
Hemostasis and History
The term hemostasis essentially means to stop bleeding and refers to the physiologic process that keeps blood within damaged blood vessels, the opposite of hemorrhage. Multiple mechanisms are involved in hemostasis, a process critical for survival. The elucidation of models to explain the molecular and cellular interactions of the clotting cascade continue to evolve over time. The best understood clotting cascade is the Waterfall/Cascade model that most clinicians learned in medical school and was developed about 50 years ago but is still used as an educational tool.1 However, this acellular model does not tell the entire story and has been further refined by Hoffman and Monroe2 in their cell-based model that focuses on both cellular and humoral interactions and (see Fig. 27-1). Hemostasis is also a complex inflammatory response that provides host defense mechanisms to prevent exsanguination following injury, trauma, and/or surgery. Many of the hemostatic factors have complex inflammatory signaling properties that orchestrate further host defense mechanisms, healing, and a multitude of other functions. There are multiple aspects of the physiology of hemostasis and clot formation that will be considered separately.
Initiation of Coagulation
Initiation of coagulation by procoagulant activities has been traditionally separated into extrinsic, intrinsic, and common pathways. However, a better understanding of the complex interactions has created a better conceptual integration of these pathways. Following tissue injury and vascular endothelial disruption, activation of hemostasis occurs by tissue factor (TF) expression on the subendothelial vascular basement of the blood vessel as shown in Figures 27-1 and 27-2.1–3 TF is a transmembrane receptor expressed by perivascular/vascular cells that binds factor VIIa.4 Vascular injury with loss of normal endothelial function allows for expression of extravascular TF and initiation of clotting.4 TF is also present in the circulation as microparticles that are small membrane vesicles that appear following cellular injury or death and may contribute to thrombosis with sepsis or other procoagulant states. Activated factor VII (factor VIIa), a serine protease that circulates in blood in low concentrations, allows for formation of the factor VIIa/TF complex, and conversion of factor X to factor Xa.1,3 Subsequently, factor Xa (also a serine protease) generates trace amounts (0.1–1 nM) of thrombin.1,3 Thrombin generation is subsequently amplified by other coagulation factors from the intrinsic cascade that includes factors XI, IX, and VIII dependent activities, although both the extrinsic (factor VIIa/TF) and intrinsic (factors IXa/VIIIa) tenase complexes produce factor Xa, which is also an important target form many anticoagulation agents. “Tenase” is a contraction of the words “ten” and the suffix “-ase” and refers to these factor complexes which activate inactive factor X through enzymatic cleavage. Multiple factors influence the degree of activation including the local TF concentration and type of cell surface supporting enzyme/cofactor complex assembly as platelets also will contribute to this response.1,3 The interaction of both cellular and plasma-dependent mechanisms generates the prothrombinase complex (factors Xa, Va, and prothrombin) assembly that enzymatically cleaves prothrombin to produce thrombin, another critical factor targeted in anticoagulation therapy. The orchestration of hemostasis and factors influencing its balance are shown in Figures 27-1 and 27-2.
As part of the activation, there are also checks and balances in the system to prevent an over exuberant prothrombotic effect from occurring and regulate thrombin generation to localize clot at the site of vascular injury as shown in Figure 27-2. Tissue factor pathway inhibitor (TFPI) neutralizes factor Xa when it is in a complex with TF-factor VIIa.1,3 The other regulator of TF-trigger procoagulant response is antithrombin (AT, formerly called antithrombin III; a serine protease inhibitor; SERPIN), which circulates at a high concentration (150 µg/mL, ~2.7 µM) and neutralizes the initially formed factor Xa and thrombin.1,3 Overall factor VIIa patrols the circulation in search of sites of vascular injury where TF is exposed, and trace quantities of factor Xa and thrombin initiate a procoagulant response. Plasma levels of the different coagulation proteins are listed in Table 27-1.

Propagation of Coagulation
Platelets further amplify or potentially initiate clot formation at the site of vascular injury. Inflammatory cells all contain adhesion molecules to facilitate binding in the rapid flow of blood vessels as shown in Figure 27-1. Following vascular injury and exposure of the subendothelial vascular basement membrane, von Willebrand factor (vWF) that circulates in a multimeric form binds to the damaged blood vessel. Platelets then adhere to subendothelial collagen-vWF via their glycoprotein (GP) Ib receptors and are activated. Thrombin generation that also occurs locally is a potent activator/agonist for platelets by stimulating protease activated receptor (PAR)-1 and PAR-4.1,3 Thrombin activation of platelets further amplifies clot formation by multiple mechanisms. Platelet glycoprotein Ib receptors bind to factor XI, and they also localize factor VIII to the site of endothelial disruption via its carrier protein vWF.5 Also, factor V is released from platelet α-granules upon platelet activation, and factors XI, VIII, and V further amplify and sustain procoagulant responses (the “intrinsic pathway”) after thrombin-mediated activation. The serine protease factor XIa mediates the activation of factor IX to factor Xa, and factor VIIIa serves as a cofactor to factor IXa. Factor IXa, a serine protease, activates factor X to factor Xa, and factor Va serves as a cofactor to factor Xa.1,3 In the absence of factor VIIIa or factor IXa, as is clinically observed in hemophilia A or B, the initiation of coagulation is normal, but amplification/propagation is altered. Patients with hemophilia clot, but they develop bleeding in muscle and joints due to low TF expression.
Tissue Factor, Thrombin, and Fibrin(ogen) in Clot Formation and Stability
When generated, thrombin facilitates the proteolytic conversion of circulating, soluble fibrinogen to an insoluble fibrin meshwork. This complex mechanism involves the cleavage of N-terminal peptides from fibrinogen, end-to-end polymerization of fibrin monomers to protofibrils, and lateral aggregation of protofibrils to fibers.1,3 Fibrin’s biophysical characteristics provide extensive structural support to the clot; individual fibers can be strained >330% without rupturing. The fibrin network that forms can be influenced by many different factors including fibrin(ogen)-binding proteins (e.g., factor XIII), thrombin, and fibrinogen present during fibrin formation.
Role of Fibrinogen
Fibrinogen is a critical protein for clot formation, and has a critical role in hemostasis.6 Fibrinogen is critical for clot formation and creating the dense lattice structure as shown in Figure 27-3. Fibrinogen also binds to platelet glycoprotein IIb/IIIa receptors to facilitate clot formation and is affected by many antiplatelet agents. In addition, fibrinogen facilitates the cross-linking and network formation for clot and the subsequent fibrin polymerization that is catalyzed by thrombin and thrombin-activated factor XIII that locally at the site of activation. Of all the coagulation factors, fibrinogen circulates at the highest concentration (7.6 µM, ~200 to 400 mg/dL). In pregnancy and during acute inflammatory responses that often occur postoperatively, fibrinogen is an acute-phase reactant.6 Platelets that are activated by multiple agonists express glycoprotein IIb/IIIa receptors. Thrombin catalyzes the conversion of fibrinogen to fibrin monomers after thrombin cleaves the fibrinopeptides from the fibrinogen Aa and B3 chains. Platelets that are activated release factor XIII A subunits that further polymerize fibrin monomers into fibrin. Activated factor XIII also cross-links a2-antiplasmin to fibrin, making fibrin more resistant to degradation. Thrombin released locally modulates the thickness and the fibrinolytic resistance of fibrin fibers.

Thrombin generation is critical to clot formation, platelet activation, and fibrinogen cross linking.1,3 With normal hemostatic function, the peak thrombin level reaches 200 to 500 nM, facilitating the formation of a dense fibrin network for normal clot function and hemostasis. However, in patients with hemophilia and other bleeding abnormalities, lower levels of thrombin generation occur and as a result clot formation is altered, thus hemophiliacs commonly bleed into joints. Thrombin generation at the junction of the injured vasculature and subendothelial basement membrane is amplified by platelet activation and release of procoagulant microparticles.1,3 Thrombin has a complex effect of releasing tissue plasminogen activator (t-PA) to simultaneously initiate fibrinolysis. This occurs by binding to thrombomodulin but also by activated factor XIII and activation of thrombin-activatable fibrinolysis inhibitor (also called TAFI). Fibrin polymerization, thrombin, and activated factor X generated at the site of vascular injury site are released systemically.
Critical Factor Levels for Hemostasis
A critical question is what levels of fibrinogen, platelets, and other coagulation proteins are necessary to optimize hemostasis in the surgical patients. Many blood bank experts as well as older guidelines only recommended treating fibrinogen levels if they have decreased below ~1.0 g/L (100 mg/dL), a level similar to the management of congenital afibrinogenemia.6 There is increasing data about the critical role of fibrinogen and current European guidelines recommend higher fibrinogen levels of 1.5 to 2.0 g/L for treating coagulopathy. Recent studies have demonstrated the critical role of fibrinogen for aortic surgery, postpartum hemorrhage, cardiac surgery, and cystectomy. Of note is that studies suggest that further normalization of fibrinogen to levels more consistent with normal circulating concentrations of 2 to 3 g/L may be important for adequate hemostasis.
Role of Factor XIII
Factor XIII plays a major role in the terminal phase of the clotting cascade that promotes formation of cross-linked fibrin polymers and generation of a stable hemostatic plug.7 Factor XIII exists as a tetrameric precursor (zymogen) of 2 A and 2 B subunits and is converted into an active transglutaminase (factor XIIIa) by thrombin and calcium.7 In this activated form, factor XIIIa mechanically stabilizes fibrin and protects it from fibrinolysis. As a result, patients with a deficiency in factor XIII develop a rare but severe bleeding disorder.8,9 A congenital deficiency in factor XIII is clinically defined as a plasma level of less than 5% of the protein.7 Most cases of factor XIII deficiency are due to lack of the A subunit with less than 1% factor XIII activity. Congenital factor XIII deficiency is inherited as an autosomal recessive disease and was first reported in Switzerland in 1960.10 The incidence of factor XIII deficiency is currently estimated as one in 3 to 5 million births in the United States.11 An acquired deficiency in factor XIII can arise from the development of antibodies against factor XIII. We have also studied factor XIII repletion, and although it did not decrease bleeding or transfusion requirement in cardiac surgery, levels of >50% to 60% may to reduce bleeding in surgical patients with low fibrinogen levels (<1.5 g/L).7
Role of Platelets and von Willebrand Factor
Platelets adhere to sites of vascular injury and to each other by direct and indirect effects that are part of a complex cellular mechanism required for hemostasis. Although platelet aggregation is mediated in part by bridging/binding of the integrin glycoprotein IIb/IIIa on platelet surfaces by the adhesive protein fibrinogen, this process is far more complex than simple interactions and the lattice formation of the two elements.
There are multistep adhesion processes involving distinct receptors and adhesive ligands that are also dependent on flow conditions, especially with the critical role of platelet function in arterial hemostatic mechanisms. Thus, following vascular injury, the subendothelial surface is exposed which then binds to vWF that is synthesized in the endothelium and critical for platelet adhesion in arteries and arterioles that have high shear rates.
vWF is critical to facilitate platelet adhesion in rapid blood flow environments. vWF binds to an adhesion ligand, its platelet membrane receptor, GPIb-IX-V. Once platelets adhere, they are activated by a complex series of steps including release of adenosine diphosphate (ADP) and thromboxane A2, agonists that activate additional platelets and bind P2Y12 receptors and express IIb/IIIa receptors. These important receptors are the target of common pharmacologic agents including clopidogrel, prasugrel, and ticagrelor. Platelets provide a catalytic membrane surface for further thrombin generation and clot formation and mediate additional platelet and leukocyte recruitment by mechanisms that include release of microparticles that mediate leukocyte-leukocyte and leukocyte–endothelial cell interactions. When activated, platelets may also form occlusive thrombi in cardiovascular diseases that result in myocardial infarction, stroke, or other acute ischemic syndromes of other organs.
Endothelial Regulation of Coagulation
The vascular endothelium provides an extensive interface that is critical for both anticoagulant and procoagulants functions as shown in Figure 27-2.12–15 Increased shear forces and flow across the endothelium release important anticoagulation agents (Table 27-2) that include a diverse series of molecules, including nitric oxide, prostacyclin, and ecto-ADPase that degrade the platelet agonist ADP. Additionally, endothelium-derived TFPI is localized on the surface of vascular endothelium, and reduces the procoagulant activities of the TF–factor VIIa initiation step.1,3 Heparin sulfate is located on endothelial surfaces and binds antithrombin in the circulation to further provide anticoagulant activity on the vascular surface. Thrombin is scavenged to keep thrombin activity local at the site of vascular injury by another endothelium-bound protein called thrombomodulin.1,3 Thrombomodulin is used clinically in Japan for DIC and is currently being studied for treatment of sepsis in the United States. Endothelial activation also provides anticoagulation by releasing t-PA from the endothelial stores of the Weibel-Palade bodies. t-PA activates plasmin from plasminogen which, in turn, promotes fibrinolysis, a critical component of vascular patency.1,3


Full access? Get Clinical Tree
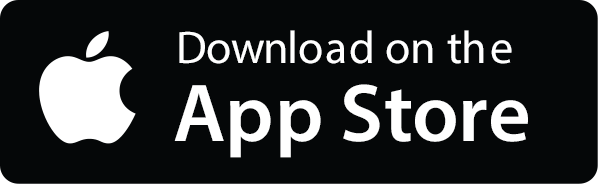
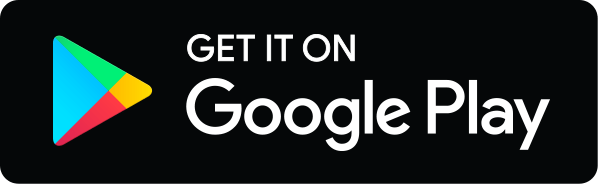