Critical care, including postoperative care, of the neurologically ill patient involves application of the general critical care principles of management with an added focus on prevention and treatment of secondary brain injury. Systemic and neurologic monitoring is typically required and the knowledge and implementation of emergent, timely intervention is paramount. Neurologic injuries, including traumatic brain injuries (TBI), may incur complications or sequelae , which result from the primary (initial) injury or a secondary (subsequent) injury. The lesion sustained at the time of impact, described as the primary injury, results from the high-energy acceleration or deceleration of the brain within the cranial vault. The secondary injury manifests as a result of a more complex process induced by alterations in cerebral blood flow (CBF), inflammation, hypermetabolism, and tissue necrosis. Sequelae of head injury often correlate with the severity of head injury. The Glasgow Coma Scale (GCS) is a commonly used tool for quantifying the severity of head injury. The GCS score ranges from 3 to 15. Scores of 9 through 15 indicate mild-to-moderate head injury, and a score of 8 or less indicates severe injury. Multisystem sequelae of severe head injury include airway obstruction, respiratory dysfunction, cardiovascular dysfunction, fat embolism syndrome, hematologic abnormalities, neuromuscular dysfunction, metabolic abnormalities, electrolyte imbalances, gastrointestinal abnormalities, immunologic abnormalities, endocrine abnormalities, infectious complications, secondary brain injury, and cerebral hyperperfusion syndrome (CHS). Independent predictors of poor outcome include hypotension, hypoxia, hypoglycemia, hyperthermia, hypocapnia, and intracranial hypertension.
Respiratory complications
Alteration in Ventilatory Control
Spontaneous respirations are controlled by neural centers in the pons (pontine respiratory group [PRG]) and medulla oblongata (dorsal respiratory group [DRG] and ventral respiratory group [VRG]). These centers send impulses down the spinal cord to the motor neurons supplying the respiratory muscles. Efferent impulses from the DRG travel primarily to the inspiratory muscles whereas those from the VRG travel mainly to the expiratory muscles, some inspiratory muscles, as well as muscles of the tongue, pharynx, and larynx. On either side of the medulla between the nucleus ambiguus and the lateral reticular nucleus lie a group of synaptically coupled neurons, which form a complex called the pre-Botzinger complex (pre-BOTC). This pre-BOTC is responsible for the initiation of the rhythmic respirations and is, therefore, also known as the pacemaker of respirations. Chemoreceptors in the medulla respond primarily to changes in pCO 2 and, to a lesser extent, to changes in pH and pO 2 by altering respiratory patterns to compensate for imbalances. These compensatory responses are affected in brain injury leading to hypoxia, hypercapnia, or both. In patients with mild-to-moderate brain injury, the primary response is that of hyperventilation and hypocapnia, which in turn reduces cerebral blood flow causing a relative decrease in the ICP. In contrast, patients with severe brain injury are prone to develop significant hypopnea or even apnea causing a sudden, severe increase in pCO 2 levels. Hypercarbia can cause cerebral vasodilatation, which in turn leads to an increase in the ICP.
Alterations in respiratory patterns are common in patients with brain injury. These include the following:
Cheyne–Stokes pattern : Cheyne–Stokes is a common pattern of cyclical breathing seen in patients with neurologic injuries. It is characterized by repeated crescendo-decrescendo patterns of breathing and includes a period of apnea at the end of each cycle. The level of consciousness also follows this pattern where the patient becomes increasingly alert to nearly awake during the crescendo phase and possibly vocalizing at the peak of this phase. A gradual slowing of respirations follows a waning of consciousness until the patient is completely apneic and unarousable. This pattern of breathing is associated with deep cerebral hemispheric lesions (stroke, TBI, brain tumor), severe metabolic derangements and central chemosensitivity to changes in CO 2 and O 2 tensions. High pCO 2 leads to excessive compensatory hyperventilation, in turn causing decreased pCO 2, which causes apnea, restarting the cycle.
Biot’s (ataxic) pattern : Ataxic breathing refers to an irregular pattern of both rate and rhythm of breathing. It occurs with lesions in the dorsomedial medulla and may be accompanied by hypersensitivity to respiratory depressants and is considered to be a preterminal event.
Apneustic pattern : Pontine injury can result in a long, gasping inspiration followed by an insufficient length of expiration.
Cluster pattern : Cluster breathing is associated with an irregular frequency and amplitude followed by apneic episodes of varying duration. It can occur due to upper medullary and lower pontine lesions, anoxic encephalopathy, Shy–Drager syndrome as well as subarachnoid, cerebellar and brainstem hemorrhagic lesions.
Central hyperventilation pattern : Patients during early periods of brainstem herniation may cause an increase in the rate and depth of respirations resulting in respiratory alkalosis. This must be differentiated from Kussmaul’s respiration, which is described below.
Kussmaul’s pattern : Initially, acidosis prompts rapid, shallow breathing. As acidosis worsens, Kussmaul’s breathing develops, characterized by deep, labored, and gasping breaths. This compensatory overbreathing can result in reduced carbon dioxide tension and reduced cerebral blood flow ( Fig. 23.1 ).
Fig. 23.1
Abnormal respiratory patterns associated with pathologic brain lesions. A, Cheyne–Stokes respiration. B, Central neurogenic hyperventilation. C, Apneustic breathing. D, Cluster breathing. E, Ataxic breathing.
(Adapted from Plum F, Posner JB: Diagnosis of Stupor and Coma, 3rd ed. New York, Oxford University Press, 1980.)
Anatomic Considerations
Respiratory complications can be separated into upper respiratory and lower respiratory tract complications.
Upper respiratory tract (airway) complications are a leading cause of morbidity and mortality in brain-injured patients. In addition, traumatic injuries may cause significant bone injuries such as fractures and displacements that can subsequently lead to bone fragments being embedded in the soft tissues of the airway. Airway dysfunction and edema are relatively common especially following TBI. The patients may lose sensorimotor functional integrity of the airway leading to an increased incidence of aspiration pneumonia. Cranial nerve injuries may further render the patient susceptible to pulmonary aspiration syndrome. Airway protection is ideally managed with endotracheal intubation in patients with extensive brain injury. Injuries involving the cervical spine and/or facial structures may require emergent control of airway via a cricothyrotomy or tracheostomy. Airway edema, secondary to direct trauma or in the postoperative setting, is assessed by performing the “leak test”. The lack of detection of the characteristic audible leak upon deflation of the endotracheal tube cuff signifies the presence of significant tracheal edema. Airway edema is a major problem in the pediatric age group due to the inherent narrowing of the subglottic portion of the airway along with smaller diameter of the airways. Nonetheless, it is always prudent to maintain a secure airway in suspected or documented cases involving edema of the airway until one can establish resolution of this edema. Therapeutic modalities include parenteral steroids, inhaled racemic epinephrine and upright positioning. A helium/oxygen mixture can be used to treat dyspnea due to airway edema following extubation. This mixture generates less resistance than atmospheric air when passing through the narrow airways of the lungs, and thus requires less effort by a patient to breathe in and out of the lungs ( Fig. 23.2 ).


Full access? Get Clinical Tree
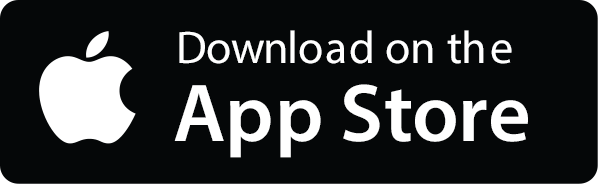
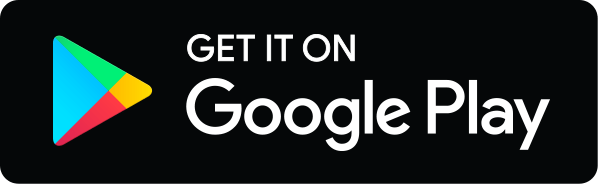
