Chapter 21
Positioning for Anesthesia and Surgery
Physiologic Effects of Surgical Positions
Cardiovascular System
Hemodynamic changes are usually minimal in the supine and lateral positions.1,2 However, cardiac output and blood pressure are often decreased in the sitting, prone, and flexed lateral positions, where the lower extremities are dependent.1,3 Although central venous pressure (CVP) is increased in the prone position, left ventricular volume is reduced, probably due to decreased venous return and increased intrathoracic pressure.4 Cardiac index (CI) may be decreased5,6 or unchanged5 in the prone position compared with the supine; the effect may be frame dependent.6 In the lateral decubitus position with the kidney rest elevated, hypotension is likely because the legs are dependent, venous return is reduced by extreme flexion, and the kidney rest may compress the great vessels.1,3 Conversely, blood pressure may appear normal or higher in the lithotomy position, in which elevation of the legs above the trunk provides an autotransfusion of 100 to 250 mL per leg.
Mean arterial pressure increases or decreases by approximately 2 mmHg per inch for each change in height between the heart and a body region.7 Therefore regions elevated above the heart in the head-up, sitting, and lithotomy positions may be at risk for hypoperfusion and ischemia, particularly if hypotension occurs. The decrease in hemodynamic parameters depends on the degree of elevation of the torso. Hemodynamic changes are minimal if the patient is placed in a 45-degree, head-up sitting position, but cardiac output decreases 20% if the patient is raised to 90 degrees, because venous blood pools in the extremities. When the patient is in the seated position, as compared with supine, CI, CVP, and pulmonary capillary wedge pressure (PCWP) decrease significantly and SVR increases.8 In procedures in which the head is elevated and cerebral perfusion is a concern, invasive arterial blood pressure monitoring should be instituted, with the transducer placed at the level of the circle of Willis.9
Positioning devices and mechanical ventilation may contribute to decreased cardiac output and hypotension. In the lateral decubitus position, elevation of the kidney rest under the flank may cause vena cava compression. When the patient is in the lateral decubitus position, the kidney rest should lie under the dependent iliac crest.1 Extreme flexion of the hips in some variations of the prone or lithotomy positions may occlude the femoral vessels and contribute to decreased venous return. Large tidal volumes and positive end-expiratory pressure (PEEP) may generate high intrathoracic pressures, with a subsequent reduction in atrial filling and cardiac output.
A variety of methods have been suggested for attenuating the hemodynamic changes associated with surgical positioning. Slow assumption of the surgical position allows the cardiovascular system time to compensate for position-induced hemodynamic alterations. Because hemodynamic changes may be influenced by anesthetic technique, using a nitrous-narcotic technique or a lighter level of anesthesia (less than 0.5 minimum alveolar concentration) or gradually attaining a deeper level of anesthesia may attenuate position-induced hypotension.3 Intravascular volume loading before positioning can reduce or eliminate hypotension.1,6 However, volume replacement must be done judiciously because excessive fluid administration can lead to volume overload in susceptible individuals when the patient is returned to the supine position, or when the vasodilatory effects of general anesthetics are terminated.3
The Trendelenburg position is often used to treat hypotension, because it is assumed to increase venous return and MAP. When placed in a head-down position, normotensive individuals compensate for increases in CVP and pulmonary artery pressure (PAP) with vasodilation and a decrease in heart rate from stimulation of baroreceptor reflexes. However, hypotensive individuals may not respond in the same manner. Investigators have demonstrated variable effects of the Trendelenburg position on cardiovascular parameters. Changes in intrathoracic blood volume of 2% to 3% in unanesthetized normovolemic individuals are reported with the Trendelenburg position.10 CVP, mean PAP, and pulmonary artery occlusion pressure can be increased in the head-down position, but this increase may not reflect changes in CI, stroke volume, or MAP.10,11 Others have shown no increase in MAP, an increase in SVR, and a decrease in CI in hypotensive patients placed in Trendelenburg position.12
Patients with comorbidities may be susceptible to the detrimental effects of various positions. The combination of the lithotomy position and a head-down tilt can have a detrimental effect on myocardial function in patients with coronary artery disease, because CVP, PAP, and PCWP are increased, whereas cardiac output is decreased.13 The Trendelenburg position may increase myocardial work by increasing central blood volume, cardiac output, and stroke volume. Individuals with very poor cardiac function can have decreased cardiac output if the increased central blood volume moves them to a worse position on the Frank-Starling curve. The lower extremities of individuals with peripheral vascular disease may be at risk of ischemia in the lithotomy and Trendelenburg positions because a relative state of hypoperfusion exists when the lower extremities are elevated above the heart.
The prone and Trendelenburg positions may increase venous pressure in the head, with resultant swelling of facial, pharyngeal, and orbital structures. Intracranial pressure can be elevated when the head is dependent, because venous pressures are transmitted to the head and intracranial structures through the valveless jugular system. Cerebral blood flow can be decreased when inflow is limited by venous congestion in intracranial structures. Postoperative visual loss (POVL) may result from an increase in ocular venous pressures and concomitant decrease in ocular perfusion pressure. Facial edema, macroglossia, and airway edema are reported following the prone and head-down positions.14 A 10-degree head-up tilt may prevent the development of facial edema.
Respiratory System
Postural changes may significantly alter compliance, lung volumes, and the distribution of ventilation and pulmonary blood flow. Positioning devices may cause mechanical interference with movement of the belly wall and abdominal contents, the chest wall, or the diaphragm.15 Therefore anesthetic-induced depression of ventilation may be worsened by the majority of surgical positions. Individuals with preexisting diseases that alter respiratory function may be more susceptible to the deleterious ventilatory effects of surgical positions.
Effective respiratory gas exchange depends on a balance of ventilation and perfusion throughout the lungs. Traditionally, gravitational effects on gas and blood flow are thought to result in differences in ventilation and perfusion in different lung segments. In both awake and anesthetized patients, gravitational forces are theorized to create a gradient that favors perfusion in dependent portions of the lungs and ventilation in nondependent regions.16,17 However, new imaging techniques have identified a concentric pattern of blood flow in the lungs, with central regions receiving a greater proportion of flow than the periphery.18,19 The mechanism for this gradient has not been identified, but the diameters and branching patterns of pulmonary vessels and the distance blood must flow to reach a site are possible factors. Nongravitational factors such as cardiac output, pleural pressures, and lung volumes are also thought to play a factor in regional lung perfusion.16,19
Positional changes may result in redistribution of ventilation and perfusion. These changes are less in the sitting position and more evident in the prone and lateral positions. In the prone position, changes in ventilation-perfusion () ratios have been postulated as the cause of improved oxygenation.16 More lung volume is present posteriorly than anteriorly, where anterior mediastinal structures occupy significant space; as a consequence, posterior lung segments are better ventilated. Ventilation is more uniform and
matching is better in the prone position than in the supine position.17 In the lateral decubitus position, ventilation and perfusion are greater in the dependent lung than in the nondependent lung in awake, spontaneously breathing patients.19
mismatching in the lateral decubitus position may affect oxygenation, especially with procedures requiring one-lung ventilation. Hypoxic pulmonary vasoconstriction in the unventilated lung further redistributes blood flow to the dependent lung to improve oxygenation.19 Patients are susceptible to atelectasis in the lateral position, because closing volumes occur above functional residual capacity (FRC), with closing occurring earlier in the dependent than in the nondependent lung. Tidal volumes of 10 to 12 mL/kg and an Fio2 of 0.5 or higher have been suggested to compensate for
mismatch in the lateral position; however, excessive tidal volumes can cause barotrauma, decrease hypoxic vasoconstriction, and reduce oxygenation in patients in the lateral position. Tidal volumes of 5 to 7 mL/kg and higher respiratory rates cause smaller declines in oxygenation. Although the application of PEEP to the dependent lung is sometimes used in an attempt to recruit collapsed alveoli and increase compliance, controversy exists regarding its effectiveness in improving patient oxygenation. The administration of oxygen at 2 to 4 L/min to the nonventilated lung may improve hypoxia. Application of 5 to 10 cm H2O of continuous positive airway pressure (CPAP) to the nondependent lung can significantly improve oxygenation during one-lung ventilation, but the re-expansion of lung tissue may impede surgical access.19
Changes in the elastance and resistance of the diaphragm and abdomen occur when shifting between positions. These changes have little effect on movement of the chest wall in healthy individuals but may have an effect in persons with conditions that predispose to abnormalities of lung function.20 In the prone position, diaphragmatic excursion can be limited by the abdominal viscera if the abdomen is compressed by the weight of the body or positioning devices. If the abdomen hangs free, gravity allows the abdominal contents to shift, reducing interference with diaphragmatic movement.21,22 In the anesthetized patient in the lateral position, abdominal contents shift cephalad, moving the hemidiaphragm of the dependent lung upward, thereby decreasing ventilation in the dependent lung and reducing its compliance. In the nondependent lung of the anesthetized patient, ventilation is greater and compliance increased due to the caudal shift of the upper hemidiaphragm allowing unrestricted lung excursion (see Chapter 27). The lithotomy position has little effect on the compliance of the respiratory system in healthy, conscious volunteers.15 However, extreme flexion of the thighs in the exaggerated lithotomy position compresses the abdomen, shifts the abdominal viscera cephalad, and limits diaphragmatic movement. As a result, compliance and tidal volume are reduced, and airway pressures and dead space–to–tidal volume ratios are increased.23 This effect may be amplified in obese individuals.
Lung capacities are decreased with most position changes. In the supine position, FRC and total lung capacity are significantly decreased compared with the sitting position due to the cephalad shift of the diaphragm caused by pressure of the abdominal viscera.15,20 Some investigators have found an increase in FRC with patients in the prone position, when the abdomen hangs free.16,24 Theories pose that better matching of ventilation and perfusion, rather than changes in lung volumes or capacities, cause improvements in oxygenation in the prone position.15
In the awake patient in the lateral position, ventilation favors the dependent lung during spontaneous respiration. With the addition of anesthesia, positive pressure ventilation, and paralysis, the upper lung becomes easier to ventilate. Displacement of the relaxed diaphragm by abdominal viscera and the downward gravity force of the mediastinum result in decreased compliance of the lower lung.19–21
Ventilatory changes associated with the lithotomy position are dependent on the extent to which the legs are flexed on the abdomen and the concomitant use of the head-down position. In the normal lithotomy position without Trendelenburg, changes in FRC are similar to those associated with the supine position, and the diaphragm does not shift farther cephalad.23 However, adding Trendelenburg position to lithotomy can cause an additional decrease in FRC.
The sitting position is more favorable for ventilation and has less effect on lung volumes than other positions. The more the torso is elevated, the smaller the effect on lung mechanics. Forced vital capacity and FRC are within normal parameters in the seated position.21 The abdominal contents shift caudally and anteriorly, causing less interference with diaphragmatic movement and allowing greater expansion of dependent lung regions.15 Compared with the supine position, in which the abdominal muscles are used for breathing, in the sitting position, the rib cage contributes more to ventilation.21 However, respiratory benefits of the sitting position are attenuated when the sitting position is modified to minimize cardiovascular effects. Flexion of the lower extremities at the hip and elevation of the legs causes abdominal contents to shift cephalad against the diaphragm. The sitting position then more closely resembles the supine position, limiting diaphragmatic excursion and decreasing FRC and closing volumes.9
The Trendelenburg position exacerbates the deleterious ventilatory effects of the various positions. The diaphragm is displaced cephalad, and its excursion is limited by shifting of the abdominal contents, decreasing the FRC progressively as the degree of Trendelenburg position increases. Movement of the mediastinum toward the head may result in the endotracheal tube migrating into the right mainstem bronchus.25,26 This complication may also occur upon establishment of the pneumoperitoneum, in which the diaphragm is displaced in a cephalad direction by pressurized gas.26,27
Pathophysiology of Nerve Injury
Transection, compression, stretch, and kinking are the primary mechanisms responsible for nerve injuries.28,29 Nerves may be transected by surgical maneuvers or by trauma. Compression can happen when a nerve is forced against a bony prominence or a hard surface such as an armboard or operating table. In the lateral position, for example, the weight of the superior leg pushes against the dependent extremity and may compress the common peroneal nerve of the dependent leg against the operating table. Stretch or traction injuries occur where nerves such as the sciatic nerve or brachial plexus have a long course across many structures. Peripheral nerves have some laxity that allows a limited amount of elongation. However, excessive elongation or stretch may cause conduction changes, axonal disruption, or interruption of the nerve’s vascular supply.30–32 Kinking injuries happen when a peripheral nerve is pinched between two immovable structures. For example, the femoral nerve can be kinked under the inguinal ligament when the thighs are flexed on the abdomen, as in the exaggerated lithotomy position.
A common component of all peripheral nerve injuries is ischemia. Intraneural blood flow may be compromised by stretch, compression, or disruption of the nerve tissue itself.33 Other causes include occlusion of major vessels, emboli, tissue edema, or inhibition of perfusion at the capillary level. For example, pressure applied over a body surface may limit venous capillary outflow, causing a rise in venous capillary pressure and a decrease in the hydrostatic pressure gradient between interstitial tissues and the capillary.34 Ultimately, tissue edema occurs as fluid is sequestered in the cells and interstitial space. As venous capillary pressure rises, the arterial-venous pressure gradient is reduced, decreasing flow to tissues along the capillary. As venous and tissue pressures continue to rise, arterial inflow is eventually obstructed and ischemia results. Low mean arterial blood pressure may augment the development of ischemic conditions.31
Tissue metabolism continues even in the absence of blood flow. When ischemia ensues, adenosine triphosphate (ATP) production is decreased, causing failure of the transmembrane sodium-potassium pump and accumulation of sodium within the cell. The resulting osmotic pressure gradient favors the movement of water into the cells. Intracellular volume is increased, and tissue edema occurs.35 A vicious cycle of ischemia results as tissue pressures increase, preventing the movement of fluid and nutrients from the capillaries into the cells.
The susceptibility of peripheral nerves to ischemia may be partially due to their anatomic structure (Figure 21-1). Peripheral nerves are composed of bundles of nerve fibers (fascicles) and their vascular supply is encased in protective connective-tissue coverings. Each nerve fiber is composed of one or more axons sheathed by Schwann cells (neurolemma) that are either myelinated or unmyelinated. The axons and neurolemma are covered by a loose connective tissue called the endoneurium. The perineurium is a tough connective tissue that binds the fascicles into identifiable structures. The epineurium consists of two layers: an inner epineurium that supports the fascicles and an outer epineurium that covers the external surface of the nerve.31,36 The quantity of these protective tissues varies between nerves and even along the same nerve. The entire nerve trunk is covered by a loose layer of connective tissue that allows it to slide across joints and other tissues.31
Peripheral nerves have an extensive microvascular supply. Blood vessels in the epineurium run parallel to the nerve and form numerous anastomoses with the perineurium. Collateral connections between the perineurium and the endoneurial capillaries run obliquely between the layers and thus may be more susceptible to compression by increases in tissue pressure. In addition, the endoneurial space lacks lymphatic vessels, so edema and fluid accumulation in this region may obstruct the microcirculation.31
Factors Contributing to Nerve Injuries
Postoperative peripheral nerve injuries are frequently attributed to incorrect surgical positioning; however, current evidence indicates that multiple factors are involved in postoperative peripheral neuropathies.37,38 Perioperative factors that contribute to the development of nerve injuries include ancillary positioning devices, prolonged surgical procedures, and anesthetic technique. Patient-related factors include gender, age, body habitus, and preexisting medical conditions. However, the precise mechanism of nerve injury is often unclear, suggesting that further investigation is needed to identify causative factors.37,39
Positioning Devices
Controlled studies of complications related to specific positioning devices are largely nonexistent. Isolated case reports provide much of the evidence that is known about nerve injuries attributable to improper use of positioning devices. Ancillary positioning devices, such as straps used to restrain the patient or an extremity, may cause pressure and temporary injury if excessively tightened. For example, the lateral femoral cutaneous nerve in the thigh is susceptible to injury by tight table straps or the leg-holding device used for knee arthroscopy.40 Common peroneal nerve injury has been attributed to the use of candy cane stirrups. Brachial plexus injury has been caused by a damaged armboard falling off the operating room (OR) table41 or the use of shoulder braces with steep Trendelenburg position.37 Compression injury of the radial nerve has been reported after the intraoperative use of tourniquets and blood pressure cuffs and by compression between the humerus and a firm surface, such as a positioning device.42,43 Improper placement of an axillary roll may cause compartment syndrome and compression of neural and vascular structures.44
Length of Procedure
Prolonged surgical procedures contribute to postoperative positioning complications. Postoperative visual loss, nerve injuries, and compartment syndrome have been associated with a variety of procedures and positions in which the common denominator was a duration of more than 4 hours.45–48 Rhabdomyolysis and acute renal failure also have been reported after lengthy procedures.49,50 One possible explanation is that during long procedures, the weight of the body causes external compression of dependent tissues and states of low perfusion.50 The longer this situation persists, the higher the potential for development of edema and ischemic injury.
Anesthetic Techniques
Anesthetic techniques may contribute to the development of position-related injuries.51 Patients receiving general anesthesia cannot move in response to painful stimuli generated by uncomfortable body positions. The constraints of the procedure or surgeon may limit movement even when patients are sedated. Muscle relaxation due to neuromuscular blocking drugs or volatile anesthetics may contribute to stretch injuries by allowing increased mobility of joints.30 For example, limited elbow extension from tight biceps muscles can be overcome by neuromuscular blockade, allowing the arms to be extended flat and subsequently stretching the median nerve.31 The hypotensive effects of general anesthetics may lower perfusion pressures below acceptable levels in patients who are hypertensive or have other comorbidities. The use of hypotensive techniques to reduce blood loss should be balanced against the risk of possible complications resulting from decreased perfusion pressures, particularly during prolonged procedures and in the sitting, lithotomy, and Trendelenburg positions, in which gravity affects blood flow.
Although neuraxial and peripheral nerve blocks are associated with both permanent and temporary nerve injuries, the majority of injuries are not related to positioning but to block technique, hematoma formation, and needle trauma.52 However, recognition of compartment syndrome may be delayed when providers attribute the patient’s symptoms to the residual effects of regional blocks and local anesthetics.53,54 Anesthetists must have a high index of suspicion when return of function is delayed beyond what is expected for a particular technique or local anesthetic and when patients complain of severe pain in the presence of a seemingly adequate block.
Patient-Related Factors Contributing to Nerve Injuries
Extremes of body habitus are correlated with an increased incidence of positioning complications.47,49,50 Individuals who are underweight may develop decubiti or nerve damage due to lack of adequate adipose tissue over bony prominences.47,55 For example, thin patients may be at higher risk for sciatic nerve damage when the opposite buttock is elevated,28 and thinner women (body mass index [BMI] less than 22) are more likely to develop ulnar neuropathy.52,55 Individuals with a muscular physique may also be at increased risk for compartment syndrome and ulnar nerve injury.
Obesity increases morbidity from positioning because large tissue masses place increased pressure on dependent body parts. Extreme abduction of the arms may cause stretch injuries to the lower roots of the brachial plexus, whereas the upper roots may be damaged by excessive rotation of the head to the opposite side. Ulnar neuropathy has been associated with an increased BMI.56 Adipose tissue is poorly perfused and may contribute to this problem. For example, in the lateral position, a heavy superior extremity may interfere with perfusion by exerting substantial pressure on the inferior extremity.
Preexisting Conditions
Preexisting conditions appear to be associated with an increased risk of developing postoperative position-related injuries. Hypertension, diabetes mellitus, peripheral vascular disease, peripheral neuropathies, and alcoholism can exacerbate the physiologic effects of various positions. Nerve injury and preexisting neuropathies are more common in patients with diabetes,28 and diabetes is the most common metabolic cause of spontaneous isolated femoral neuropathy.57 A history of smoking within 1 month of the surgical procedure has been identified as a risk factor for nerve injury, as well as for delayed healing.47,58 Individuals with subclinical ulnar nerve entrapment, which may not be apparent to the patient or anesthetist, are also at risk for nerve injuries.59,60
Box 21-1 highlights factors associated with position-related injuries.
Perioperative Neuropathies
In the awake patient, pain or discomfort from extreme body positioning would prompt optimization of position for the relief of symptoms. The induction of anesthesia renders a patient unable to make these changes and therefore susceptible to injury. The addition of muscle relaxants may allow body or extremity positioning that the awake patient would not otherwise tolerate, making the patient even more vulnerable to injury.33 Table 21-1 and Box 21-2 identify specific nerve injuries associated with positioning and recommendations for prevention.
TABLE 21-1
Potential Position-Related Injuries
Body System or Anatomic Location | Potential Injury |
Head, eyes, ears, nose, and throat | Postoperative vision loss Corneal abrasion Facial edema Vocal cord edema |
Cardiovascular | Vascular occlusion Deep vein thrombosis Ischemic injuries |
Respiratory | Atelectasis Endobronchial intubation |
Neurologic | Peripheral neuropathy Quadriplegia Decreased cerebral blood flow Increased intracranial pressure |
Genitourinary | Myoglobinuria Acute renal failure |
Musculoskeletal | Amputation Backache Compartment syndrome Rhabdomyolysis |
Integumentary | Abrasion Alopecia Decubiti |
Ulnar Neuropathy
Ulnar neuropathy is one of the most frequently reported injuries after surgery and anesthesia, with a reported incidence ranging from 0.04% to 0.5%.29,59,61 Ulnar neuropathy is a well-known complication of cardiac surgery, with a prevalence as high as 38%.62 The American Society of Anesthesiologists (ASA) and American Association of Nurse Anesthetists Foundation (AANA-F) closed-claims studies found that the ulnar nerve was the first (28%) and second (16%) most commonly injured nerve.61,63,64
The ulnar nerve traverses the length of the upper extremity from its origins as a branch of the medial cord of the brachial plexus to its terminal branches in the hand. In the upper arm, the ulnar nerve passes along the anterior aspect of the medial head of the triceps muscle and posterior into the groove between the medial epicondyle of the humerus and the olecranon (Figure 21-2). In this region, the nerve is sheathed in the cubital tunnel before exiting and passing between the two heads of the flexor carpi ulnaris. The cubital tunnel retinaculum (CTR) forms the roof of the cubital tunnel (see Figure 21-2), a potential area for nerve compression because fibrous tissue and the elbow capsule form a semirigid canal that changes shape with flexion and extension of the forearm.47 When the elbow is flexed, the distance between the olecranon and medial epicondyle increases, stretching the CTR, decreasing the size of the tunnel, and increasing pressure on the nerve.29
Surgical positioning, age, preexisting diseases, and mechanical factors such as tourniquets and blood pressure cuffs have all been proposed as contributing to ulnar nerve injuries. However, ulnar neuropathy is more frequently associated with male gender,33,55,59 the presence of a preexisting asymptomatic neuropathy,59 prolonged hospital stays,33,59 and extremes of body habitus.33,52 Median sternotomy and sternal retraction are also proposed as a cause of ulnar nerve injury after cardiac surgery.65

Full access? Get Clinical Tree
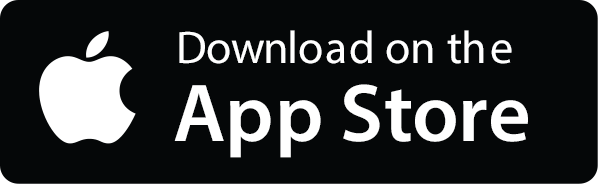
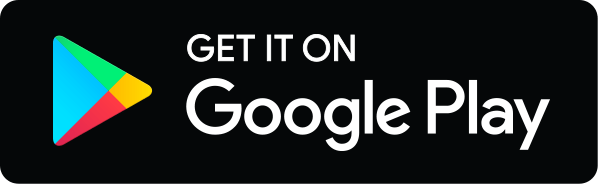