html xmlns=”http://www.w3.org/1999/xhtml” xmlns:mml=”http://www.w3.org/1998/Math/MathML” xmlns:epub=”http://www.idpf.org/2007/ops”>
A 64-year-old female presented for a total shoulder replacement, which was scheduled to be performed in the beach chair position and last for approximately 3.5 hours. An interscalene catheter was placed preoperatively and surgery proceeds under general anesthesia. When the catheter is discontinued on postoperative day 2 and the patient is noted to have a wrist drop.
Objectives
1. Analyze the incidence of neural deficit after a plexus or peripheral nerve block regional anesthetic.
2. Discuss regional anesthetic factors that may contribute to neurologic deficits and comment on the relation to the site of the block, local anesthetic dose, perineural additives, block needle type, injection pressure, and site of injection (epineural, sub-epineural, perineural).
3. Describe how patient factors impact the incidence of post-block neural deficits.
4. List surgical factors that may impact the development of a postoperative neurologic deficit.
5. Provide a differential diagnosis for nerve deficits following surgery performed under plexus or peripheral nerve block regional anesthesia.
6. Discuss the management of a patient with a postoperative neurologic deficit.
1. Analyze the incidence of neural deficit after a plexus or peripheral nerve block regional anesthetic
Serious permanent nerve injury is a rare event after peripheral nerve block; although minor temporary injuries are surprisingly common, with an incidence ranging up to 7.9% at one month to 0.3% by three months [1–3]. Permanent injury rates, defined as a neurologic abnormality present at or beyond 12 months post-procedure, have consistently ranged from 0.029 to 0.2% [1–2, 4–10]. Ultrasound (US) guidance techniques have not reduced rates compared to nerve stimulation techniques, although a recent multicenter survey found a nerve injury rate of 0.0037% at hospital discharge with US-guided axillary blocks [8, 10–14]. Most long-term reported injuries are multifactorial and more likely caused by non-anesthesia related factors [7]. Compared with single-injection techniques, continuous catheters have not been associated with increased rates of nerve injury [2, 15].
The perioperative nerve injury (PNI) rate associated with total shoulder arthroplasty is 4% under general anesthesia (GA) alone [16]. Despite advances in surgical techniques, this number has not appreciably changed over time. Data from a clinical registry at the Mayo Clinic (1993–2007) demonstrated a PNI rate of 3.7% during GA [17]. This compares to a PNI rate of 1.7% in patients who received a single-injection interscalene block (ISB). Patients who received an ISB had significantly reduced odds for developing PNI [17]. Over 97% of patients with PNI achieved complete or partial recovery at 2.5 years post-procedure, and 71% experienced full recovery. Finally, complete recovery from PNI was no different between patients having received GA only, versus ISB [17].
2. Discuss regional anesthetic factors that may contribute to neurologic deficits and comment on the relation to the site of the block, local anesthetic dose, perineural additives, block needle type, injection pressure, and site of injection (epineural, sub-epineural, perineural)
The connective tissue and adipose layers associated with the nerve become more prominent in cross-sectional volume proceeding from the nerve roots to distal peripheral nerves; hence the nerve is more vulnerable to injury at the root level [18]. Effective interscalene block may be obtained with the block needle tip positioned further away from the nerve roots than previously thought optimal [19].
Anesthetic-related causes of PNI can be divided into needle-related and non-needle-related causes. All local anesthetics (LAs) have inherent neurotoxic and myotoxic properties and cause concentration-dependent reductions in neural blood flow with related neurotoxicity (lidocaine > bupivacaine > ropivacaine) [20–23]. However, there is no clinical evidence to favor one LA over another [24].
Epinephrine is the most commonly administered adjuvant and is used to prolong block duration, decrease peak serum concentrations, and act as a marker for intravascular injections. However, epinephrine potentiates LA-induced reduction in neural blood flow in animal models, when used in a concentration of 1:200,000 (5 µg/ml) [25]. Concentrations of 1:400,000 (2.5 µg/ml) resulted in similar magnitude flow reductions, but with a return to baseline in three minutes. Although there are no human clinical studies demonstrating an increased risk for PNI associated with epinephrine-containing LA solutions, a conservative interpretation of the animal evidence would be to avoid epinephrine in patients at increased risk for PNI.
In an in vitro model, ropivacaine-induced neurotoxicity (0.25%) was not augmented by perineural clonidine or buprenorphine; however, the addition of midazolam or high-dose dexamethasone increased neurotoxicity [23]. Although human clinical studies have not demonstrated neurotoxicity associated with perineural dexamethasone in doses ranging up to 10 mg to date, the in vitro toxicology evidence suggests caution with respect to perineural dexamethasone administration. Further, parenteral dexamethasone may also prolong block duration [26].
It is difficult to penetrate the fascicle of a nerve using a typical short bevel block needle, as the diameter of a 22-gauge block needle is similar to the diameter of the average-sized nerve fascicle [27]. Nerve fascicles move out of the way with needle approach; however, with forceful penetration, larger diameter needles cause more histologic tissue damage than smaller needles [28–29]. Although a Tuohy needle would appear less damaging, there is not a difference in histologic damage with equivalent sized Tuohy or pencil-point block needles [30].
Unintentional intraneural puncture of peripheral nerves, defined as needle tip penetration of the epineurium while remaining outside of the perineum (extra-fascicular), occurs much more frequently than previously thought with paresthesia, nerve-stimulator, and US-guided needle localization techniques [31–35]. Approximately 17% of US-guided needle localization techniques are associated with extra-fascicular intraneural injections, as evidenced by an increase in nerve diameter size during injection, without evidence of long-term neurologic injury [31–32, 34–35]. Although operator errors involving needle-tip visualization are partially to blame for this, a major component is attributed to the limited resolution of current US technology [14]. Intraneural, but extra-fascicular injections of LAs in porcine models, even in volumes as large as 20 ml, were not associated with functional motor injury [36]. However, intra-fascicular injections in animal models resulted in extensive axonal damage [37–38]. Additionally, injections around the roots of the brachial plexus during a US-guided ISB, with observable increase in nerve root diameter but without accompanying pain or paresthesia, led to a severe, long-term neurologic injury [39].
Based on the collective evidence, a cautious approach would be to avoid deliberate intraneural injection. A test injection (≤0.5 ml) of LA solution has demonstrated 84 to 94% sensitivity in detecting intraneural swelling using US, although post-injection histologic analysis could not determine if swelling was due to extra-fascicular or intra-fascicular injection [40–41]. While nerve stimulation has a sensitivity of approximately 70% in detecting needle-to-nerve contact, it is highly specific for intraneural needle placement when the current is less than or equal to 0.2 mA. Therefore, stimulation may provide additional safety information or indication for needle withdrawal prior to injection [42].
Needle contact with the epineurium also raises the possibility of lacerating the external vascular supply, the vasa nevorum, potentially creating an external compressive hematoma. Needle penetration through the epineurium may injure sub-epineural blood vessels causing intraneural hematoma with similar catastrophic consequences. A cautious US-guided needle localization technique, including hydrolocation and hydrodissection, can help identify the needle tip and decrease direct needle–nerve contact.
Injection-pressure monitoring is a new guidance modality. Opening pressures greater than 15 psi were sensitive for detecting needle–nerve contact but required use of an automated pump; thus limiting clinical applicability [43]. The future role of triple guidance, including the combination of US, nerve stimulation, and injection-pressure monitoring, in order to reduce the incidence of inadvertent intraneural injection and potential PNI is yet to be determined.
3. Describe how patient factors impact the incidence of post-block neural deficits
Many surgical patients have undiagnosed, subclinical neurologic injury, which may manifest as clinical postoperative neurologic deficits. Surgical- and anesthesia-related factors may not be severe enough to induce clinically apparent injury on their own. However, when combined with a nerve injured prior to surgery, they may result in postoperative deficits. This is the basis of the double-crush theory of nerve injury [44]. Patients presenting with an undocumented pre-existing neurologic injury may cause confusion about PNI etiology when this is first documented postoperatively.
In the largest database observational study to date examining the incidence of postoperative nerve injury involving 380,680 anesthetics, patient-related risk factors that independently predicted the risk of developing a postoperative nerve injury included the presence of diabetes, hypertension, or tobacco use [6]. Notably, all of these conditions involve microangiopathic changes that may reduce nerve perfusion and increase the risk of ischemia. Other patient-related factors include pre-existing neurologic conditions with a waxing and waning course (e.g., multiple sclerosis or post-polio syndrome) and previous exposure to radiation or chemotherapeutic agents (e.g., vincristine), which may lower the threshold for further injury [45].
4. List surgical factors that may impact the development of a postoperative neurologic deficit
The most common surgical factors contributing to postoperative neurologic deficit development include stretch and compression of the brachial plexus, lumbosacral plexus, or relevant peripheral nerves. The brachial plexus is at risk for stretch-related injuries during shoulder surgery as a direct result of positioning required for the surgical procedure. Notably, the beach chair position is associated with a lower risk of positioning injuries than the lateral decubitus position. Nerves are particularly intolerant of stretch, with significant ischemia developing with an 8% increase in elongation and complete arrest of flow at 15% [46]. Other causes of surgically induced nerve injury include compression of nerves by retractors or tourniquets resulting in ischemia; surgically induced edema creating nerve compression and ischemia; hematoma formation externally compressing nerves; direct nerve contusion or transection from blunt or sharp dissection instruments; and postsurgical inflammatory neuropathy [47].
5. Provide a differential diagnosis for nerve deficits following surgery performed under plexus or peripheral nerve block regional anesthesia
The most common injuries involve the upper and middle trunks, and musculocutaneous, suprascapular, and axillary nerves [16, 46, 48–49]. A useful way to categorize PNI is to subdivide this into surgery-related, block-related, or patient-related causes [3]. Once the injury site is localized by electrodiagnostic studies (i.e., motor and sensory nerve conduction studies and electromyography), a clinical correlation can be made with a specific injury mechanism at that site, such as tourniquet, surgical incision, block needle, or patient-related factors [50–52].
Electrodiagnostic studies can determine if the injury is primarily a demyelinating process, which is associated with an excellent prognosis for a full recovery, or an axonal injury process, which has a mixed prognosis and a longer recovery period. A demyelination injury is characterized primarily by conduction slowing and increased latency on motor and sensory nerve studies. Axonal injury is identified by a loss of amplitude with minimal latency changes [51]. Needle electromyography (EMG) can confirm axonal injury, location, and timing. This is done by detecting the presence or absence of fibrillation potentials, which are unique to axonal injury, in muscles innervated by the affected nerve roots of the injured nerve or plexus [51]. Additionally, needle EMG can detect a previously undiagnosed related nerve injury that is in the process of recovery, with signature morphologic patterns in the motor unit action potentials (MUAP) consisting of polyphasic, extended-duration MUAPs with satellite potentials [50–52].
6. Discuss the management of a patient with a postoperative neurologic deficit
It is important to examine the patient and document the injury immediately and rule out a treatable cause, such as hematoma or other mass effect. This can be done with physical examination and studies such as US or magnetic resonance imaging (MRI)/magnetic resonance neurography (MRN). While purely sensory deficits can be managed conservatively with multimodal neuropathic analgesics and observed, any motor weakness is a serious injury and warrants immediate neurological consultation.
Baseline electrodiagnostic studies should be obtained to detect a previously unknown or prior subclinical injury [50–52]. These studies should be repeated at one month in order to detect onset of a new acute injury, as well as to provide information regarding the location, severity, and ultimate prognosis for recovery. One month after an injury is diagnosed; electrodiagnostic studies can determine the injury type, a demyelinating injury (neurapraxic) with an excellent prognosis or an axonal injury (axonotmetic), which is associated with mixed prognosis. Electrodiagnostic studies can also determine whether or not a nerve transection injury (neurotmetic) has occurred, which if present, will require referral for reconstructive neurosurgery for potential nerve grafting, nerve transfers, or tendon transfers [53–54]. If there is no significant improvement in function, electrodiagnostic studies should be repeated at three months and six months. By six to nine months post-injury, the option of reconstructive nerve grafts or transfers should be considered, as the muscle fibers and neuromuscular junctions will irretrievably degenerate without intervention. Unfortunately, there is no neuropharmacologic therapy currently available to stimulate peripheral nerve regeneration.

Full access? Get Clinical Tree
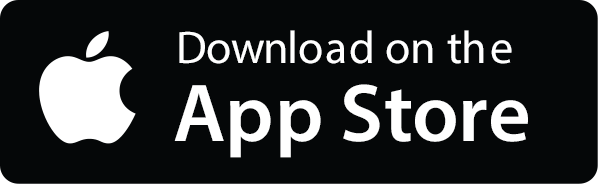
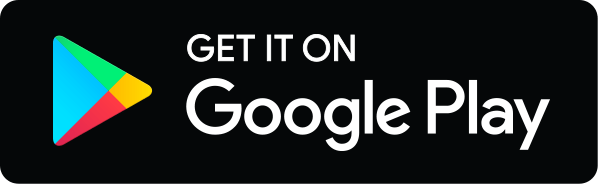