocardium. Notably, isolated fixed defects (i.e., no associated reversible defects) were not associated with elevated cardiac risk. While available evidence does suggest that stress echocardiography has better prognostic accuracy, these data should be interpreted cautiously since they are older, heterogeneous, and do not account for varying local expertise in stress testing modalities. In addition, it remains unclear as to whether cardiac stress tests provide incremental prognostic information in patients who have already been assessed using clinical risk factors.
Some patients with high-risk findings on initial cardiac stress testing may require subsequent evaluation of coronary anatomy using either noninvasive computed tomography coronary angiography (CTCA) or invasive coronary angiography. These tests can diagnose IHD, assess its severity, and help assess perioperative cardiac risk. Using contemporary imaging technology, CTCA has reasonably high accuracy for detecting clinically significant coronary artery stenosis. An initial retrospective cohort study found CTCA to improve prediction of cardiac complications after intermediate-risk noncardiac surgery, as compared to using the RCRI alone. Conversely, a larger multicenter prospective cohort study found that the addition of CTCA to the RCRI was five times more likely to overestimate risk in low-risk individuals than correctly identify a previously misclassified high-risk individual. Thus, CTCA does not appear to be an appropriate first-line test to supplement clinical risk stratification. Nonetheless, it may be a reasonable follow-up option in patients with high-risk cardiac stress test results.
Invasive coronary angiography is the gold standard for diagnosing IHD and may also be a follow-up option for patients with high-risk cardiac stress test results. Two Italian randomized trials in vascular surgery patients have suggested that routine preoperative invasive coronary angiography—followed by revascularization of any critical stenosis—reduces risks of postoperative myocardial ischemia, and long-term mortality. Although interesting, these findings still do not support a clinical shift to this invasive assessment strategy, largely because any patient-relevant benefits were seen over long-term follow-up, not the immediate postoperative period. Any merit for an invasive coronary artery assessment strategy is highly related to whether coronary revascularization before noncardiac surgery is beneficial. This issue remains controversial. The most relevant study is the Coronary Artery Revascularization Prophylaxis trial. In the multicenter randomized controlled trial of 510 vascular surgery patients with known significant IHD, preoperative revascularization using CABG or PCI with bare metal stents (BMSs) did not reduce the risk of postoperative myocardial infarction or long-term mortality. Notably, the trial excluded patients with left main coronary artery stenosis, which is the only subgroup where revascularization was associated with improved survival in a related cohort study. At present, both American and European guidelines only recommend consideration for revascularization in patients who meet usual nonoperative indications (e.g., left main coronary artery stenosis, triple-vessel coronary artery disease), while the CCS guidelines recommend against preoperative revascularization in any patient with stable IHD.
In general, randomized controlled trials have also not shown benefit from de novo medical therapy to decrease perioperative cardiac risk, including β-adrenergic blockers, α 2 -adrenergic agonists, and low-dose aspirin. Despite initial promising data on perioperative β-adrenergic blockade, these benefits were not replicated in larger multicenter randomized trials, such as the Perioperative Ischemic Evaluation Study-1 (POISE-1) trial. Current randomized trial evidence shows that perioperative β-adrenergic blockade reduces the risk of postoperative myocardial infarction, but at the cost of increased risks of acute stroke, hypotension, and death. Although it is possible that these risks are mitigated by starting β-adrenergic blockers several days before surgery, and titrating therapy to a reasonable target heart rate without precipitating hypotension, there are no compelling data showing the efficacy and safety of this approach. Caution should especially be exercised when using β-adrenergic blockers in individuals with known CVD because of the risks of perioperative stroke.
Conversely, most long-term cardiovascular medications in patients with IHD should be continued up to surgery, including β-adrenergic blockers, statins, and most other antihypertensive medications. Nonetheless, there are some exceptions. Since ACEI and ARB administration within 24 hours before surgery is associated with increased risks of hypotension and myocardial injury, it is reasonable to withhold these medications for 24 hours before surgery, provided that they are restarted postoperatively (see section on “Hypertension”). Despite theoretical benefits, randomized controlled trials to date have not shown benefits from routinely continuing aspirin before noncardiac surgery. For example, in the POISE-2 trial, continuing low-dose aspirin (100 mg/day) did not prevent cardiac complications but increased risks of major bleeding. The lack of clear benefit may be explained, in part, by acute thrombosis being a relatively infrequent contributor to perioperative myocardial infarction. Nonetheless, since only one third of participants in the POISE-2 trial had diagnosed vascular disease, it is possible that continuation of aspirin benefits some very high-risk subgroups. Consistent with this possibility, a relatively small post-hoc subgroup analysis from the POISE-2 trial found that perioperative aspirin reduced the risk of death or myocardial infarction in patients with prior PCI. Based on these data, a reasonable strategy is to only continue aspirin selectively in patients where the risk of cardiac events is felt to exceed the risk of major bleeding. Other medications that should generally be withdrawn before surgery include P2Y 12 inhibitors (e.g., clopidogrel, ticagrelor, prasugrel) and DOACs (see sections on “Atrial Fibrillation” and “Preoperative Antiplatelet Therapy” ).
Coronary Stents
Following PCI with stent implantation, patients require an initial period of dual antiplatelet therapy (DAPT) with aspirin and a P2Y 12 inhibitor (e.g., clopidogrel, ticagrelor, prasugrel), after which they can be transitioned to aspirin monotherapy. The purpose of DAPT is to prevent potentially catastrophic stent thrombosis during the vulnerable period preceding stent reendothelialization. Temporary preoperative discontinuation of DAPT during this vulnerable period predisposes patients to cardiovascular complications, especially given the prothrombotic state triggered by surgical stress. Based on emerging evidence and improved DES technology, recommendations pertaining to minimum duration of DAPT before elective noncardiac surgery continue to evolve—as reflected in updated 2016 guidelines published by the ACC/AHA. These guidelines recommend that elective noncardiac surgery should be delayed for 30 days or more after BMS implantation. In the case of DES, the ideal recommended minimum delay is 6 months, which is consistent with several cohort studies showing relatively low perioperative cardiac risk when elective noncardiac surgery was performed 6 months or more after DES implantation. Based on expert opinion, the guidelines also indicate that elective noncardiac surgery can sometimes be performed from 3 to 6 months after DES implantation (particularly with new generation stents), if the risk of stent thrombosis is judged to be less than the risk of further delaying surgery. When DAPT is temporarily interrupted before noncardiac surgery, the guidelines strongly recommend that aspirin be continued, and P2Y 12 inhibitor therapy be restarted as soon as possible after surgery.
Although not explicitly indicated in the 2016 ACC/AHA guidelines, factors other than stent type and time interval since PCI should also inform judgments on the safety of performing elective noncardiac surgery in patients with coronary stents. For example, a large retrospective cohort study of about 26,600 patients found that the risks of noncardiac surgery early after stent implantation were increased when PCI had been performed for acute myocardial infarction, as opposed to unstable angina or non-acute coronary syndrome indications. As a consequence, it may be particularly important to delay noncardiac surgery for 6 months when the indication for DES was treatment of an acute myocardial infarction.
During preoperative evaluation, the anesthesiologist should determine the presence, type (drug-eluting vs. bare-metal), location, and original indication of any coronary stent. Given the complex issues related to these patients, subsequent management should be performed in collaboration with a cardiologist and the responsible surgeon, especially for patients receiving DAPT. Whenever possible, surgical procedures should be performed following critical time windows (i.e., 30 days after BMS, or 3-6 months after DES), aspirin should be continued throughout the perioperative period, and any P2Y 12 inhibitor therapy should be restarted as soon as possible after surgery. The importance of continuing aspirin perioperatively is supported by the substudy of the POISE-2 randomized trial. In this subgroup analysis of 470 patients with prior PCI, aspirin reduced the risk of death or myocardial infarction (hazard ratio, 0.50; 95% confidence limits, 0.26-0.95) without any significantly increased bleeding risk. Unfractionated heparin and low-molecular-weight heparin (LMWH) should not be used to “bridge” patients who have been withdrawn from antiplatelet therapy, especially since heparin can paradoxically increase platelet aggregation. Following surgery, close monitoring for myocardial injury (i.e., serial troponin measurement) should be strongly considered, with any suspected stent thrombosis treated using PCI. High-risk patients are thus ideally best managed in facilities with immediate access to interventional cardiology.
Heart Failure
Heart failure has been defined as a clinical syndrome resulting from impaired diastolic filling or systolic ejection of the cardiac ventricles. Its major clinical manifestations are dyspnea, fatigue, and fluid retention. Although prevalence estimates are affected by differences in diagnostic criteria, recent estimates suggest that heart failure afflicts more than 6.5 million individuals in the United States, and more than 23 million worldwide. Heart failure is the sequelae of a broad array of underlying pathology, including ischemic heart disease (i.e., ischemic cardiomyopathy), hypertension, valvular heart disease, myocarditis, infiltrative disorder (e.g., sarcoidosis, amyloidosis), and peripartum cardiomyopathy. In addition, many affected individuals have no identifiable underlying cause (i.e., idiopathic dilated cardiomyopathy). Several approaches can be used to classify heart failure, including the presence versus absence of associated signs or symptoms (i.e., compensated vs. decompensated heart failure), and the extent of functional limitation. In heart failure patients, functional status is typically classified based on New York Heart Association (NYHA) categories:
- ▪
NYHA class I: no limitation of physical activity; ordinary activity not a cause of fatigue, palpitations, or syncope
- ▪
NYHA class II: slight limitation of physical activity; ordinary activity resulting in fatigue, palpitations, or syncope
- ▪
NYHA class III: marked limitation of physical activity; less than ordinary activity resulting in fatigue, palpitations, or syncope; comfort at rest
- ▪
NYHA class IV: inability to do any physical activity without discomfort; symptoms at rest
Heart failure can also be classified based on the severity of ventricular systolic dysfunction, namely as heart failure with reduced ejection fraction (HFrEF) versus heart failure with preserved ejection fraction (HFpEF). Individuals with HFpEF (or diastolic heart failure) have normal left ventricular ejection fraction (≥50%), normal left ventricular end-diastolic volume, and abnormal diastolic function. By comparison, HFrEF (or systolic heart failure) is characterized by more significant left ventricular systolic dysfunction (i.e., ejection fraction ≤ 40%). Individuals with borderline left ventricular systolic function (i.e., ejection fraction 41%-49%) are classified as borderline HFpEF. They tend to have characteristics and outcomes similar to patients with HFpEF. Among patients with heart failure, about half have HFpEF. Although they have a high mortality risk (10%-20% at 1-year), patients with HFpEF have lower adjusted risks of death (adjusted hazard ratio 0.68) over long-term follow-up than patients with HFrEF. Most medical therapy for improving morbidity and mortality in heart failure (i.e., ACEI, ARB, aldosterone antagonist, β-adrenergic blocker, ivabradine) has only demonstrated efficacy in HFrEF. In contrast, medical therapy for HFpEF is largely aimed at symptoms and underlying conditions (e.g., hypertension). Other heart failure-related treatments include diuretics (for volume overload), anticoagulants (for atrial fibrillation or left ventricular thrombus), implantable cardioverter-defibrillators (ICDs), or cardiac resynchronization therapy (CRT).
In the perioperative setting, heart failure is a recognized risk factor for mortality and morbidity after major surgery. Symptomatic heart failure has been consistently identified as a risk factor for adverse perioperative outcomes in multiple studies. For example, in a retrospective cohort study of about 159,000 Medicare beneficiaries in the United States, heart failure was associated with significantly higher risks of 30-day mortality (adjusted hazard ratio 1.63) after noncardiac surgery. Similarly, a more recent matched cohort study using the NSQIP registry showed new or worsened heart failure within 30 days before surgery to be associated with increased risks of 30-day mortality (adjusted relative risk 2.08) or major morbidity (adjusted relative risk 1.54). Symptomatic heart failure is also a component of the Revised Cardiac Index, which is commonly used for estimating perioperative cardiac risk cardiovascular complications. Among patients with heart failure, perioperative risk may be higher in patients with HFrEF versus HFpEF. Specifically, in a cohort study of 174 heart failure patients undergoing noncardiac surgery, an ejection fraction less than 30% was associated with much higher adjusted risks (adjusted odds ratio 4.88) of postoperative death, myocardial infarction, or heart failure exacerbation. Nonetheless, HFpEF may still be prognostically important in the perioperative setting, as evidenced by a meta-analysis that found diastolic dysfunction to be associated with a doubling (pooled adjusted odds ratio 2.03) in the risk of postoperative adverse cardiac events. Although symptomatic HF is a clear indicator of increased perioperative risk, the prognostic importance of asymptomatic systolic dysfunction is less clear. For example, in a cohort study of 339 individuals undergoing noncardiac surgery, a reduced ejection fraction was associated with increased cardiac morbidity, but this information did not improve risk prediction beyond that achieved with clinical risk factors. Similarly, in another cohort study of 570 individuals undergoing noncardiac surgery, a reduced ejection only had prognostic importance within the subgroup with RCRI scores of 2 or greater. Consistent with these data, the current ACC/AHA guidelines discourage routine preoperative assessment of ventricular function ( Box 31.3 ).
Class IIa (Reasonable to Perform)
- ▪
It is reasonable for patients with dyspnea of unknown origin to undergo preoperative evaluation of LV function.
- ▪
It is reasonable for patients with heart failure with worsening dyspnea or other change in clinical status to undergo preoperative evaluation of LV function.
Class IIb (May Be Considered)
- ▪
Reassessment of LV function in clinically stable patients with previously documented LV dysfunction may be considered if there has been no assessment within a year.
Class III (Should Not Be Performed Since It Is Not Helpful)
- ▪
Routine preoperative evaluation of LV function is not recommended.
LV, Left ventricle.
The clinical stability of heart failure symptoms prior to surgery is another important determinant of perioperative risk, as evidenced by a single-center retrospective cohort study from the United States. After examination at a hospitalist-run preoperative evaluation clinic, clinically stable heart failure patients had relatively low risks of 30-day mortality (1.3%) after noncardiac surgery, albeit with longer hospital length of stay and higher readmission rates relative to matched controls. Consistent with these data, the ESC/ESA guidelines recommend that elective intermediate-risk and high-risk noncardiac procedures be deferred for at least 3 months after initiation of medical therapy in patients with newly diagnosed heart failure.
The preoperative history pertaining to heart failure should clarify its type, etiology, severity, stability (including prior exacerbations), recent investigations (e.g., echocardiograms), and current therapy (medical and device-based). The anesthesiologist should inquire about recent weight gain, fatigue, shortness of breath, orthopnea, paroxysmal nocturnal dyspnea, nocturnal cough, peripheral edema, hospitalizations, and recent changes in medical management. The patient’s functional status should be classified according to the NYHA categories. It is especially important to determine if the signs and symptoms of heart failure are in their usual stable state (i.e., compensated heart failure) or have recently significantly worsened (i.e., decompensated heart failure). Decompensated heart failure is a very high-risk condition that warrants postponement of surgery for all except lifesaving emergency procedures. No consensus exists on how long nonemergent surgery should be deferred after resolution of acute decompensated heart failure, although a reasonable approach is to delay elective procedures (including most time-sensitive procedures) for 1 month, and urgent procedures for 24 hours.
On physical examination, the findings of heart failure may be subtle. Furthermore, they can vary between HFrEF versus HFpEF, and between compensated versus decompensated states. More useful signs for determining heart failure include a third heart sound, jugular venous distension, pulmonary rales, and lower extremity edema. A chest radiograph may provide further diagnostic guidance, especially in dyspneic patients, with pulmonary vascular redistribution and interstitial edema useful findings for supporting the presence of heart failure. Natriuretic peptide measurement can further clarify whether a patient has heart failure. Both BNP and NT pro-BNP have excellent diagnostic performance, especially for ruling out heart failure, in the ambulatory setting (pooled negative likelihood ratio values 0.29-0.38 at manufacturers’ recommended thresholds) and emergency department setting (pooled negative likelihood ratio values 0.08-0.13 at manufacturers’ recommended thresholds). Preoperative natriuretic peptide concentrations also provide other potentially important information. Both BNP and NT pro-BNP concentrations are markers of perioperative cardiac risk (see section on “Ischemic Heart Disease”). In addition, for heart failure patients who have undergone prior natriuretic peptide measurement, preoperative BNP or NT pro-BNP testing provides insights into whether patients remained in their usual stable clinical state. Consistent with these data, the ESC/ESA guidelines recommend preoperative natriuretic peptide measurement in patients who have established or suspected heart failure, and are undergoing noncardiac intermediate or high-risk noncardiac surgery. While routine preoperative echocardiography (or other noninvasive ventricular function measurement) is not useful, such specialized testing is helpful for assessment of dyspnea of unknown origin or recent altered clinical status in an individual with known heart failure. Furthermore, both American and European guidelines are supportive of selective preoperative echocardiography in clinically stable patients with known ventricular dysfunction, especially in the absence of testing in the previous year (see Box 31.3 ). Other tests for patients with heart failure include ECGs and blood sampling to measure electrolyte and creatinine concentration. Digoxin levels are not routinely determined unless toxicity, undertreatment, or noncompliance is suspected.
Consideration should be given for collaborative perioperative management with a cardiologist or heart failure specialist of severely affected heart failure patients (i.e., NYHA III or IV; decompensated heart failure) who will undergo intermediate-risk or high-risk procedures. Most medical therapy, including β-adrenergic blockers, hydralazine, nitrates, and digoxin, should be continued preoperatively. Loop diuretics (e.g., furosemide) can be continued on the day of surgery for most procedures since this strategy does not increase risks of intraoperative hypotension or adverse cardiac events. The exception is lengthy high-risk procedures with projected significant blood loss or fluid requirements, in which potent diuretics should be held on the morning of surgery. Since ACEI and ARB administration within 24 hours before surgery is associated with increased risks of intraoperative hypotension, and postoperative myocardial injury, it is reasonable to withhold these medications for 24 hours before surgery, provided that they are restarted postoperatively once patients are hemodynamically stable (see section on “Hypertension”). Patients on anticoagulant therapy will likely need these medications temporarily discontinued before surgery (see later section on “Atrial Fibrillation”). In addition, patients with pacemakers, ICDs, and CRT devices have their own special perioperative considerations (see later section on “Cardiovascular Implantable Electronic Devices”).
Murmurs and Valvular Abnormalities
When a cardiac murmur is identified during preoperative assessment, the subsequent goals are to identify any associated cardiovascular symptoms (e.g., dyspnea, chest discomfort, orthopnea, fatigue, syncope), determine the underlying cause of the murmur, and distinguish clinically significant murmurs from unimportant ones. For example, benign functional murmurs occur with turbulent flow across the aortic or pulmonic outflow tracts during high-flow states such as hyperthyroidism, pregnancy, or anemia. Murmurs can be graded according to loudness ( Table 31.7 ), however, the loudness of a murmur does not necessarily indicate the severity of a lesion. In contrast, the location of the murmur, and changes in intensity associated with maneuvers can be informative ( Table 31.8 ). A Valsalva maneuver decreases right- and left-heart filling, thereby reducing the intensity of most murmurs except those of mitral valve prolapse and hypertrophic cardiomyopathy. Standing also decreases preload, and thereby increases the intensity of murmurs of mitral valve prolapse and hypertrophic cardiomyopathy. Conversely, squatting increases venous return and afterload, thereby increasing most murmurs except those of mitral valve prolapse and hypertrophic cardiomyopathy. Having the patient repeatedly perform a hand grip increases heart rate and arterial blood pressure, thereby augmenting murmurs of mitral regurgitation and aortic insufficiency; conversely, this maneuver decreases murmurs of aortic stenosis and hypertrophic cardiomyopathy. The likelihood of a murmur being pathologic increases in the presence of increased age, cardiovascular risk factors, other abnormal heart sounds, cardiomegaly, abnormal ECG, evidence of excessive intravascular volume, and a history of rheumatic fever, pulmonary disease, or anorectic drug use. Diastolic or continuous murmurs are almost always pathologic and require further evaluation. Nonetheless, it is typically not easy to distinguish benign from pathologic murmurs based on history and physical examination alone. Thus, cardiovascular medicine guidelines now recommend transthoracic echocardiography for the initial evaluation of any patient with suspected valvular heart disease. Additionally, perioperative guidelines recommend preoperative echocardiography for any patient who has clinically suspected moderate or severe valvular stenosis or regurgitation, and no echocardiogram within the previous year. For patients with known valvular heart disease, repeat echocardiography is recommended if there has been a significant change in clinical status or physical examination since the last test.
Grade | Description |
---|---|
I | Faintest that can be heard with difficulty |
II | Faint but easily heard |
III | Moderately loud without a thrill |
IV | Loud with a palpable thrill |
V | Very loud but still need stethoscope (thrill present) |
VI | Heard without a stethoscope |
Lesion | Location | Timing | Description |
---|---|---|---|
Aortic Stenosis | Second parasternal interspace | Midsystolic | Crescendo-decrescendo, radiates to the carotids; with or without S 3 , S 4 ; Valsalva maneuver and sustained hand grip exercise decrease intensity |
Aortic Insufficiency | Third and fourth parasternal interspaces | Holodiastolic | Decrescendo, blowing, high pitched, radiates to the carotids; Austin-Flint rumble at the apex; squatting, hand grip exercise, and leaning forward increase intensity |
Mitral Stenosis | Apex | Middiastolic | Opening snap; low-pitched rumble radiates to the axilla; squatting and hand grip exercise increase intensity |
Mitral Regurgitation | Apex | Holosystolic | High pitched, blowing, radiates to the axilla; loud S 3; standing decreases intensity; squatting and hand grip exercise increase intensity |
Mitral Valve Prolapse | Apex | Late systolic | Crescendo, midsystolic click; Valsalva maneuver and standing increase intensity; squatting decreases intensity |
Hypertrophic Cardiomyopathy | Apex, lower left sternal border | Midsystolic | S 4 , Single S 2 ; Valsalva maneuver and standing increase intensity; squatting, passive leg raising, and hand grip exercise decrease intensity |
Aortic Stenosis
Aortic valve stenosis is the leading cause of left ventricular outflow obstruction in adults. In high-income countries, aortic stenosis principally occurs because of the progressive calcific disease of a native trileaflet valve or a congenitally bicuspid valve. Stenosis of bicuspid valves typically occurs when individuals are in their fourth and fifth decades of life, whereas stenosis of native trileaflet valves occurs once individuals are age 60 years old or older. Aortic stenosis severity is classified based on the valve area and mean transvalvular pressure gradient ( Table 31.9 ). A limitation of using the pressure gradient alone to assess severity is that the gradient may decrease if the left ventricle systolic function begins to decrease. In patients with known aortic stenosis, serial echocardiography is recommended every 6 to 12 months for patients with severe disease, every 1 to 2 years for moderate disease, and every 3 to 5 years for mild disease. After a prolonged asymptomatic phase, symptoms may develop in affected patients once stenosis becomes severe. The cardinal symptoms of severe aortic stenosis are angina, heart failure, and syncope, but patients are much more likely to complain of exertional dyspnea and decreased exercise tolerance.
Grade | Transvalvular Jet Velocity (m/s) | Mean Pressure Gradient (mm Hg) | Valve Area (cm 2 ) |
---|---|---|---|
Mild | 2.0–2.9 | <20 | ≥1.5 |
Moderate | 3–3.9 | 20–39 | 1.0–1.5 |
Severe | ≥4 | ≥40 | <1.0 |
Aortic stenosis causes a systolic ejection murmur (see Table 31.8 ), best heard in the right upper sternal border and often radiating to the neck. A similar murmur occurs with aortic sclerosis , which is defined as thickening of the aortic valve without associated stenosis. Aortic sclerosis is present in 25% of individuals age 65 years or older, and 50% of individuals age 80 years or older. Aortic sclerosis is associated with an increased risk of cardiovascular events, and a 2% annual risk of progression to aortic stenosis. The radiating pattern of the systolic ejection murmur can help rule out aortic stenosis, with the absence of radiation to the right clavicle being associated with a negative likelihood ratio of 0.1 for aortic stenosis. In addition to a systolic ejection murmur, aortic stenosis is associated with a delayed carotid upstroke and paradoxically split second heart sound. Any patient with a previously undiagnosed suspicious systolic murmur should have an echocardiogram, especially since noncardiologists have difficulty in distinguishing murmurs of aortic stenosis from those of aortic sclerosis. Typical ECG abnormalities associated with aortic stenosis include LVH (often with a strain pattern), left axis deviation, and LBBB.
Moderate to severe aortic stenosis is associated with increased risk of perioperative cardiovascular complications. Nonetheless, contemporary studies suggest that noncardiac surgery can be performed with acceptable mortality risks in individuals with asymptomatic severe aortic stenosis Thus, guidelines support proceeding with major elective noncardiac surgery in patients with asymptomatic severe aortic stenosis, provided that appropriate intraoperative and postoperative hemodynamic monitoring is available. Conversely, for patients with symptomatic severe aortic stenosis, aortic valve replacement should be considered before their planned noncardiac surgery. If a symptomatic patient is deemed high-risk or ineligible for surgical aortic valve replacement, alternative intervention options include transcatheter aortic valve replacement (TAVR) or percutaneous aortic balloon dilation. Multidisciplinary collaboration with a cardiologist is invaluable for the perioperative management of these high-risk patients.
Patients with moderate to severe aortic stenosis also have an increased risk of bleeding from an acquired von Willebrand syndrome, which occurs in 67% to 92% of patients with severe stenosis. The underlying pathophysiology is mechanical disruption of von Willebrand multimers during turbulent blood flow through the narrowed valve. Of note, prophylaxis for infective endocarditis is not recommended.
Aortic Insufficiency
Aortic valve insufficiency occurs with valvular leaflet disease, aortic root dilation, or both. Causes of valvular disease include rheumatic heart disease, bicuspid valves, collagen vascular disease, and endocarditis. With respect to aortic root dilation, causes include ankylosing spondylitis, osteogenesis imperfecta, syphilis, hypertension, age-related degeneration, Marfan syndrome, and collagen vascular diseases. These causes generally lead to progressive chronic aortic insufficiency that remains asymptomatic for decades. In contrast, acute aortic insufficiency can result from trauma, infections, or aortic dissection; this is an emergent condition that results in cardiogenic shock.
On auscultation, aortic insufficiency is associated with a diastolic murmur (see Table 31.8 ), the intensity of which does not correlate well with the severity of regurgitation. Patients typically have a widened pulse pressure, which manifests as Corrigan or water-hammer pulses (bounding carotid pulse with a rapid downstroke), de Musset sign (head bob with each heart beat), Duroziez sign (systolic and diastolic bruit heard over the femoral artery when it is partially compressed), and Quincke pulses (capillary pulsations in the fingertips or lips). Although auscultation by a cardiologist can help rule in or rule out aortic regurgitation (based on the presence or absence of an early diastolic murmur), the accuracy of auscultation by noncardiologists is uncertain. Thus, an echocardiogram is helpful for any patient suspected of having a diastolic murmur.
Limited prior research suggests that patients with moderate-to-severe aortic insufficiency experience increased risks of perioperative mortality and morbidity, especially in the presence of impaired left ventricular function (ejection fraction < 55%) or renal insufficiency. Nonetheless, expert consensus from current guidelines is supportive of patients with asymptomatic severe aortic insufficiency to proceed with major noncardiac surgery accompanied by careful perioperative management, including hemodynamic monitoring, afterload control, and fluid balance. Prophylaxis for infective endocarditis is not recommended.
Mitral Stenosis
Mitral stenosis is much rarer than aortic stenosis, and almost always related to rheumatic heart disease. Other less common causes include mitral annular calcification and radiation-associated valve disease. The normal mitral valve has a cross-sectional area of 4 to 6 cm 2 . Mitral stenosis involves progressive reduction of this area, with shortness of breath with exertion occurring when the area falls below 2.5 cm 2 , and symptoms at rest occurring once the area falls below 1.5 cm 2 . Severe mitral stenosis is defined by a valve area less than 1 cm 2 and is typically associated with a pulmonary artery systolic pressure > 50 mm Hg, and a resting mean transvalvular gradient ≥ 10 mm Hg.
When mitral stenosis becomes symptomatic, patients experience dyspnea, fatigue, orthopnea, pulmonary edema, and hemoptysis because of increased left atrial pressures and decreased cardiac output. Atrial fibrillation can also develop, in turn causing heart failure in the short term and thrombosis in the long term. Patients with atrial fibrillation require long-term anticoagulation, with recent guidelines recommending vitamin K antagonist therapy (e.g., warfarin) as opposed to DOACs. Pulmonary hypertension (see later section on “Pulmonary Hypertension”) and right-sided heart failure can also occur in patients with significant stenosis. The physical examination should evaluate for rales and signs of right-sided heart failure such as jugular venous distention, peripheral edema, hepatomegaly, right ventricular heave, and ascites. On auscultation, mitral stenosis is associated with a diastolic murmur that should be evaluated with echocardiography.
Medical management includes β-adrenergic blockers for controlling heart rate, antiarrhythmic agents for preventing or controlling atrial fibrillation, and anticoagulants in patients with atrial fibrillation. Both β-adrenergic blockers and antiarrhythmic agents should be continued preoperatively, while anticoagulation should be managed in conjunction with the treating cardiologist and surgeon. Prophylaxis for infective endocarditis is not recommended. If a patient with mitral stenosis meets guideline-based indications for valvular intervention (e.g., percutaneous mitral balloon commissurotomy), consideration should be given to performing this intervention before major elective noncardiac surgery. If an asymptomatic patient with severe mitral stenosis has valve morphology that is not favorable for a percutaneous intervention, it is still reasonable to proceed with major elective noncardiac surgery provided that appropriate intraoperative and postoperative hemodynamic monitoring is used.
Mitral Regurgitation
Acute mitral regurgitation can occur in the setting of myocardial infarction, trauma, or infective endocarditis. Chronic mitral regurgitation is typically associated with degenerative mitral valve disease (including mitral valve prolapse), rheumatic heart disease, ischemic heart disease, and cardiomyopathies. Chronic mitral regurgitation progresses very gradually. Symptoms develop relatively late, and typically only after onset of left ventricular dysfunction. Associated symptoms are initially vague, but progress to fatigue, dyspnea, and atrial fibrillation with disease progression.
On auscultation, patients with mitral regurgitation usually have a holosystolic murmur heard best at the cardiac apex (see Table 31.8 ). The murmur grade is somewhat correlated with the severity of mitral regurgitation in primary mitral valve disease (e.g., degenerative mitral valve disease), but not in secondary functional regurgitation (e.g., ischemic heart disease, cardiomyopathies). Although auscultation by a cardiologist can help determine mitral regurgitation (based on the presence or absence of a late systolic or holosystolic murmur in the mid-left thorax), the accuracy of auscultation by noncardiologists is uncertain. Thus, an echocardiogram is helpful for any patient with a suspicious systolic murmur. Chronic mitral regurgitation is generally well tolerated perioperatively, unless other valvular lesions (e.g., mitral stenosis) or left ventricular dysfunction coexist. Consistent with these observations, limited prior research suggests that patients with mitral regurgitation have increased risks of cardiovascular complications, but not mortality. Current guidelines suggest that patients with asymptomatic severe mitral regurgitation can proceed to major elective noncardiac surgery provided that appropriate intraoperative and postoperative hemodynamic monitoring is available. Prophylaxis for infective endocarditis is no longer recommended.
Mitral Valve Prolapse
Mitral valve prolapse is characterized by the systolic billowing of an abnormally thickened mitral valve leaflet into the left atrium, with or without coexistent mitral regurgitation. Diagnostic criteria for mitral valve prolapse have evolved to the current definition based on echocardiography alone, namely ≥ 2 mm billowing of any portion of the mitral leaflets above the annular plane in the long axis view. It is the common cause of isolated mitral regurgitation requiring surgical repair in high-income countries. Nonetheless, only 4% of patients with mitral valve prolapse have severe valvular regurgitation, with most affected individuals having mild, trace, or no mitral regurgitation. The condition may be primary (i.e., myxomatous degeneration) or secondary (e.g., associated with Marfan syndrome, Ehlers-Danlos syndrome, and osteogenesis imperfecta). Nonspecific symptoms (e.g., atypical chest pain, palpitations, dyspnea, exercise intolerance, dizziness) have been attributed, albeit unreliably, to mitral valve prolapse—in what has been termed as the “mitral valve prolapse syndrome.”
On auscultation, these patients may have a systolic click and a mid-systolic murmur at the cardiac apex (that intensifies with a Valsalva maneuver). Auscultation by a cardiologist can help diagnose mitral valve prolapse (based on the presence or absence of a systolic click and murmur); however, the accuracy of auscultation by noncardiologists is uncertain. From the perioperative perspective, the key issue is to differentiate patients with clinically significant mitral valve regurgitation from those with an incidental finding of prolapse that does not warrant further evaluation. Thus, echocardiography may be helpful to further investigate murmurs suspected of representing significant mitral regurgitation. Prophylaxis for infective endocarditis is no longer recommended.
Tricuspid Regurgitation
Tricuspid regurgitation is a common valvular abnormality, with 70% of normal adults exhibiting a small degree of tricuspid regurgitation on echocardiography. Since it is usually asymptomatic and not easily audible on physical examination, tricuspid regurgitation is most commonly noted as an incidental echocardiographic finding. Significant tricuspid regurgitation is most commonly caused by dilatation of the right ventricle and tricuspid annulus (i.e., secondary disease). Causes of secondary tricuspid regurgitation include left-sided heart failure, mitral valve disease (stenosis or regurgitation), primary pulmonary disease (e.g., pulmonary hypertension), left-to-right intracardiac shunts (e.g., atrial septal defect, ventricular septal defect), pulmonary artery stenosis, and right ventricle disease (e.g., arrhythmogenic right ventricular dysplasia). Tricuspid regurgitation is less commonly caused by processes directly affecting the tricuspid valve (i.e., primary disease), such as Ebstein anomaly, infective endocarditis (typically in intravenous drug users), rheumatic heart disease, carcinoid syndrome, connective tissue disorders (e.g., Marfan syndrome), myxomatous degeneration, or direct injury (e.g., permanent pacemaker or ICD lead). Certain drugs—namely anorectics (fenfluramine, phentermine) and pergolide (dopamine agonist)—have also been associated with primary tricuspid regurgitation.
Patients with mild or moderate tricuspid regurgitation are generally asymptomatic. Individuals with severe regurgitation may report pulsations in the neck (related to distended jugular veins), as well as symptoms of right-sided heart failure (e.g., ascites, peripheral edema) and underlying conditions (e.g., pulmonary hypertension). Relevant findings on physical examination include distended jugular veins, hepatomegaly, ascites, dependent edema, and a right ventricular heave on chest palpation. On auscultation, tricuspid regurgitation causes a holosystolic murmur that is heard best at the midsternal border or subxiphoid area. Nonetheless, the murmur is often absent or very soft, even with severe regurgitation. The intensity of the murmur can be increased using maneuvers that increase venous return (e.g., inspiration, abdominal pressure). Although auscultation by a cardiologist can help determine moderate-to-severe tricuspid regurgitation (based on the presence or absence of increased murmur intensity with inspiration or abdominal pressure), the accuracy of auscultation by noncardiologists is uncertain. Thus, echocardiography may be helpful in a patient with a suspicious murmur, especially within the context of signs or symptoms of right-sided heart failure. The preoperative management of patients with severe tricuspid regurgitation should be guided by the presence of any underlying conditions, right-sided heart failure, and known or suspected pulmonary hypertension. Where indicated, perioperative management should be conducted collaboratively with a heart failure cardiologist or pulmonary hypertension specialist. Prophylaxis for infective endocarditis is no longer recommended.
Hypertrophic Cardiomyopathy
Hypertrophic cardiomyopathy is a genetic disorder of the myocardium, which can result in dynamic left ventricular outflow tract obstruction, myocardial ischemia, diastolic dysfunction, and mitral regurgitation (related to systolic anterior motion of the mitral valve leaflets). Most affected individuals have a relatively normal lifespan; however others are at risk for progressive heart failure, sudden cardiac death, and atrial fibrillation. Most patients with hypertrophic cardiomyopathy are asymptomatic. When symptoms are present, they are quite variable, and not well correlated with the extent of LVH or outflow tract obstruction. Typical associated symptoms include fatigue, exertional dyspnea, atypical or angina chest pain, exertional presyncope or syncope, and palpitations. Symptomatic patients may be prescribed long-term medical therapy with negative inotropic agents (i.e., β-blockers, verapamil, disopyramide). Other symptomatic individuals with severe left ventricular outflow tract obstruction may require intervention with either surgical myectomy or alcohol septal ablation. Some patients may also undergo ICD insertion if they are deemed high-risk for sudden cardiac death.
Although the physical examination in a patient with hypertrophic cardiomyopathy may be normal, the classic finding on auscultation (see Table 31.8 ) is a mid-systolic murmur that increases with maneuvers to decrease ventricular size (e.g., Valsalva maneuver) and decreases with maneuvers to increase ventricular size (e.g., passive leg raise). Auscultation by a cardiologist can help rule in or rule out hypertrophic cardiomyopathy (based on decreased murmur intensity with passive leg elevation or increased murmur intensity with shifts from a squatting to standing position), however, the accuracy of auscultation by noncardiologists is uncertain. Accordingly, an echocardiogram should be obtained if a patient has a personal (or family) history of exertional syncope or cardiac arrest; a suspicious murmur is found on auscultation; or concerning ECG findings (i.e., LVH, ST-segment abnormalities, T-wave abnormalities) are observed in an otherwise healthy nonhypertensive patient. In general, patients with hypertrophic cardiomyopathy can safely undergo most low-risk and intermediate-risk noncardiac surgical procedures, especially with close hemodynamic monitoring and management. Medical therapy (e.g., β-adrenergic blockers, verapamil) should be continued perioperatively, but prophylaxis for infective endocarditis is no longer recommended. Appropriate perioperative management should be instituted for any patient who has an associated ICD.
Prosthetic Heart Valves
In patients with prosthetic heart valves, the preoperative evaluation should determine the underlying indication that led to valve replacement; type, age, and current status of the valve prosthesis; need for long-term anticoagulation entailed by the valve prosthesis; and planned perioperative anticoagulation management plan. The anesthesiologist should review the most recent echocardiogram, and order a repeat echocardiogram if there are any signs or symptoms suggestive of valve dysfunction (e.g., new onset heart failure). In addition, a recent complete blood count may be helpful since these patients may have a valve-related hemolytic anemia.
Anticoagulation with a vitamin K antagonist (e.g., warfarin) is required for 3 to 6 months after open surgical bioprosthetic valve implantation, after which patients can be transitioned to aspirin therapy alone (75-100 mg daily). Life-long aspirin therapy is recommended for patients who have undergone TAVR, as well as clopidogrel (75 mg daily) for the first 6 months in all patients and vitamin K antagonists for the first 3 months in select individuals. Conversely, patients with mechanical prosthetic valves require life-long therapy with both aspirin and vitamin K antagonists. Importantly, DOACs—such as dabigatran, rivaroxaban, edoxaban, or apixaban—should not be used for anticoagulation therapy in patients with mechanical heart valves. In general, decisions about temporary preoperative discontinuation of anticoagulants, duration of anticoagulant discontinuation, need for “bridging” therapy with a shorter-acting drug, and type of bridging agent (intravenous heparin or LMWH) should be made in conjunction with the treating cardiologist and surgeon. Current guidelines make recommendations for bridging therapy largely based on the location of the mechanical heart valve and nature of planned surgery ( Box 31.4 ). Prophylaxis for infective endocarditis is recommended for specific procedures (see section on “Infective Endocarditis Prophylaxis”).
Class I (Recommended)
- ▪
Continuation of vitamin K antagonist anticoagulation with a therapeutic INR is recommended in patients with mechanical heart valves undergoing minor procedures (e.g., dental extractions, cataract removal) where bleeding is easily controlled.
- ▪
Temporary interruption of vitamin K antagonist anticoagulation, without bridging agents while the INR is subtherapeutic, is recommended in patients with a bileaflet mechanical AVR and no other risk factors ∗
∗ Risk factors include atrial fibrillation, previous thromboembolism, hypercoagulable condition, older-generation ball-cage or tilting disc mechanical valve, left ventricular systolic dysfunction, and ≥ 2 mechanical valves.
for thrombosis who are undergoing invasive or surgical procedures.
Class IIa (Is Reasonable)
- ▪
Bridging anticoagulation therapy during the time interval when the INR is subtherapeutic preoperatively is reasonable on an individualized basis—with the risks of bleeding weighed against the benefits of thromboembolism prevention—for patients who are undergoing invasive or surgical procedures with a (i) mechanical AVR and any thromboembolic risk factor, (ii) older-generation mechanical AVR, or (iii) mechanical MVR.
AVR, Aortic valve replacement; INR, international normalized ratio; MVR, mitral valve replacement.
Infective Endocarditis Prophylaxis
Patients at risk for infective endocarditis (e.g., valve replacement, complex congenital heart disease, previous endocarditis) and scheduled for procedures with the potential for transient bacteremia must be identified preoperatively. Current guidelines have dramatically scaled back the indications for prophylaxis. For example, current ACC/AHA guidelines recommended prophylaxis only for patients who are both at increased risk of developing infective endocarditis and also experiencing adverse outcomes from endocarditis ( Box 31.5 ). These target patient subgroups are also with those identified in the most recent ESC guidelines. Prophylaxis is required when eligible patients undergo dental procedures that involve manipulation of gingival tissue, manipulation of the periapical region of teeth, or perforation of the oral mucosa. Prophylaxis is not required when these same individuals undergo nondental procedures (e.g., transesophageal echocardiography, gastroscopy, colonoscopy, cystoscopy, dermatological procedures), unless there is active infection at the procedure site.
Previous infective endocarditis
Prosthetic cardiac valves, including transcatheter-implanted prostheses, and homografts
Prosthetic material used for cardiac valve repair, such as annuloplasty rings and chords
Unrepaired cyanotic congenital heart disease, including palliative shunts and conduits
Repaired congenital heart disease, with residual shunts or valvular regurgitation at the site of or adjacent to the site of a prosthetic patch or prosthetic device
Cardiac transplant with valve regurgitation due to a structurally abnormal valve
Prophylaxis is reasonable before dental procedures that involve manipulation of gingival tissue, manipulation of the periapical region of teeth, or perforation of the oral mucosa.
Rhythm Disturbances on the Preoperative Electrocardiogram
Arrhythmias and conduction disturbances are common during the perioperative period. Supraventricular and ventricular arrhythmias are associated with increased perioperative risk, both because of the arrhythmias themselves and because they are markers for cardiopulmonary disease. Uncontrolled atrial fibrillation (i.e., causing symptoms or hemodynamic compromise) and high-risk ventricular tachyarrhythmias (see section on “Ventricular Arrhythmias”) are high-risk conditions that warrant postponement of elective surgery until evaluation and stabilization are complete. Rapid atrial fibrillation (rates > 100 beats/min), symptomatic bradycardia, or high-grade heart block (e.g., third-degree heart block) also warrants postponement of elective procedures to facilitate evaluation, stabilization, and possible cardiology evaluation.
First-degree atrioventricular (AV) block is defined as a PR interval exceeding 0.20 ms with an associated heart rate of 50 to 100 beats/min; it is considered generally benign. Second-degree heart block is defined by a PR interval exceeding 0.20 ms and the presence of some blocked atrial beats (resulting in a dropped or missing QRS complex after a P wave). There are two types of second-degree block. Mobitz type I block (also called Wenckebach block) is characterized by progressive lengthening of the PR interval until the dropped beat occurs. It is a more benign block that is related to AV nodal delay, easily responsive to atropine, and unlikely to progress to complete heart block. Mobitz type II block is characterized by a fixed and prolonged PR interval that does not change before the dropped QRS complex. It is related to an infranodal block, capable of progressing to complete heart block, and generally treated with a pacemaker (unless it is due to a reversible cause such as ischemia or drugs). Third-degree AV block or complete heart block, which is characterized by complete dissociation between the atrial and ventricular beats, requires pacemaker placement unless a reversible source is identified. A prolonged QT interval should prompt an evaluation of electrolytes (including magnesium and calcium), and search for any potentiating drugs. Syncope, presyncope, or a family history of sudden death in a patient with a prolonged QT interval mandates a cardiology consultation (see section on “Long QT Syndrome”).
Three factors determine the need for permanent pacemaker treatment for an arrhythmia, namely whether the arrhythmia is associated with symptoms, where the conduction abnormality is located, and whether a reversible cause can be identified. Indications for perioperative pacemaker placement are the same as those in nonsurgical patients (common indications listed in Box 31.6 ). In general, pacemaker placement is required for symptomatic bradycardia or conduction delays that lead to syncope or presyncope. Conduction disease below the AV node (i.e., in the His-Purkinje system) is also generally less stable and likely benefits from permanent pacemaker placement. The presence of such conduction disease is suggested by a normal or minimally prolonged PR interval, Mobitz type II block, and QRS complex abnormalities (BBB, fascicular block, or both).
Class I Indications
- ▪
Sinus bradycardia with symptoms due to the bradycardia (typically seen with heart rates less than 40 bpm or with frequent sinus pauses)
- ▪
Symptomatic chronotropic incompetence (i.e., impaired heart rate response to exercise)
- ▪
Third-degree AV block
- ▪
Advanced second-degree AV block (block of ≥2 consecutive P waves)
- ▪
Symptomatic Mobitz I or II second-degree AV block
- ▪
Mobitz II second-degree AV block with a widened QRS or chronic bifascicular block, regardless of symptoms
- ▪
Exercise-induced second- or third-degree AV block
Class II Indications
- ▪
Sinus bradycardia (heart rate < 40 bpm) with symptoms suggestive (but not definitively so) of bradycardia
- ▪
Sinus node dysfunction with a history of unexplained syncope
- ▪
Chronic heart rates < 40 bpm in an awake but minimally symptomatic patient
- ▪
Asymptomatic Mobitz II second-degree AV block with a narrow QRS interval
- ▪
Bifascicular or trifascicular block associated with syncope possibly related to intermittent third-degree heart block
- ▪
First-degree AV block with a very long PR interval (which effectively leads to AV dissociation and hemodynamic compromise)
AV, Atrioventricular; bpm, beats per minute.
Class I Indications: Permanent pacing is definitely beneficial, useful, and effective.
Class II Indications: Permanent pacing may be indicated but there is conflicting evidence and/or divergence of opinion.
BBBs can be classified as complete versus incomplete, and right bundle branch block (RBBB) versus LBBB. BBBs may be normal variants in some individuals, or the result of age-related fibrosis in the conduction system. Nonetheless, they can be associated with important underlying conditions. For example, LBBB may be related to structural heart disease (i.e., hypertensive heart disease, IHD, cardiomyopathies, valvular heart disease), while RBBB may be the result of elevated right ventricular pressures (i.e., pulmonary hypertension, cor pulmonale, pulmonary embolism), prior radiation exposure, myocarditis, and structural heart disease (i.e., IHD, cardiomyopathies, valvular heart disease, congenital heart disease). The presence of a BBB is not itself an indicator of increased perioperative cardiovascular risk, especially after accounting for known clinical risk factors. Nonetheless, data from the nonoperative setting suggest that a recent onset or new diagnosis of a bundle branch should prompt a more extensive evaluation for undiagnosed cardiovascular disease. For example, LBBB is associated with increased risks of incident cardiovascular disease, cardiovascular mortality, and mortality in the nonoperative setting. Additionally, RBBB is associated with increased risks of mortality in patients with suspected IHD, known IHD, and heart failure. Based on this approach, if preoperative evaluation does not suggest significant pulmonary disease, IHD, structural heart disease, or Brugada syndrome (see section on “Brugada Syndrome”), no further preoperative evaluation of an isolated asymptomatic RBBB is warranted. Conversely, RBBB in a patient with known or suspected pulmonary disease (e.g., pulmonary hypertension) may suggest severe respiratory or vascular compromise; thus, consideration should be given to pulmonary evaluation and echocardiography if intermediate- or high-risk surgery is planned.
Atrial Fibrillation
Atrial fibrillation is a common arrhythmia characterized by a variable irregular ventricular response, and an absence of regular or organized atrial activity. The arrhythmia is classified as paroxysmal (i.e., terminates spontaneously or with intervention within 7 days of onset), persistent (i.e., fails to self-terminate within 7 days), long-standing persistent (i.e., lasts more than 12 months), or permanent (i.e., persistent atrial fibrillation where a joint decision has been made by the patient and clinician to no longer pursue a rhythm control strategy). Atrial fibrillation can occur in the absence of any structural heart disease (previously termed “lone atrial fibrillation”), or in combination with underlying disorders. In high-income countries, hypertension and ischemic heart disease are the most common underlying disorders, while in low and middle-income countries, rheumatic heart disease is a very common associated condition. Other associated conditions include valvular heart disease, heart failure, hypertrophic cardiomyopathy, congenital heart disease, obesity, diabetes mellitus, CKD, and increased age. Potentially reversible acute triggers for atrial fibrillation are hyperthyroidism and recent surgery (especially cardiac or thoracic surgery).
Affected individuals are at elevated risk for death, heart failure, thromboembolic events (i.e., stroke), and hospitalization. In the nonoperative setting, treatment involves ventricular rate control, consideration of rhythm control to restore sinus rhythm, and prevention of systemic embolization. In large randomized trials, strategies that focused on heart rate control versus rhythm control have shown similar effects on the risks of stroke or death. The CHA 2 DS 2 -VASc score ( Table 31.10 ) can be used to estimate the long-term systematic embolization risk in patients with atrial fibrillation. Based on this score, patients can be classified as low-risk (0 points: 0.2% annualized stroke rate), intermediate-risk (1 point: 0.6% annualized stroke rate), or high-risk (≥2 points: >2.2% annualized stroke rate). Based on strong randomized controlled trial data, current ACC/AHA guidelines recommend long-term oral anticoagulation for patients with nonvalvular atrial fibrillation (i.e., unrelated to mitral stenosis or prosthetic heart valves) and CHA 2 DS 2 -VASc scores of 2 or more, and support omitting anticoagulation in individuals with CHA 2 DS 2 -VASc scores of zero. There is more uncertainty about the best treatment for individuals with CHA 2 DS 2 -VASc scores of 1. Consistent with this uncertainty, ACC/AHA guidelines indicate that (1) oral anticoagulant therapy, (2) aspirin therapy, or (3) omitting anticoagulation are all reasonable approaches in these intermediate-risk patients. With respect to specific anticoagulant therapy, patients can be prescribed either a vitamin K antagonist (e.g., warfarin) or DOAC (e.g., dabigatran, rivaroxaban, edoxaban, apixaban) as reasonable options for patients with nonvalvular atrial fibrillation.
Risk Factor | Points |
---|---|
Heart Failure Associated signs and symptoms, or left ventricular systolic dysfunction | 1 |
Hypertension | 1 |
Age ≥ 75 years | 2 |
Diabetes mellitus | 1 |
Previous stroke, transient ischemic attack, or thromboembolism | 2 |
Vascular Disease Myocardial infarction, peripheral artery disease, or aortic plaque | 1 |
Age 65–74 years | 1 |
Female sex | 1 |
Although atrial fibrillation has typically been ignored during preoperative cardiac risk assessment, accumulating evidence challenges this approach in two main respects. Specifically, preexisting atrial fibrillation appears to be an indicator of increased perioperative risk. For example, in a large international multicenter prospective cohort study, preexisting atrial fibrillation was associated with an elevated risk of postoperative cardiovascular events (i.e., stroke, cardiovascular death, myocardial injury, heart failure, or cardiac arrest), but not death. In an American population-based retrospective cohort study, chronic atrial fibrillation was associated with a significantly higher risk of perioperative stroke.
The preoperative evaluation of patients with atrial fibrillation focuses on underlying conditions (e.g., ischemic heart disease), complications (e.g., heart failure, stroke), rate or rhythm control strategies, and anticoagulation strategies. Patients with rapid ventricular rates (>100 beats/min) typically require rate control before any elective surgery. Patients with both atrial fibrillation and slow ventricular rate without rate-controlling medications may have sick sinus syndrome. They should be questioned regarding any previous episodes of syncope or presyncope. Any long-term β-adrenergic blockers, digoxin, calcium channel blockers, or antiarrhythmic medications should be continued.
The critical component for preoperative planning for most patients with atrial fibrillation is appropriate perioperative management of long-term anticoagulants. This management should ideally be conducted collaboratively with the treating physician and responsible surgeon. There are three overarching issues, namely (1) whether temporary preoperative discontinuation is needed, (2) when oral anticoagulants should be discontinued, and (3) whether bridging therapy with LMWH is required. It is reasonable to continue vitamin K antagonist therapy if an individual does not have patient-related risk factors for bleeding (e.g., liver disease, abnormal renal function, prior bleeding complications), and is scheduled for a procedure without important bleeding risk (e.g., dental extraction, simple cutaneous procedures, pacemaker insertion). Otherwise, patients should have their anticoagulant medications temporarily discontinued before surgery, including all patients who are receiving DOACs or might need neuraxial anesthesia. If a decision to temporarily discontinue oral anticoagulants is made, vitamin K antagonists should be stopped 5 days before surgery. A longer discontinuation period may be needed for initial international normalized ratio (INR) values greater than 3.0. Ideally, the INR should be rechecked within 24 hours before surgery, and a low dose of oral vitamin K administered for any INR result above 1.5. The timing of preoperative discontinuation of DOACs ( Table 31.11 ) should be guided by the specific drug prescribed, expected procedural bleeding risk, renal function (based on estimated glomerular filtration rate [GFR]), and planned use of neuraxial anesthesia. The ongoing multicenter Perioperative Anticoagulant Use for Surgery Evaluation prospective cohort study is expected to provide more high-quality data on the safety of a further simplified strategy for preoperative discontinuation of DOACs ( Table 31.12 ).
Direct Thrombin Inhibitor (i.e., Dabigatran) | Direct Factor Xa Inhibitor (i.e., Rivaroxaban, Edoxaban, Apixaban) |
---|---|
Low Bleeding Risk Procedures (ACC Recommendations) ∗ | |
eGFR ≥ 80 mL/min: ≥ 24 h eGFR 50–79 mL/min: ≥ 36 h eGFR 30–49 mL/min: ≥ 48 h eGFR 15–29 mL/min: ≥ 72 h eGFR < 15 mL/min: No data | eGFR ≥ 30 mL/min: ≥ 24 h eGFR 15–29 mL/min: ≥ 36 h eGFR < 15 mL/min: No data (consider ≥ 48 h) |
Uncertain, Intermediate, or High Bleeding Risk Procedures (ACC Recommendations) ∗ | |
eGFR ≥ 80 mL/min: ≥ 48 h eGFR 50–79 mL/min: ≥ 72 h eGFR 30–49 mL/min: ≥ 96 h eGFR 15–29 mL/min: ≥ 120 h eGFR < 15 mL/min: No data | eGFR ≥ 30 mL/min: ≥ 48 h eGFR < 30 mL/min: No data (consider ≥ 72 h) |
Planned Neuraxial Anesthesia (ASRA Recommendations) † | |
Uniform approach: 120 h Approach based on eGFR
| 72 h |
∗ From Doherty JU, Gluckman TJ, Hucker WJ, et al. 2017 ACC Expert Consensus Decision Pathway for Periprocedural Management of Anticoagulation in Patients With Nonvalvular Atrial Fibrillation: A Report of the American College of Cardiology Clinical Expert Consensus Document Task Force . J Am Coll Cardiol . 2017;69:871–898.
† From Horlocker TT, Vandermeuelen E, Kopp SL, et al. Regional anesthesia in the patient receiving antithrombotic or thrombolytic therapy: American Society of Regional Anesthesia and Pain Medicine Evidence-Based Guidelines (Fourth Edition). Reg Anesth Pain Med . 2018;43:263–309.
Direct Thrombin Inhibitor (i.e., Dabigatran) | Direct Factor Xa Inhibitor (i.e., Rivaroxaban, Apixaban) |
---|---|
Low Bleeding Risk Procedures (No Planned Neuraxial Anesthesia) | |
eGFR ≥ 50 mL/min: last dose 2 days before surgery eGFR 30–49 mL/min: last dose 4 days before surgery | eGFR ≥ 30 mL/min: last dose 2 days before surgery |
High Bleeding Risk Procedures (No Planned Neuraxial Anesthesia) | |
eGFR ≥ 50 mL/min: last dose 3 days before surgery eGFR 30–49 mL/min: last dose 5 days before surgery | eGFR ≥ 30 mL/min: last dose 3 days before surgery |
Accumulating evidence indicates that most patients with nonvalvular atrial fibrillation do not require bridging therapy if their anticoagulant therapy is temporarily discontinued before surgery. For example, in the multicenter Bridging Anticoagulation in Patients Who Require Temporary Interruption of Warfarin Therapy for an Elective Invasive Procedure and Surgery (BRIDGE) randomized trial, placebo was non-inferior to LMWH bridging therapy with respect to risks of arterial thromboembolism following perioperative interruption of vitamin K antagonist therapy. In addition, bridging therapy led to increased risks of major bleeding. When interpreting the trial data, the challenge pertains to their generalizability to patients in clinical practice. For example, about 62% of the 1884 participants in the BRIDGE trial had an expected 1-year stroke rate less than 5% (i.e., equivalent to CHA 2 DS 2 -VASc score ≤ 4), while about 14% had an expected 1-year stroke rate over 10% (equivalent to CHA 2 DS 2 -VASc score ≥ 7). To help clinicians better navigate clinical decision making, the ACC published a 2017 expert consensus report on perioperative anticoagulation management in nonvalvular atrial fibrillation. In the case of vitamin K antagonist therapy, the recommended approach is to omit bridging therapy in low-risk patients who have CHA 2 DS 2 -VASc scores of 4 or less and no prior stroke, TIA, or systemic embolization. Conversely, bridging therapy should be considered for high-risk patients with CHA 2 DS 2 -VASc scores of 7 or more, recent (i.e., prior 3 months) stroke, TIA, or systemic embolization. For intermediate-risk patients (i.e., those not meeting either the low-risk or high-risk criteria), bridging therapy should be considered if there is no significant bleeding risk and a more remote time (more than 3 months) of stroke, TIA, or systemic embolization. Bridging therapy is generally not needed after preoperative interruption of DOACs because of their relatively short half-lives.
Supraventricular Arrhythmias
Supraventricular tachycardia can result from rapidly firing ectopic atrial foci with rapid conduction through the AV node, or from a reentry mechanism. Pathways for a reentry mechanism typically involve the AV node-Purkinje system and an accessory reentry pathway. In a reentry mechanism, the cycle self-perpetuates because conduction occurs down one pathway and up the other. Pharmacologic AV blockade (i.e., adenosine, verapamil, β-adrenergic blockers) can help slow the ventricular rate in most supraventricular tachycardias, with the exception of Wolff-Parkinson-White (WPW) syndrome. WPW syndrome is characterized by the presence of an accessory pathway (bundle of Kent) that permits both retrograde and antegrade conduction. Antegrade conduction over the accessory pathway results in a short PR interval (<0.12 ms) and a slurring of the upstroke of the QRS complex (termed a delta wave). Individuals with WPW syndrome are predisposed to supraventricular tachycardia. Additionally, treatment of supraventricular tachycardia in affected individuals with AV nodal blocking drugs can paradoxically increase conduction over the accessory pathway, thus potentially causing ventricular fibrillation. Thus, acute supraventricular tachycardia in these patients should be treated with electrical cardioversion (especially if hemodynamically unstable), ibutilide, or procainamide. Long-term management of WPW syndrome typically involves catheter ablation of the recurrent accessory pathway. Long-term antiarrhythmic medications should be continued perioperatively in patients with known supraventricular tachycardia.
Ventricular Arrhythmias
Ventricular ectopic beats can be differentiated from atrial ectopic beats by a wide QRS complex (>0.12 ms) and absence of an associated P-wave. To help predict the risk of sudden death, ventricular arrhythmias may be classified based on the type of rhythm disturbance and presence of underlying heart disease.
- ▪
Benign ventricular arrhythmias include isolated ventricular premature beats (VPBs) without associated heart disease. Affected individuals have no increased risk of sudden cardiac death, and do not need further cardiology evaluation.
- ▪
Potentially lethal arrhythmias include more than 30 VPBs per hour, or nonsustained ventricular tachycardia in association with underlying heart disease. Affected individuals are at moderately high risk of sudden cardiac arrest and may benefit from an ICD. They also require cardiology evaluation and echocardiography, along with the possible need for cardiac stress testing, coronary angiography, and electrophysiology testing.
- ▪
Lethal arrhythmias include sustained ventricular tachycardia, ventricular fibrillation, and VPBs associated with underlying heart disease, depressed cardiac function, and hemodynamic compromise. Affected individuals are at high risk for sudden cardiac arrest, and likely benefit from an ICD. They also require cardiology evaluation and echocardiography, along with possible cardiac stress testing, coronary angiography, and electrophysiology testing. In general, reversible causes of ventricular arrhythmias (e.g., hypokalemia, ischemia, acidosis, hypomagnesemia, drug toxicity, endocrine dysfunction) should be sought out and treated. Any long-term antiarrhythmic medications should be continued perioperatively.
Long QT Syndrome
The long QT syndrome (LQTS) is a myocardial repolarization disorder associated with a prolonged QT interval. The QT interval is measured using a 12-lead ECG (preferably leads II and V5) from the onset of the QRS complex to the end of the T wave. Since the interval varies inversely with heart rate, a corrected QT interval (QT c ) can be calculated, with the most common formula being:
QTc=QTintervalRRinterval
In adults, an abnormally prolonged QTc is defined as a value exceeding the 99th percentile. The QTc is prolonged if exceeding 0.48 seconds in women and 0.47 seconds in men. Individuals with LQTS are predisposed to developing torsades de pointes , a polymorphic ventricular tachycardia with frequent variations of the QRS axis or morphology. Affected patients are generally asymptomatic in the absence of arrhythmias. Conversely, after the onset of an arrhythmia, they may develop palpitations, syncope, seizures, and sudden cardiac death.
LQTS may be either congenital (i.e., genetic) or acquired. Causes of acquired LQTS include antiarrhythmic drugs (e.g., quinidine, sotalol, dofetilide, ibutilide), psychotropic medications (e.g., haloperidol, methadone), erythromycin, cisapride, and metabolic abnormalities (i.e., hypokalemia, hypomagnesemia, hypocalcemia). Notably, while amiodarone does markedly prolong the QT interval, it is rarely associated with torsades de pointes except in association with hypokalemia. The 2010 ACC/AHA scientific statement on the prevention of torsades de pointes suggests that QT c be documented before initiation or after an increased dose of QT-prolonging drugs, and thereafter at least every 8 to 12 hours. Other components of treatment for LQTS include β-adrenergic blockers (congenital LQTS), ICD implantation, and correction of underlying metabolic disorders.
Brugada Syndrome
Brugada syndrome is a rare cause of sudden cardiac arrest that occurs in the absence of structural heart disease. It is an autosomal dominant disorder that is more common in men, rarely diagnosed in children, and often affects individuals of Asian ethnicity. Patients usually have normal findings on echocardiography, stress testing, and cardiac magnetic resonance imaging (MRI). The most significant clinical manifestations are ventricular arrhythmias, syncope, and sudden death. Patients may also be at increased risk of atrial arrhythmias, especially atrial fibrillation. Brugada syndrome is characterized by an ECG with pseudo-RBBB and persistent ST-segment elevation in leads V 1 to V 3 ( Fig. 31.7 ). Unlike usual RBBB, a widened S wave in the left lateral leads is usually absent in Brugada syndrome. In some patients, these ECG changes are transient and provoked by medications. Asymptomatic patients who have these typical ECG features but no other associated clinical criteria have been described as having “Brugada pattern.” The website www.brugadadrugs.org lists drugs that are associated with adverse events in Brugada syndrome. These medications include some commonly used anesthetic drugs, such as propofol and bupivacaine. The syndrome has no proven pharmacologic treatment; indeed, class I antiarrhythmic medications (e.g., flecainide, procainamide) and β-adrenergic blockers can worsen the risk of lethal arrhythmias. ICD implantation is the standard of care for Brugada syndrome.

Cardiovascular Implantable Electronic Devices
Cardiovascular implantable electronic devices (CIEDs), which include both permanent pacemakers and ICDs, are very common. For example, in the United States alone, about 190,000 permanent pacemakers and 145,000 ICDs are implanted every year (see Chapter 38). The preoperative evaluation should characterize the device with respect to type, age, manufacturer, model number, current settings, and timing of recent interrogation. Patients frequently carry a manufacturers’ identification card with some of this relevant information. Permanent pacemaker capabilities are usually classified using a five-letter code ( Table 31.13 ). The anesthesiologist should also evaluate any coexisting cardiac disease because patients with CIEDs invariably have conditions such as heart failure, IHD, valvular heart disease, or potentially lethal arrhythmias—all of which have perioperative implications. It is especially important to note device features (e.g., rate modulation) that can malfunction with perioperative electromagnetic and other interferences. Sources of electromagnetic interference during the perioperative period include electrocautery (especially monopolar), radiofrequency ablation, lithotripsy devices, and radiation therapy, while direct mechanical interference can occur with guidewire movement during central venous catheter insertion. These sources of interference can result in CIED malfunction, such as inappropriate ICD discharge or inappropriate changes in pacing rates. Especially during delicate surgical procedures (e.g., intracranial, spinal, ocular), an unexpected ICD discharge with movement of the patient can have catastrophic results.
Position I | Position II | Position III | Position IV | Position V |
---|---|---|---|---|
Chamber(s) Paced | Chamber(s) Sensed | Response to Sensing | Rate Modulation | Multisite Pacing |
O = None | O = None | O = None | O = None | O = None |
A = Atrium | A = Atrium | I = Inhibited | R = Rate modulation | A = Atrium |
V = Ventricle | V = Ventricle | T = Triggered | V = Ventricle | |
D = Dual (A + V) | D = Dual (A + V) | D = Dual (T + I) | D = Dual (A + V) |
In general, the anesthesiologist conducting a preoperative evaluation of a patient with a CIED should collaboratively plan perioperative management with the relevant CIED care team ( Box 31.7 ). The CIED care team has been defined as the physicians and physician extenders who monitor the patient’s CIED function. Ideally, patients with CIEDs should have these devices interrogated preoperatively, with recommendations that this be performed within the prior 6 months for an ICD, prior 12 months for a permanent pacemaker, and prior 3 to 6 months for any CRT device. Key issues for consideration when planning the perioperative care of a patient with a CIED include whether the patient has an ICD, whether the patient is pacemaker-dependent, and what happens with magnet placement over the CIED. In general, a magnet suspends antitachyarrhythmia therapy in most ICDs, and switch pacemakers (but not ICDs) to an asynchronous pacing mode. There is some disagreement among guidelines whether to place a magnet over the CIED during the intraoperative period, versus arrange for temporary device reprogramming (i.e., switch pacemaker to asynchronous mode, and disable antitachyarrhythmia ICD). Especially given the complexity of newer generation CIEDs, routine magnet use should not be viewed as an alternative for appropriate preoperative preparation. Collaboration with the CIED care team is the preferred approach for managing these patients in an individualized manner. Preoperative recommendations for CIEDs are presented in Box 31.8 . In addition, these patients require a preoperative ECG. A chest radiograph is not mandatory before surgery but can reveal the device location and manufacturer’s code.
The perioperative management of CIEDs must be individualized to the patient, type of CIED, and procedure being performed. A single recommendation for all CIED patients is not appropriate.
The CIED care team is defined as the physicians and physician extenders who monitor the CIED function of the patient.
The surgical or procedural team should communicate with the CIED care team to identify the type of procedure and likely risk of EMI.
The CIED care team should communicate with the procedure team to deliver a prescription for the perioperative management of patients with CIEDs.
For most patients, the prescription can be made from a review of the records of the CIED clinic. A small percentage of patients may require consultation from CIED specialists if the information is not available.
It is inappropriate to have industry-employed allied health professionals independently develop this prescription.
CIED , Cardiovascular implantable electronic devices; EMI, electromagnetic interference.
- ▪
Inactivation of ICDs is not absolutely necessary for all procedures
- ▪
Not all pacemakers need to be altered to pace asynchronously in all patients or for all procedures
- ▪
Pacemakers can be reprogrammed or magnets can be used to force pacemakers to pace asynchronously to prevent inhibition
- ▪
ICDs can be reprogrammed or magnets can be used to inhibit ICD arrhythmia detection and tachyarrhythmia functions
- ▪
Magnets can/will not force pacemakers in ICDs to pace asynchronously
- ▪
Inactivation of ICDs is recommended for all procedures above the umbilicus involving electrocautery or radiofrequency ablation
- ▪
It is preferable to change to asynchronous pacing in pacemaker-dependent patients for procedures involving electrocautery or radiofrequency ablation above the umbilicus
The procedure team provides the following information to the CIED team:
- ▪
Type of procedure
- ▪
Anatomic site of procedure
- ▪
Patient position during procedure
- ▪
Will electrocautery (and type of cautery) be used?
- ▪
Are there other sources of EMI?
- ▪
Other issues such as likelihood of damage to leads (e.g., chest procedures), anticipated large blood loss, and surgery in close proximity to CIED
The CIED care team provides the following information to the procedure team:
- ▪
Type of device (e.g., pacemaker, ICD)
- ▪
Indication for device (e.g., sick sinus syndrome, primary or secondary prevention of lethal arrhythmias)
- ▪
Programming (e.g., pacing mode, rate, rate responsive, heart rates for shock delivery)
- ▪
Is the patient pacemaker-dependent, and what is the underlying heart rate/rhythm?
- ▪
Magnet response
- ▪
Pacing rate
- ▪
Is the device responsive to a magnet?
- ▪
Will ICD functions resume automatically with magnet removal?
- ▪
Does magnet need to be placed off-center?
- ▪
CIED, Cardiovascular implantable electronic device; EMI, electromagnetic interference; ICD, implantable cardioverter-defibrillator.
Peripheral Artery Disease
PAD, which is defined as atherosclerosis of the large-sized and medium-sized noncardiac arterial vasculature, affects about 200 million people worldwide. PAD is diagnosed based on an ankle-brachial index (ABI or ratio of ankle-to-arm systolic blood pressure) of less than 0.90. The disease generally affects lower extremity vessels more than upper extremity vessels. PAD progresses variably across individuals, from an initially asymptomatic phase to intermittent claudication (i.e., extremity pain with activity) to critical limb ischemia. Risk factors for PAD include increased age, smoking, hypertension, diabetes mellitus, CKD, and known atherosclerosis in other sites (e.g., IHD). Conversely, patients with PAD likely have atherosclerosis in other sites (e.g., heart, brain). For example, a systematic review in the nonoperative setting found a prevalence of IHD in individuals with PAD ranged from 60% based on cardiac stress testing to 90% based on coronary angiography. Individuals meeting diagnostic criteria for PAD (i.e., ABI < 0.90) have elevated risks for subsequent myocardial infarction, acute stroke, or cardiovascular death in the nonoperative setting. PAD is also a marker of increased risk in surgical patients. An ABI less than 0.90 is independently associated with an increased risk of cardiovascular complications after noncardiac surgery. Known PAD is also associated with elevated risks of mortality after major noncardiac surgery. In addition, claudication related to PAD generally limits functional capacity and thereby masks the symptoms of underlying IHD or heart failure.
The preoperative evaluation of PAD should encompass related symptoms (e.g., intermittent claudication, rest pain), risk factors (e.g., hypertension, diabetes mellitus, smoking, CKD), and associated comorbidities (e.g., IHD, CVD). Arterial blood pressure should be measured in both upper extremities, along with the presence of peripheral arterial pulses. Auscultation for bruits over the abdomen and femoral arteries, as well as palpation for abdominal masses, should be part of the vascular examination. Typical preoperative laboratory testing includes an ECG and blood sampling for a complete blood count, creatinine concentration, and glucose concentration. These individuals may often need further specific workup for IHD with testing such as natriuretic peptides and cardiac stress testing (see section on “Ischemic Heart Disease”). Depending on concomitant risk factors and cardiovascular medications, patients may be on long-term therapy with aspirin, P2Y 12 inhibitors (e.g., clopidogrel), and DOACs. DOACs should be temporarily discontinued before surgery (see section on “Atrial Fibrillation”). In most cases, P2Y 12 inhibitor therapy should also be interrupted before surgery, with the possible exception of cases with very recent coronary stent implantation (see section on “Coronary Stents”). As a general strategy for patients undergoing noncardiac surgery, continuing aspirin perioperatively does not prevent cardiovascular complications except in those with drug-eluting coronary stents, but leads to an increased risk of major bleeding (which is a risk factor for perioperative stroke). Nonetheless, selective continuation of aspirin should be considered in patients undergoing vascular surgery (to mitigate risks of bypass graft occlusion), as well as those with high-risk IHD, prior PCI, or recent stroke (i.e., previous 9 months).
Pulmonary Disorders
See Chapters 13, Chapters 53 .
Asthma
The Global Initiative for Asthma ( http://ginasthma.org ), describes asthma as a “ heterogeneous disease, usually characterized by chronic airway inflammation. It is defined by the history of respiratory symptoms such as wheeze, shortness of breath, chest tightness, and cough that vary over time and in intensity, together with variable expiratory airflow limitation. ” These episodes of airflow obstruction within the lung are often reversible, either spontaneously or with treatment. The airway obstruction can be precipitated by irritants (e.g., smoke), allergens, infections, medications, or airway instrumentation. Worldwide, it is estimated that asthma afflicts more than 300 million people, and is responsible for one in every 250 deaths. Asthma is classified as intermittent, persistent mild, persistent moderate, and persistent severe, based on symptoms, nighttime awakenings, short-acting bronchodilator requirement, functional impairment, and pulmonary function test (PFT) abnormalities. Although spirometry is the preferred diagnostic test for asthma, a normal result does not exclude asthma. Typical findings on PFTs are a reduction in the ratio of the forced expiratory volume in 1 second (FEV 1 ) to forced vital capacity (FVC)—with a ratio below 0.7 being indicative of airflow obstruction. Importantly, normal initial PFT results do not necessarily exclude asthma. If results are normal but a diagnosis of asthma is still strongly suspected, a methacholine challenge test or a trial of bronchodilator therapy should be performed. Patients with mild well-controlled asthma have no greater perioperative risk than do individuals without asthma. In addition, PFTs, while helping to establish the diagnosis of asthma, generally have no perioperative prognostic value in these patients.
In a patient with known asthma, the anesthesiologist should inquire about dyspnea, chest tightness, cough (especially nocturnal), recent exacerbations (with associated triggers), therapy (especially corticosteroids), prior hospitalizations, prior emergency department visits, prior critical care unit admissions, prior need for endotracheal intubation, and recent upper respiratory tract infections (see section on “Upper Respiratory Tract Infections”). It is helpful to ask patients to compare their current asthma symptoms to their “normal” or “best” status based on symptoms, exercise tolerance, and medication requirements. The physical examination should assess the quality of breath sounds, quantity of air movement, degree of wheezing, and oxygen saturation by pulse oximetry. The degree of wheezing does not always correlate with the severity of bronchoconstriction. With severe obstruction, airflow is dangerously restricted, and wheezing diminishes. Wheezing is common in asthma but it is not specific for the disease. For example, wheezing may be present in chronic obstructive pulmonary disease (COPD), gastroesophageal reflux disease, vocal cord dysfunction, tracheal stenosis, bronchial stenosis, cystic fibrosis, allergic bronchopulmonary aspergillosis, and heart failure. Observing the degree of accessory muscle use can also help gauge the severity of bronchoconstriction.
Arterial blood gases are not necessary unless the patient is having a severe acute exacerbation. Patients taking oral corticosteroids should have their blood glucose checked. Chest radiography is needed only if an infection or pneumothorax is suspected. Bronchodilators, corticosteroids (inhaled and oral), and any antibiotics must be continued on the day of surgery. β-adrenergic agonists are a useful prophylactic intervention to lower the risk of bronchospasm after induction of anesthesia. This therapy can be supplemented with a short preoperative course of oral corticosteroids (prednisone 20 mg-60 mg daily for 3-5 days) in any newly diagnosed or poorly controlled asthmatic patient. Importantly, some asthmatics on chronic corticosteroid treatment may need perioperative “stress dose steroids” (see section on “Hypothalamic-Pituitary-Adrenal Disorders”).
Chronic Obstructive Pulmonary Disease
COPD is a chronic respiratory disease that affects 175 million people worldwide, and causes 3 million deaths every year. It is characterized by persistent airflow obstruction that occasionally is partially reversible (see Chapter 13, Chapter 53 ). It may be the result of smoking, environmental pollutants (e.g., air pollution), α 1 -antitrypsin deficiency, chronic infections, and long-standing asthma. The Global Initiative for Chronic Obstructive Lung Disease describes COPD as a “ common, preventable, and treatable disease that is characterized by persistent respiratory symptoms and airflow limitation that is due to airway and/or alveolar abnormalities usually caused by significant exposure to noxious particles or gases ” ( http://www.goldcopd.org ). COPD includes subtypes such as “chronic bronchitis” and “emphysema.” Chronic bronchitis is defined as a chronic productive cough for 3 months in each of 2 successive years in a patient in whom other causes of chronic cough (e.g., bronchiectasis) have been excluded. Emphysema refers to pathologic structural changes in the lung that can occur with COPD, including enlargement of airspaces distal to the terminal bronchioles, as well as destruction of these airspace walls. The severity of COPD is classified based on both spirometry findings and symptoms. Once a patient has evidence of airway obstruction on spirometry (FEV 1 /FVC ratio < 0.7), mild FEV 1 airflow limitation is at or greater than 80% of predicted, moderate limitation is FEV 1 between 50% and 79% of predicted, severe limitation is FEV 1 between 30% and 49% of predicted, and very severe limitation is FEV 1 is less than 30% of predicted. Symptom severity is classified separately using validated scales. A COPD exacerbation is defined as “ an acute worsening of respiratory symptoms that result in additional therapy ” ( http://www.goldcopd.org ).
The preoperative evaluation of patients with COPD is similar to that of patients with asthma, with an additional emphasis on signs of recent infection (e.g., changes in sputum amount or color). A barrel chest and pursed-lip breathing also suggest advanced disease. COPD is a known risk factor for postoperative pulmonary complications (see section on “Postoperative Pulmonary Complications”). Nonetheless, there is no established threshold of COPD severity indicative of highly prohibitive perioperative risk. In general, PFTs are not useful for estimating perioperative risk in patients with COPD, with the exception of individuals undergoing lung resection surgery (see Chapter 53 and section on “Patients Scheduled for Lung Resection”). In general, preoperative PFTs are indicated when there is uncertainty as to whether the patient’s lung function is optimized, or uncertainty as to the underlying basis for unexplained dyspnea. Patients who are hypoxic, or require supplemental oxygen, may benefit from further testing, including arterial blood gas measurement, in addition to routine measurement of oxygen saturation using pulse oximetry. A chest radiograph is useful only if infection or bullous disease is suspected. A key goal in the preoperative preparation of a patient with COPD is optimizing pulmonary function before any elective surgery. Accordingly, patients with a recent exacerbation may require more intensive bronchodilator treatment, brief courses of antibiotics or oral corticosteroids, and possible deferment of elective surgery. Smoking cessation should be encouraged for any ongoing smokers (see section on “Smokers and Second-Hand Smoke Exposure”). Additionally, consideration can be given to preoperative inspiratory muscle training and physiotherapy in high-risk patients, and discussion about the potential respiratory benefits of neuraxial anesthesia or analgesia (see section on “Postoperative Pulmonary Complications”). Inhalers and other long-term medications for COPD should be continued on the day of surgery, and individuals on chronic corticosteroid treatment may need “stress dose steroids” (see section on “Hypothalamic-Pituitary-Adrenal Disorders”).
Restrictive Pulmonary Disorders
Restrictive lung disease is characterized by a reduced total lung capacity and may be related to both pulmonary or extrapulmonary conditions. Pulmonary causes include idiopathic interstitial pneumonia, prior lung resection, pulmonary fibrosis, and interstitial lung disease secondary to connective tissue disease. Extrapulmonary causes include chest wall limitations (e.g., kyphoscoliosis, obesity, ankylosing spondylitis), muscle dysfunction (e.g., muscular dystrophies, myasthenia gravis, diaphragm paralysis), and pleural disease (e.g., mesothelioma, effusion, pneumothorax). In a patient with suggestive symptoms or history of associated diseases, a chest radiograph and PFTs can help establish the diagnosis. Typically, the FEV 1 and the FVC are reduced proportionally such that the FEV 1 /FVC ratio is normal (i.e., > 0.7). Preoperative PFTs can also help assess for acute or progressive worsening of known restrictive lung disease; however, routine testing is not necessary in the absence of clinical suspicion. These patients are also at risk of pulmonary hypertension that may not be recognized because of overlapping symptoms with restrictive lung disease. Thus, echocardiography may also be indicated to investigate causes of worsening symptoms in a patient with known restrictive lung disease.
Patients Scheduled for Lung Resection
Most patients scheduled for lung resection operations have underlying lung disease (this topic is covered in more detail in Chapter 53 ). Spirometry is useful in estimating risk and excluding patients who are highly likely to lack adequate pulmonary reserve after the planned resection (see Chapter 53 ). Current American College of Chest Physicians (ACCP) guidelines recommend measurement of both FEV 1 and diffusing capacity for carbon monoxide (D LCO ) in all patients being considered for lung resection surgery. The amount of residual lung function after resection can be estimated by using a combination of spirometry and radionuclide quantitative lung scanning. The predicted postoperative FEV 1 (PPO FEV 1 ) is predicted by multiplying the preoperative FEV 1 by the percentage of perfusion to the nonoperative lung or lung region:
PPOFEV1=PreoperativeFEV1×PerfusiontononresectedlungTotalperfusiontolungs
The PPO D LCO is calculated using an analogous equation for preoperative D LCO . Patients with both PPO FEV 1 and PPO D LCO values exceeding 60% of predicted are considered low-risk and can generally proceed directly to surgery. If either value is within the range of 30% to 60% of predicted, simple objective exercise testing with a shuttle walk test or symptom limited stair climbing test is recommended. For individuals with poor performance on these simple tests (i.e., < 400 m on shuttle walk test or < 22 m on stair climbing test), as well as for individuals with either PPO FEV 1 or PPO D LCO values less than 30% predicted, the ACCP guidelines recommend CPET to measure peak oxygen consumption (VO 2 peak). A preoperative VO 2 peak greater than 20 mL/kg/min is consistent with low perioperative risk, 10 to 20 mL/kg/min is consistent with moderate risk, and less than 10 mL/kg/min is consistent with high risk. Nonsurgical options should be considered in high-risk scenarios, while shared decision making should be incorporated in any intermediate-risk scenarios.
Obstructive Sleep Apnea
In North America, the prevalence of sleep-disordered breathing is 9% among females aged 30 to 60 years of age, and 24% among males in the same age group (see Chapter 10 ). OSA is the most common type of sleep-disordered breathing, with varying prevalence across age groups, ethnicities, and countries. Recent North American estimates suggest that it afflicts about 9% of females aged 50 to 70 years, and 17% of males in this same age group. OSA is characterized by recurrent upper airway collapse during sleep that leads to reduced or complete cessation of airflow, despite ongoing breathing efforts. Affected individuals develop intermittent hypercapnia, intermittent hypoxemia, and fragmented sleep. Risk factors for OSA include increased age, male sex, obesity, smoking, pregnancy, heart failure, end-stage renal disease, and craniofacial abnormalities. The diagnosis of OSA is based on the presence of symptoms, such as nonrestorative sleep, snoring, hypertension, and the frequency of sleep-related respiratory events during polysomnography or home sleep apnea testing. Once diagnosed, disease severity is typically characterized by the apnea-hypopnea index (AHI), which is the number of apneic and hypopneic episodes per hour of sleep. Mild OSA is defined by 5 to 15 episodes per hour, moderate disease by 16 to 30 episodes per hour, and severe disease by more than 30 episodes per hour. OSA is associated with an increased prevalence of systemic hypertension, pulmonary hypertension, IHD, heart failure, cardiac arrhythmias (i.e., atrial fibrillation, bradyarrhythmias, ventricular ectopy), stroke, type 2 diabetes mellitus, obesity hypoventilation syndrome, and nonalcoholic fatty liver disease. The main effective treatments for OSA in the nonoperative setting are continuous positive airway pressure (CPAP) and weight loss.
Most cases of OSA in surgical patients remain undiagnosed at the time of surgery. Screening questionnaires can help, in part, address this problem. For example, the eight-item STOP-Bang questionnaire is a straightforward validated tool to screen for OSA in preoperative evaluation clinics ( Fig. 31.8 ). Surgical patients with scores of 2 or less are at very low risk (negative likelihood ratio 0.24 for AHI ≥ 5), and those with scores of 5 or more are at increased risk (positive likelihood ratio 1.8 for AHI ≥ 5). Since individuals with scores of 3 to 4 are in an indeterminate zone, additional screening criteria have been proposed for this subgroup, including serum bicarbonate concentration level at 28 mmol/L or greater, and differentially weighting responses to the questionnaire. There is some uncertainty as to the operational burden of screening all surgical patients for OSA, especially since the positive likelihood ratio for a higher STOP-Bang score is not particularly high. A reasonable option may be to apply screening in higher-risk populations, such as individuals with obesity, associated comorbidities, and known or suspected difficult intubation characteristics.

Patients with OSA are at increased perioperative risk because of the sleep disorder itself and associated comorbidities. Mask ventilation, direct laryngoscopy, endotracheal intubation, and fiberoptic visualization of the airway are more difficult in patients with OSA (see Chapter 44 ). In addition, these patients are also more sensitive to the respiratory depressant effects of opioids. In general, patients with OSA have elevated risks of perioperative airway obstruction, hypoxemia, atelectasis, pneumonia, cardiovascular complications, and prolonged hospitalizations. There is uncertainty as to how much of this increased risk is explained by OSA itself, versus associated comorbidities (e.g., IHD, heart failure, diabetes mellitus, obesity). For example, some cohort studies that found that patients with known OSA, or patients screened as being high-risk for OSA, were not at elevated risk of mortality or postoperative hypoxemia, after accounting for coexisting comorbidities.
Preoperative evaluation focuses on characterizing all cases of known OSA, and selectively identifying patients at risk for undiagnosed OSA. In addition, associated comorbidities should be investigated and optimized as deemed clinically appropriate. For example, echocardiography may be indicated if undiagnosed heart failure or pulmonary hypertension is suspected. For patients with known OSA, the anesthesiologist should inquire about known disease severity, document current treatment, and instruct the patient to bring their CPAP equipment or oral appliances on the day of surgery (so that therapy can be restarted promptly after surgery).
Pulmonary Hypertension
Pulmonary hypertension is defined as a persistent mean pulmonary artery pressure of 25 mm Hg or more at rest. The condition may occur in isolation or with associated medical conditions. Based on the World Health Organization, pulmonary hypertension is classified into 5 groups ( Box 31.9 ). Idiopathic and heritable pulmonary arterial hypertension (formerly called primary pulmonary hypertension) are relatively rare. Other more common forms occur with a variety of diseases including cardiac, pulmonary, liver, thromboembolic, and collagen vascular diseases (e.g., scleroderma, systemic lupus erythematosus [SLE]). Pulmonary hypertension is also associated with human immunodeficiency virus (HIV) infection, anorectic drug exposure (e.g., fenfluramine), OSA, and chronic liver disease (especially with portal hypertension).
Pulmonary Arterial Hypertension
- 1.
Idiopathic pulmonary arterial hypertension
- 2.
Heritable pulmonary arterial hypertension
- 3.
Drug-induced or toxin-induced pulmonary arterial hypertension
- 4.
Associated with other conditions
- (a).
Connective tissue disease
- (b).
Congenital heart disease
- (c).
Portal hypertension
- (d).
Human immunodeficiency virus infection
- (e).
Schistosomiasis
- (a).
- 5.
Pulmonary venoocclusive disease and/or pulmonary capillary hemangiomatosis
- 6.
Persistent pulmonary hypertension of newborn
Pulmonary Hypertension Related to Left-heart Disease
- 1.
Left ventricular systolic dysfunction
- 2.
Left ventricular diastolic dysfunction
- 3.
Valvular heart disease
- 4.
Extrinsic compression of central pulmonary veins
- 5.
Congenital or acquired obstruction of the left heart inflow or outflow tract, and congenital cardiomyopathies
Pulmonary Hypertension Related to Lung Disease or Hypoxemia
- 1.
Chronic obstructive pulmonary disease
- 2.
Interstitial lung disease
- 3.
Other pulmonary diseases with mixed restrictive and obstructive pattern
- 4.
Sleep disordered breathing
- 5.
Alveolar hypoventilation disorders
- 6.
Developmental lung disease
- 7.
Chronic exposure to high altitude
Chronic Thromboembolic Pulmonary Hypertension
Pulmonary Hypertension with Unclear Multifactorial Etiology
- 1.
Hematologic disorders (chronic hemolytic anemia, myeloproliferative disorders, splenectomy)
- 2.
Systemic disorders (sarcoidosis, pulmonary histiocytosis, lymphangioleiomyomatosis)
- 3.
Metabolic disorders (glycogen storage disease, Gaucher disease, thyroid disorders)
- 4.
Other conditions (tumor obstruction, fibrosing mediastinitis, chronic kidney disease, segmental pulmonary hypertension
Patients with pulmonary hypertension have a high rate of perioperative morbidity and mortality. Hypoxia, hypercarbia, hypothermia, vasoconstrictor use, and increased sympathetic tone (even from anxiety) during the perioperative period increase pulmonary vascular resistance, with the potential for acute decompensation with right-sided heart failure. Occult pulmonary hypertension is more problematic than the fully recognized disease because symptoms may be attributed to other diseases, and perioperative decompensation may occur unexpectedly. Current American and European guidelines recommend that affected patients be managed collaboratively with a pulmonary hypertension specialist team during the perioperative period, and that they undergo surgery at centers with requisite expertise.
It may be challenging to detect pulmonary hypertension de novo during preoperative evaluation. The initial symptoms of pulmonary hypertension are usually nonspecific and insidious. Diagnosis is also typically delayed, with about 20% of patients having symptoms for more than 2 years prior to a formal diagnosis. Typical initial symptoms are exertional dyspnea, lethargy, and fatigue. With disease progression, symptoms related to right ventricular overload develop—these symptoms include exertional chest pain, exertional syncope or presyncope, upper abdominal pain (i.e., liver congestion), and dependent edema. Physical examination may reveal a split S 2 with a loud second component, right ventricular heave, tricuspid regurgitation murmur, ascites, hepatomegaly, jugular venous distention, and peripheral edema. An ECG and echocardiogram are useful in patients with suspected pulmonary hypertension, and those with moderate-to-severe known disease. Typical ECG findings include right axis deviation, RBBB, right ventricular hypertrophy, and tall R waves in leads V 1 and V 2 . Right atrial hypertrophy and “P-pulmonale” may be present in severe pulmonary hypertension, with peaked P waves, usually in leads II, III, aVF, and V 1 . An echocardiogram is the initial screening test of choice for pulmonary hypertension. It can estimate pulmonary arterial pressures, assess right ventricular function, identify left-sided heart failure, and detect structural heart disease (e.g., valvular heart disease, congenital heart disease). Patients with significant echocardiographic findings may require subsequent right and left cardiac catheterizations, especially given the potential inaccuracy of estimating right-sided pressures by echocardiography alone. Other useful laboratory tests include complete blood count, electrolyte concentrations, creatinine concentrations, and liver function tests (i.e., liver congestion or drug-related side effects).
Patients with pulmonary hypertension may be receiving treatment with diuretics, calcium channel blockers, supplemental oxygen, phosphodiesterase type 5 inhibitors (e.g., sildenafil, tadalafil), endothelin receptor antagonists (e.g., bosentan, ambrisentan), and prostacyclin pathway agonists (e.g., iloprost, epoprostenol). Some drugs are given by continuous intravenous infusions, and even momentary interruption of therapy can be catastrophic. In general, all these medications should be continued perioperatively. Some patients may be anticoagulated. The timing of any preoperative anticoagulant discontinuation, and assessment for bridging therapy, should be made collaboratively with a pulmonary hypertension specialist team.
Smokers and Second-Hand Smoke Exposure
Tobacco exposure, either directly or through “second-hand” smoke, increases the risk of many perioperative complications, including respiratory, cardiac, and infectious events. Current smokers have elevated risks for a range of postoperative complications, including mortality, cardiac complications, pulmonary complications, acute stroke, and surgical site infections. Overall, about two thirds of smokers want to quit. The U.S. Public Health Service recommends that “ all physicians should strongly advise every patient who smokes to quit because evidence shows that physician advice to quit smoking increases abstinence rates .” Even brief advice by a physician to quit smoking increases quit rates (relative risk 1.66; 95% CI, 1.42-1.94), albeit from a very low baseline rate of 2% to 3%. Among intensive smoking cessation interventions, both behavioral counselling and medications have demonstrated efficacy, with evidence supporting further improvement in efficacy with combination therapy. The main pharmacologic interventions for smoking cessation are nicotine replacement therapy (i.e., nicotine patches, gum, or lozenges), varenicline, and bupropion. Both bupropion and varenicline should be started at least 1 week before an attempt at quitting. Many hospitals, insurance companies, communities, and governmental agencies offer smoking cessation programs. Excellent advice and guidelines are available online (e.g., https://www.cdc.gov/tobacco/quit_smoking/index.htm and https://smokefree.gov ) or via telephone (e.g., 1-800-QUIT-NOW in the United States).
Being scheduled for surgery is a “teachable moment” for promoting smoking cessation, with population-based survey data from the United States showing significant increases in smoking cessation rates after major surgery. Within this context, interventions in the preoperative evaluation clinic result in higher rates of short-term smoking cessation (i.e., within 3-6 months after surgery). Although there is uncertainty about the robustness of smoking cessation rates over the long term, randomized controlled trials have demonstrated reduced nicotine dependence of up to 1 year postoperatively following perioperative smoking cessation interventions.
The perioperative benefits of smoking abstinence are realized within the first year after cessation. Nonetheless, there remains uncertainty regarding the minimum required period to accrue benefits. In previous systematic reviews, clinical benefits of preoperative smoking cessation were demonstrated only when it occurred at least 3 to 4 weeks before surgery. These benefits include reduced risks for respiratory and wound healing complications. Despite an early study reporting an increased perioperative risk (this association was not statistically significant) in recent quitters, a systematic review found no increased risk of adverse events with quitting smoking soon before surgical procedures (i.e., within 8 weeks). Thus, motivated patients should be encouraged to quit smoking at any point before surgery. Quitting has many theoretical benefits, even within a few days before operation. Soon after a patient quits smoking, carbon monoxide levels decrease, thereby improving oxygen delivery and use. Cyanide levels decrease, which benefits mitochondrial oxidative metabolism. Other beneficial effects include lower nicotine levels (which improves vasodilatation) and clearance of many toxic substances that impair wound healing.
Upper Respiratory Tract Infections
Traditionally, elective surgical procedures were cancelled when patients, especially children, presented with current or recent upper respiratory tract infections. The concern pertains to associated airway hyperreactivity that predisposes patients to laryngospasm, bronchospasm, atelectasis, coughing, airway obstruction, hypoxia, stridor, and breath-holding. Prior evidence suggests that this airway hyperreactivity lasts for 2 to 4 weeks after an upper respiratory tract infection. Nonetheless, since most of these associated perioperative respiratory events are mild and easily managed with modern anesthesia care, cancellation is no longer routine. For patients with severe symptoms (e.g., high fever), especially in the presence of other health conditions (e.g., significant asthma, heart disease, immunosuppression), elective surgery should be postponed until 4 weeks after resolution of the infection. Conversely for mild or uncomplicated infections in otherwise healthy patients, it is reasonable to proceed with the planned surgery and avoid the inconvenience of a last-minute cancellation. The dilemma lies with patients between these two extremes, for whom decisions regarding the suitability to proceed should be made on an individualized basis.
Cystic Fibrosis
Cystic fibrosis is an autosomal recessive disease caused by altered chloride and water transport across epithelial cells. This genetic disorder can result in progressive chronic airway disease characterized by airway obstruction, destruction, and frequent pulmonary infections. Affected individuals can also develop exocrine pancreatic insufficiency (i.e., malnutrition, diabetes mellitus, pancreatitis), bowel obstruction, sinusitis, and liver disease (i.e., biliary cirrhosis, portal hypertension). The diagnosis is based on compatible clinical findings in at least one organ system (e.g., chronic airway disease, exocrine pancreatic insufficiency, chronic pansinusitis, cystic fibrosis) in a first-degree relative in combination with either biochemical (sweat chloride ≥ 60 mmol/L on two occasions) or genetic confirmation (presence of known disease-causing genetic mutations).
The preoperative clinical evaluation should especially focus on respiratory, hepatic, and nutritional assessment. With respect to preoperative investigations, electrolyte levels, liver function tests, chest radiographs, and PFTs are useful. Collaborative perioperative management in conjunction with a pulmonologist or a cystic fibrosis specialist is ideal, with an important goal being optimization of pulmonary status (i.e., secretions, infections, bronchospasm) before surgery. Most medications for cystic fibrosis should be continued perioperatively.
Postoperative Pulmonary Complications
Postoperative pulmonary complications encompass several important clinical entities and these have been further defined in a recent systematic literature review and consensus-based process. Abbott and colleagues identified four standard outcome measures that are appropriate for widespread use in clinical trials of postoperative pulmonary complications, namely, pneumonia, atelectasis, acute respiratory distress syndrome, and aspiration. In addition, the investigators proposed a new definition of postoperative pulmonary complications that incorporates the severity of the complication based on the degree of therapy required (e.g., supplemental oxygen, positive pressure ventilation). There is considerable variation in how pulmonary outcome complications are defined and this is reflected in the inconsistent reporting of outcomes across studies and hinders interpretation of research findings. Despite the reporting inconsistencies, a reasonable estimate is that these complications develop in about 5% of patients undergoing nonthoracic surgery.
Established risk factors for pulmonary complications pertain to two general domains: patient-level risk factors and procedure-related risk factors ( Box 31.10 ). Patient-level factors include general health status (e.g., ASA physical status [ASA-PS], functional dependency), smoking history, advanced age, COPD, preexisting pulmonary disease (e.g., recent infection, low oxygen saturation) pulmonary hypertension, anemia, heart failure, preexisting sepsis, poor nutritional status (e.g., albumin concentration), and obesity (BMI > 30 kg/m 2 ). Notably absent from this list of patient-related factors are asthma, arterial blood gas results, or PFT results. The risk of complications is surprisingly low in patients who have well-controlled asthma or preoperative corticosteroid treatment. The risk is higher in asthmatic patients with recent exacerbations, prior postoperative pulmonary complications, recent hospitalizations, or endotracheal intubations for asthma. Arterial blood gases are useful in predicting pulmonary function after lung resection operations, but do not estimate perioperative pulmonary risk. Findings on PFTs, such as the degree of FEV 1 , are generally not predictive of pulmonary complications. PFTs have clear roles in diagnosis (“ Is dyspnea caused by lung disease or heart failure? ”) or informing management (“ Can dyspnea or wheezing be improved further? ”), but should not be used as a risk assessment tool or to deny a surgical procedure. Procedure-related risk factors for pulmonary complications include the type of procedure (head-and-neck, thoracic, upper abdominal, aortic, neurosurgical), long-duration procedures, emergency procedures, general anesthesia, and residual neuromuscular blockade.
Potential Patient-related Risk Factor
Advanced age
ASA-PS Class 2 or more
Congestive heart failure
Functionally dependent
Chronic obstructive pulmonary disease
Weight loss
Impaired sensorium
Cigarette use
Alcohol use
Abnormal findings on chest examination
Potential Procedure-related Risk Factor
Aortic aneurysm repair
Thoracic surgery
Abdominal surgery
Upper abdominal surgery
Neurosurgery
Head-and-neck surgery
Emergency surgery
Vascular surgery
General anesthesia
Perioperative transfusion
Potential Laboratory Test Risk Factor
Albumin concentration < 35 g/L
Chest radiograph abnormalities
BUN concentration > 7.5 mmol/L (> 21 mg/dL)
ASA-PS, American Society of Anesthesiologists Physical Status; BUN, blood urea nitrogen.
Several preoperative clinical risk indices have been developed to estimate pulmonary risk. Although these indices have reasonable predictive accuracy, they also have important limitations. Some only predict specific complication types (i.e., pneumonia vs. respiratory failure), and others are too complex for easy clinical use. The most straightforward, currently available index is likely the ARISCAT (Assess Respiratory Risk in Surgical Patients in Catalonia) score, which has also been externally validated ( Table 31.14 ). The score classifies patients as low-risk, intermediate-risk, and high-risk strata.
Components of ARISCAT Score | Points Assigned |
---|---|
Age
| 0 3 16 |
Preoperative oxygen saturation
| 0 8 24 |
Respiratory infection in prior month | 17 |
Preoperative anemia (<100 g/L) | 11 |
Surgical incision location
| 0 15 24 |
Duration of surgery
| 0 16 23 |
Emergency procedure | 8 |
ARISCAT Score | Risk of Pulmonary Complications † |
---|---|
Low-risk: < 26 points | 1.6% |
Intermediate risk: 26–44 points | 13.3% |
High-risk: ≥ 45 points | 42.1% |
∗ Estimates risk of composite endpoint of respiratory infection, respiratory failure, pleural effusion, atelectasis, pneumothorax, bronchospasm, or aspiration pneumonitis.
† Three patients were excluded because of a missing value in some variable.
When high-risk patients are identified before surgery, the anesthesiologist has several available options to help decrease their perioperative pulmonary risk. These approaches include encouraging smoking cessation (see section on “Smokers and Second-Hand Smoke Exposure”), treatment of any recent asthma or COPD exacerbation, and treatment of any recent lower respiratory tract infection. These interventions may require medications (e.g., antibiotics, bronchodilators, corticosteroids), specialist referral (e.g., pulmonologists), or delay in the planned surgical procedure. There is accumulating evidence pointing to benefits from preoperative inspiratory muscle training and physiotherapy in high-risk patients undergoing cardiac or abdominal surgery. The anesthesiologist can also use the preanesthesia evaluation to educate patients about the potential respiratory benefits of neuraxial anesthesia or analgesia, and discuss options for less-invasive surgical procedures with the referring surgeon.
Endocrine Disorders
Diabetes Mellitus
Diabetes mellitus afflicts about 420 million people worldwide. The two main disease categories are type 1 diabetes (previously called “insulin-dependent diabetes” or “juvenile-onset diabetes”) and type 2 diabetes (previously called “non–insulin dependent diabetes” or “adult-onset diabetes”). Type 1 diabetes, which accounts for about 5% to 10% of all cases of the disease, is the result of autoimmune destruction of pancreatic β-cells. Affected individuals have an absolute deficiency of insulin, but normal sensitivity to insulin. Since the disease has a typically early onset at a young age, and is often difficult to control, adults with type 1 diabetes are at risk of premature vascular disease, such as IHD, nephropathy, retinopathy, and peripheral neuropathy. They are also at risk of diabetic ketoacidosis. Type 2 diabetes is characterized by insulin resistance and a relative (but not absolute) deficiency in insulin. Most affected individuals are obese and seldom prone to ketoacidosis. Diabetes mellitus is associated with multiorgan dysfunction, including IHD, heart failure (independent of associated IHD), CVD, CKD, peripheral neuropathy, autonomic neuropathy (e.g., postural hypotension, gastroparesis), retinopathy, and reduced joint mobility (e.g., reduced cervical mobility affecting airway management). In the perioperative setting, diabetes mellitus is a risk factor for postoperative complications, including cardiac events, acute kidney injury (AKI), and surgical site infections. Insulin therapy is the main treatment for type 1 diabetes mellitus, either as multiple daily injections or a continuous subcutaneous insulin infusion. In the case of type 2 diabetes, multiple treatment options are available, including nonpharmacologic therapy (i.e., diet, weight loss, exercise), metformin, sulfonylureas (e.g., glyburide, glipizide), repaglinide, glucagon-like peptide-1 (GLP-1) agonists (e.g., liraglutide), sodium-glucose cotransporter 2 (SGLT2) inhibitors (e.g., empagliflozin), dipeptidyl peptidase-4 (DPP-4) inhibitors (e.g., sitagliptin, saxagliptin, linagliptin, alogliptin), and insulin.
During the preoperative evaluation of a patient with diabetes mellitus, the anesthesiologist should document the disease type (i.e., type 1 vs. type 2), current usual glycemic control, history of hypoglycemic episodes, current therapy, and the severity of any end-organ complications. Given the effects of diabetes mellitus on other organ systems, the history and physical examination should especially focus on the cardiovascular, renal, and neurologic systems. Inquiries about postural dizziness, early satiety, and postprandial vomiting can help assess for any autonomic neuropathy. The physical examination should include an evaluation of pulses, skin breakdown, and joint (especially cervical spine) mobility. Informative preoperative laboratory tests include an ECG and blood sampling for electrolyte, creatinine, and blood glucose concentrations. To help better estimate renal function, an estimated GFR should be calculated (see section on “Kidney Disease”). Since patients are not typically fasting when they are evaluated in a preoperative evaluation clinic, glucose concentrations measured in the clinic cannot be used to evaluate general glycemic control. A diary of multiple glucose values (preprandial and postprandial) at varying times of the day is more informative for estimating the adequacy of therapy. Alternatively, a glycosylated hemoglobin (HbA 1c ) concentration can help characterize the average plasma glucose concentration within the prior 3 months. Among surgical patients, preoperative HbA 1C is more informative than patients’ self-reported history, fasting blood glucose concentrations, and random blood glucose concentrations in identifying preexisting poor glycemic control. In the nonoperative setting, the American Diabetes Association recommends a target HbA 1c concentration under 7% for most diabetic patients. Although preoperative HbA 1c is correlated with postoperative glycemic control, its role as a predictor of postoperative complications is largely restricted to diabetic patients undergoing orthopedic or vascular surgery. Recently updated guidelines from the National Institute for Health and Care Excellence (NICE) in the United Kingdom recommend offering HbA 1c testing to diabetic surgical patients who have not been tested in the prior 3 months, and ensuring the recent HbA 1c test results be included in referral materials from patients’ primary care providers.
In the perioperative setting, the goals of glycemic management are to avoid hypoglycemia, prevent ketoacidosis, and avoid marked hyperglycemia. Tight perioperative glucose control in the immediate perioperative period is controversial. While aggressive management of hyperglycemia may theoretically help decrease postoperative complications, these theoretical clinical benefits of intraoperative intensive glucose control have not been observed in randomized trials of surgical patients. Ideally, all diabetic patients should have their surgery as an early morning case to minimize any disruption of their diabetic management fasting. Normal treatment regimen for most non-insulin diabetic medications (metformin, sulfonylureas, repaglinide, GLP-1 agonists, DPP-4 inhibitors) should be continued until (and inclusive of) the day before surgery but held on the morning of surgery. The possible exception pertains to SGLT2 inhibitors, which have been associated with euglycemic diabetic ketoacidosis in the postoperative setting. Thus, some guidelines recommend that the medications be discontinued at least 24 hours before elective surgery. Diabetic patients should discontinue short-acting insulin while fasting. The exception pertains to patients with continuous subcutaneous insulin infusion pumps. These individuals should continue their infusion at the lowest basal rate, which is usually the nighttime fasting rate. With respect to management of intermediate-acting or long-acting insulin on the day of surgery, there is no uniform consensus on optimal perioperative protocols. A reasonable approach is for patients with type 1 diabetes mellitus to take a small amount (one third to one half) of their usual morning dose of intermediate-acting or long-acting insulin (e.g., lente, isophane) to avoid diabetic ketoacidosis. Patients with type 2 diabetes mellitus can either take no insulin or up to one half of their usual dose of intermediate-acting, long-acting, or combination (e.g., 70/30 preparations) insulin on the morning of surgery.
Thyroid Disease
Thyroid hormones are important for metabolism and its regulation. Mild to moderate thyroid dysfunction probably has minimal perioperative impact. The major concern pertains to significant hyperthyroidism or hypothyroidism, which appears to increase perioperative risk. Symptoms and signs of hypothyroidism and hyperthyroidism can be subtle and nonspecific, especially with milder disease in older adults. Hyperthyroid individuals may manifest tachycardia, arrhythmias, palpitations, tremors, weight loss, and diarrhea. Patients with hypothyroidism may demonstrate hypotension, bradycardia, lethargy, weight gain, depressed cardiac function, pericardial effusions, and impaired ventilatory response to hypoxia or hypercarbia. Patients may also have goiters with related symptoms such as dysphagia, dyspnea, wheezing, and orthopnea. Individuals with hyperthyroidism due to Graves disease may also demonstrate proptosis.
The preoperative evaluation should clarify the patient’s current medical therapy as well as any recent changes. In patients with known thyroid disease, additional preoperative thyroid function testing is not needed if the patient is on a stable medication dose and was assessed as being euthyroid within the previous 6 months. If additional preoperative testing is clinically indicated, thyroid-stimulating hormone (TSH) assays are best to evaluate for hypothyroidism, while free triiodothyronine (T 3 ), free thyroxine (T 4 ), and TSH levels are useful in hyperthyroid patients. Surgery, stress, or illness can precipitate myxedema or thyroid storm in patients with untreated or severe thyroid dysfunction. In general, if a patient has moderate or worse hypothyroidism (i.e., elevated TSH and low free T 4 —with or without associated symptoms), elective surgery should be postponed until the individual is euthyroid. Similarly, elective non-thyroid surgery should also be delayed to facilitate treatment of patients with overt hyperthyroidism (i.e., suppressed TSH with elevated free T 4 or T 3 concentrations—with or without associated symptoms). Consultation with an endocrinologist is necessary if surgery is urgent in patients with thyroid dysfunction. If surgery is urgent, hyperthyroid patients can be treated with β-adrenergic blockers, antithyroid medications (e.g., methimazole, propylthiouracil, potassium iodide), and corticosteroids. Other potentially useful tests include chest radiography or computed tomography scans to evaluate tracheal or mediastinal involvement by a goiter. All thyroid replacement therapy and antithyroid drugs should be continued on the day of surgery.
Parathyroid Disease
Parathyroid hormone regulates calcium. Most cases of hyperparathyroidism are discovered based on an incidental elevated calcium level found during diagnostic testing. Primary hyperparathyroidism is caused by a primary disorder of the parathyroid glands (adenomas or hyperplasia). Secondary hyperparathyroidism is parathyroid gland hyperplasia induced by the hyperphosphatemia and hypocalcemia that occur during chronic renal failure. Tertiary hyperparathyroidism occurs when the parathyroid hyperplasia in secondary hyperparathyroidism functions autonomously. Hypercalcemia from parathyroid disease is associated with osteoporosis and bone loss. It is very unlikely that parathyroid glands become sufficiently enlarged to compromise the airway. Hypoparathyroidism is very uncommon, but it can be the consequence of a prior total parathyroidectomy.
Hypothalamic-Pituitary-Adrenal Disorders
The hypothalamus releases corticotropin-releasing hormone, which regulates adrenocorticotropic hormone (ACTH) release from the anterior pituitary gland. ACTH, in turn, regulates cortisol release from the adrenal cortex. Cortisol secretion varies with the circadian rhythm, with the highest release in the morning. Additionally, release increases with physical stress, psychological stress, fever, and hypoglycemia. Among physical stressors, surgery is one of the most potent activators of the hypothalamic-pituitary-adrenal axis. Although ACTH concentrations increase with surgical incision and through the surgical procedure, the greatest ACTH secretion occurs during termination of anesthesia and the immediate postoperative period. The magnitude and duration of the cortisol response reflects the degree of physiological stress imposed by the surgical stress. In procedures with minimal associated stress (e.g., inguinal hernia repair), increased cortisol secretion lasts for about 24 hours. In more complicated procedures (e.g., major abdominal surgery), the response is larger in magnitude and lasts for about 5 days after surgery.
Excess adrenal hormones result from endogenous cortisol associated with pituitary or adrenal tumors, or exogenous glucocorticoids used to treat disorders such as asthma or inflammatory diseases. Cushing syndrome refers to the combinations and symptoms due to chronic excess glucocorticoid exposure (either endogenous or exogenous). Cushing disease is the specific situation when the excess glucocorticoids are related to an ACTH-producing pituitary tumor. Other causes of Cushing syndrome include exogenous corticosteroids, adrenal tumors, adrenal hyperplasia, and neoplasms that secrete ectopic ACTH. The major manifestations of Cushing syndrome are obesity (with characteristic patterns of fat deposition causing “moon facies” and a “buffalo hump”), diabetes mellitus, female virilization, OSA, hypertension, elevated cardiovascular risk, elevated venous thromboembolism (VTE) risk, osteoporosis, striae, skin atrophy, and easy bruising. Airway management can be challenging in affected patients due to obesity and OSA. In addition, peripheral intravenous access can be difficult because of skin atrophy and obesity. These patients may require an ECG and blood sampling for electrolytes and glucose. Despite easy bruising, they have normal coagulation profiles.
An important issue for patients with chronic corticosteroid exposure is whether perioperative “stress-dose steroids” are needed. Both endogenous and exogenous glucocorticoids exert important negative feedback suppression on the hypothalamic-pituitary-adrenal axis. Thus, chronic exogenous corticosteroid exposure suppresses the adrenals and may blunt the normal cortisol hypersecretion associated with surgery—even if the patient does not demonstrate Cushing syndrome. Perioperative corticosteroid supplementation is needed only when a patient is likely to have suppression of the hypothalamic-pituitary-adrenal axis. Thus, supplementation is not required for individuals who have received less than 5 mg prednisone (or its equivalent) daily, or less than 3 weeks of corticosteroids (regardless of dose). These individuals should simply continue their usual long-term corticosteroid regimen through the perioperative period. Conversely, patients taking prednisone (or its equivalent) in daily doses exceeding 20 mg/day for more than 3 weeks, and patients with Cushing syndrome should have perioperative corticosteroid supplementation. The need for supplementation is unclear for patients who have taken prednisone (or its equivalent) at a daily dose of 5 to 20 mg for more than 3 weeks. The options are to simply empirically provide perioperative corticosteroid supplementation or refer the patient to an endocrinologist for formal evaluation of their hypothalamic-pituitary-adrenal axis. There is no clear consensus on the optimal perioperative corticosteroid supplementation regimen. A proposed regimen that accounts for contemporary evidence and different stress-response profiles across surgical procedures is presented in Table 31.15 .
Surgical Stress | Target Hydrocortisone Equivalent | Preoperative Corticosteroid Dose | Perioperative Corticosteroid Dose |
---|---|---|---|
Superficial procedure (e.g., biopsy, dental procedure) | 8–10 mg/day | Usual daily dose |
|
Minor (e.g., inguinal hernia repair, colonoscopy, hand surgery) | 50 mg/day | Usual daily dose |
|
Moderate (e.g., colon resection, total joint replacement, lower extremity revascularization) | 75–150 mg/day | Usual daily dose |
|
Major (e.g., esophagectomy, pancreatoduodenectomy, major cardiac, major vascular, trauma) | 75–150 mg/day | Usual daily dose |
|
∗ Administer continuous IV fluids with 5% dextrose and 0.2% to 0.45% sodium chloride (based on degree of hypoglycemia).
Patients with adrenal insufficiency have weakness, weight loss, hypotension, orthostasis, hypovolemia, hyperpigmentation, and electrolyte abnormalities. Adrenal insufficiency results from destruction of the pituitary gland, destruction of the adrenal glands (e.g., autoimmune disease, tuberculosis, HIV infection), or long-term exogenous glucocorticoid administration (most common cause). To help establish the diagnosis and cause of adrenal insufficiency, patients require a morning cortisol concentration measurement, morning plasma ACTH concentration measurement, and often an ACTH stimulation test. If the serum cortisol concentration is inappropriately low and a simultaneous plasma ACTH concentration is very high, primary adrenal insufficiency (i.e., primary adrenal disease) is the cause. Secondary (i.e., pituitary disease) or tertiary (i.e., hypothalamic disease) is the diagnosis if both serum cortisol and plasma ACTH concentrations are inappropriately low. Consultation with an endocrinologist is required if formal diagnostic testing for adrenal insufficiency is required, and to facilitate treatment of patients meeting the diagnostic criteria. Patients should continue their replacement corticosteroid therapy on the day of surgery and may need further supplementation based on the expected surgical stress response (see Table 31.15 ).
Importantly, aldosterone, although also produced by the adrenal cortex, is controlled instead by the renin-angiotensin system, not the hypothalamic-pituitary-adrenal axis. Aldosterone regulates volume and electrolytes (absorption of sodium and chloride; secretion of potassium and hydrogen ions).
Multiple Endocrine Neoplasia Syndromes
Multiple endocrine neoplasia (MEN) syndromes are autosomal dominant inherited disorders. There are three types, namely MEN type 1, MEN type 2A, and MEN type 2B ( Box 31.11 ). Although rare (2 in 100,000 for MEN type 1, and 3 in 100,000 for MEN type 2), recognition is important to facilitate treatment of the affected patient and evaluation of family members. MEN type 1 is characterized by the “3 Ps,” namely tumors of the parathyroid glands, anterior pituitary, and pancreatic islet cells. Hyperparathyroidism is the most common manifestation of MEN type 1, with 90% penetrance by the age of 40 years. Affected individuals are also predisposed to other tumors, including gastrinomas (usually in the duodenum), carcinoid tumors (thymus or bronchi), enterochromaffin cell-like gastric tumors, adrenocortical adenomas, and lipomas. Individuals with gastrinomas can develop Zollinger-Ellison syndrome, which is characterized by multiple peptic ulcers due to gastrin hypersecretion. While testing for MEN type 1 gene mutations is possible, there is little evidence that early detection improves the disease prognosis.
Multiple Endocrine Neoplasia Type 1
- 1.
Primary hyperparathyroidism
- 2.
Entero-pancreatic tumor (e.g., gastrinoma, insulinoma, nonfunctioning)
- 3.
Anterior pituitary tumor (e.g., prolactinoma)
- 4
Others
- (a).
Foregut carcinoid tumor (e.g., thymus, gastric enterochromaffin-like tumor)
- (b).
Adrenal cortical tumor (nonfunctioning)
- (c).
Lipomas
- (d).
Facial angiofibromas
- (e).
Collagenomas
- (a).
Multiple Endocrine Neoplasia Type 2A
- 1.
MEN2A classical syndrome (i.e., medullary thyroid cancer, pheochromocytoma, primary hyperparathyroidism)
- 2.
MEN2A with cutaneous lichen amyloidosis
- 3.
MEN2A with Hirschsprung disease
- 4.
Familial medullary thyroid cancer (no pheochromocytoma or parathyroid hyperplasia)
Multiple Endocrine Neoplasia Type 2B
- 1.
Medullary thyroid cancer
- 2.
Pheochromocytoma
- 3.
Others
- (a).
Mucosal neuromas
- (b).
Intestinal ganglioneuromas
- (c).
Marfanoid habitus
- (a).

Full access? Get Clinical Tree
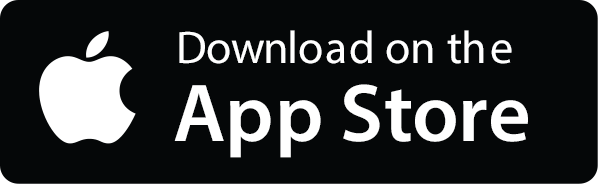
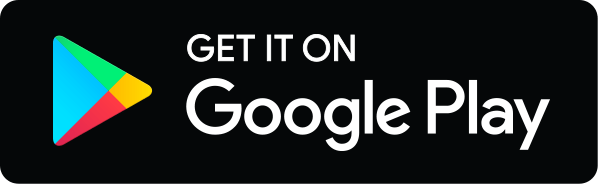
