Abstract
Anesthesiologists are frequently asked to care for patients at the extremes of age and size. These patient groups have unique features that require special consideration when formulating an anesthetic plan. The purpose of this chapter is to highlight alterations in physiology associated with these special populations, focusing in particular on how the altered physiology influences the behavior of anesthetic drugs. To translate this information into the clinical domain, the chapter aims to summarize dosing adjustments that account for body habitus (obese and morbidly obese) and age at both extremes (neonates and elderly).
Keywords
obesity, pharmacokinetics, elderly, neonate, age, size
Chapter Outline
Anesthesiologists frequently care for patients at the extremes of age and size. These patient groups have unique features that require special consideration when formulating an anesthetic plan. The purpose of this chapter is to highlight alterations in physiology associated with these special populations, focusing in particular on how the altered physiology influences the behavior of anesthetic drugs as covered in other chapters. To translate this information into the clinical domain, the chapter aims to summarize dosing adjustments that account for body habitus (obese and morbidly obese) and age at both extremes (neonates and elderly).
Obese Patients
Physiologic Changes in Obese Patients
Obesity presents anesthesiologists with numerous challenges that affect their care throughout the perioperative period. Excess body weight affects virtually every system of the body, including the cardiovascular, respiratory, endocrine, digestive, and hematologic systems, among others. In particular, increased chest wall mass, redundant airway tissue, and increased incidence of obstructive sleep apnea make this patient group at increased risk for adverse airway and respiratory events among many other weight-related issues ( Table 5.1 , Fig. 5.1 ).
Weight-Related Condition | Challenge | Management Considerations |
---|---|---|
Redundant upper airway tissue | Obstructive sleep apnea | Continuous positive airway pressure to stent open the airway when appropriate (procedures requiring sedation, recovery from general anesthesia) |
Potential difficulty with airway management (more likely in males ) | Immediate availability of airway adjuncts (e.g., video laryngoscope, supraglottic airways, elastomeric bougie, fiberoptic bronchoscope) and patient positioning | |
Redundant tissue over chest and abdomen | Reduced functional residual capacity | Induction of anesthesia: Anticipate rapid drop in oxygen hemoglobin saturation once rendered apneic despite effective preoxygenation Emergence from anesthesia: Extubate to continuous positive airway pressure. Place head of bed to 45° to optimize pulmonary mechanics. |
With mechanical ventilation, may require high airway pressures to achieve adequate ventilation, leading to an increased risk of barotrauma | Maintain plateau airway pressures <30 cmH 2 0, tidal volumes between 6-8 mL/kg, and judicious titration of positive end-expiratory pressure | |
Gastroesophageal reflux disease | Increased risk of aspiration | Avoid use of supraglottic airway devices. Consider preoperative administration of a H 2 -histamine antagonist |
Cardiovascular disease (hypertension, coronary artery disease ) | Increased likelihood of intraoperative myocardial ischemia and arrhythmias |
|
Venous thromboembolism | Deep vein thrombosis or pulmonary embolism | Prophylactic anticoagulation and intermittent pneumatic leg compression |
Hyperglycemia/insulin resistance | Perioperative hyperglycemia | Consider insulin administration to maintain blood sugar concentrations in the 140-180 mg/dL range |

In response to the increasing frequency of obese patients presenting for surgery, the American Society of Anesthesiologists (ASA) updated the ASA’s Physical Status Classification System to include obesity as a metric to consider when determining a patient’s physical status ( Table 5.2 ). This modification encourages clinicians to acknowledge how obesity-related conditions can lead to adverse events in the perioperative period. Of particular relevance to anesthesiology, obesity can alter the pharmacokinetics and effects of intravenous agents (opioids, sedatives, neuromuscular blockers) and inhalation agents. Increased fat mass, increased blood volume and cardiac output, and reduced tissue perfusion change drug disposition that can lead to changes in the magnitude and time course of drug effect ( Table 5.3 , see Fig. 5.1 ).
ASA Physical Status | BMI Range (kg/m 2 ) |
---|---|
1 | ≤30 |
2 | 31-39 |
3 | ≥40 |
Physiologic Change |
Increased cardiac output can alter early drug distribution (e.g., front-end kinetics) |
Reduced tissue perfusion slowing uptake of subcutaneous injections |
Increased or decreased hepatic cytochrome P450 microsomal enzyme function |
Alterations in hepatic drug biotransformation via uridine diphosphate glucuronosyltransferase |
Alteration in the glomerular filtration and/or renal tubular-mediated drug excretion |
The fact that most anesthetics are highly lipophilic (i.e., as measured by their octanol-water coefficient) raises concerns about accumulation of anesthetics into fatty tissue over the course of an anesthetic. When exploring differences between drugs, however, lipophilicity alone does not reliably predict volume of distribution. Lipophilic drugs can have increased, unchanged, or reduced distribution volumes because drug distribution also depends on other properties, such as protein binding, molecular size, ionization at physiologic pH (pKa), among other factors.
Understanding the relationship between total body weight, lean body weight, and fat weight is also important. As total body weight increases beyond ideal body weight (i.e., as body mass index [BMI] increases), the proportion of lean and fatty tissue begins to diverge ( Fig. 5.2 ). Above a BMI of 30 kg/m 2 , lean body weight begins to plateau, and the curve flattens even further as BMI exceeds 40 kg/m 2 as the body has proportionally less lean tissue. Obesity influences the physiology of most organ systems that can alter the pharmacologic behavior of anesthetic drugs. Although drugs from the same class (e.g., opioids) are likely to be influenced by obesity in similar ways, it is difficult to generalize obesity-related changes across all classes of anesthetics.

Anesthetic Pharmacology in Obesity
Pharmacology is the study of drug dosing (see Chapters 2 and 3 ). Anesthetic pharmacology in the morbidly obese is challenging. Although many anesthetic drugs are traditionally dosed according to total body weight (e.g., in milligrams per kilogram or micrograms per kilogram per minute), most weight-based dosing guidelines (i.e., drug labeling in the official “package insert”) are founded on data collected in subjects with normal body habitus. For most anesthetic drugs, the guidance that the dose should be altered as body weight increases might never have been tested in a rigorous way. In fact, when formulating an anesthetic regimen for a morbidly obese patient, using total body weight to calculate an appropriate dose is likely to lead to an overdose. This observation is a practical reality that anesthesiologists intuitively understand based on clinical experience. For example, a 120-kg patient does not require twice as much anesthetic as a patient of the same height who weighs 60 kg.
To address this, researchers have explored numerous weight scalars in an effort to minimize the risk of inadequate or excessive dosing in obese patients. The goal is to identify a dose for an obese patient that would behave similar to a conventional dose for a normal-sized patient. Some examples include ideal body weight (IBW), lean body mass (LBW), fat-free mass (FFM), adjusted body weight (ABW), and pharmacokinetic mass (PK mass). Table 5.4 presents the formulas used to calculate selected weight scalars. To provide a clinical context for these weight scalars, Table 5.5 presents examples of scaled weights for an obese and lean individual of the same height and the respective induction doses of commonly used intravenous anesthetics.
Propofol (2 mg/kg) | Fentanyl (2 mg/kg) | Rocuronium (0.6 mg/kg) | ||||||
---|---|---|---|---|---|---|---|---|
Scaled Weight (kg) | Total mL of Propofol at 10 mg/mL b | Total mL of Fentanyl at 50 µg/mL b | Total mL of Rocuronium at 10 mg/mL b | |||||
Dosing Scalar | Lean | Obese | Lean | Obese | Lean | Obese | Lean | Obese |
Total body weight | 60 | 120 | 12 | 24 | 2 | 5 | 4 | 7 |
Ideal body weight | 66 | 66 | 13 | 13 | 3 | 3 | 4 | 4 |
Lean body weight | 51 | 70 | 10 | 14 | 2 | 3 | 3 | 4 |
Fat-free mass | 51 | 73 | 10 | 15 | 2 | 3 | 3 | 4 |
Adjusted body weight | 64 | 88 | 13 | 18 | 3 | 4 | 4 | 5 |
Pharmacokinetic mass | 58 | 93 | 12 | 18 | 2 | 4 | 4 | 6 |
a Induction doses are shown in milliliters. The lean individual weight 60 kg with body mass index of 20 kg/m 2 . The obese individual body mass index is 40 kg/m. The lean and obese individuals are of the same height (173 cm)
Of note from Table 5.5 , using the IBW scalar yields doses that are equivalent, regardless of body habitus, the doses would likely be adequate for the lean but insufficient for the obese individual. Using LBW or FFM scalars yields doses that are lower for the lean individual but higher for the obese individual compared with IBW, but both may be inadequate, because LBW or FFM is what remains after fat mass is subtracted from TBW. Using LBW or FFM consistently results in underdosing for both lean and obese patients. Using ABW or PK mass scalars by contrast recommends doses that may be sufficient for induction for both the lean and obese individuals. Lean-scaled weight (LSW) is another weight scalar proposed for use in the operating room for drugs that scale consistent with LBW. It is based on LBW, TBW, and BMI and allows estimation of doses based on a percentage of TBW. LBW has not been validated in vivo.
Identifying the optimal weight scalar has proven to be complex. To add to the complexity, the appropriate weight scalar for a bolus dose can differ from the scalar best suited for continuous infusion. Furthermore, weight scalar choice can vary by drug or drug class. Table 5.6 presents suggested weight scalars for selected anesthetics. Some have been identified through direct experimentation in obese patient populations, whereas others are assumed based on the premise that drugs of a similar class are likely to behave similarly because they often share similar physicochemical characteristics.
Drug | Bolus | Infusion |
---|---|---|
Sedatives | ||
Propofol | Loading dose titrated to target processed EEG level a | ABW |
Midazolam | TBW b | IBW b |
Etomidate | IBW | |
Opioids | ||
Fentanyl | PK mass | PK mass |
Remifentanil | FFM | FFM |
Sufentanil | TBW | |
Hydromorphone | LBW | |
Morphine | LBW | |
Neuromuscular Blocking Agents and Reversals | ||
Succinylcholine | TBW | |
Rocuronium | IBW | |
Vecuronium | IBW | |
Cisatracurium | IBW | |
Sugammadex | IBW |
a Prior work has explored the use of several weight scalars for propofol. See text for details.
For anesthesia clinicians who use target-controlled infusions (TCI), obesity introduces the additional issue of selecting the appropriate pharmacokinetic model to drive the infusion. Although suitable pharmacokinetic models exist for many commonly used sedative-hypnotics, opioids, and neuromuscular blockers, some models are suboptimal when applied to special populations (e.g., extremes of weight or age). Furthermore, recent studies aimed at building new models for these special populations have not yet been incorporated into the software of TCI pumps. This problem has forced the clinician to develop creative work-arounds to address the shortcomings of TCI models.
The remainder of this section briefly discusses pharmacokinetic changes associated with obesity for selected sedative-hypnotics, opioids, and potent inhaled agents, reviewing examples of weight scalars and their limitations when used to formulate a rational dosing scheme. A brief review of available pharmacokinetic models used to drive TCI pumps is also provided.
Sedative-Hypnotics
Propofol is the most comprehensively studied sedative-hypnotic in the obese population. TBW has been recommended for calculation of maintenance continuous infusion rates, although recent work suggests using ABW to account for the nonlinear relationship between propofol clearance and TBW. There are also many facets to consider when choosing a scalar for bolus dosing for induction of anesthesia. To avoid medication overdose, LBW has been proposed. As illustrated in Table 5.5 , however, use of this scalar might result in underdosing and ABW might be more appropriate. With no established optimal weight scalar, recent work has explored other methods to determine the optimal bolus dose of propofol in obese patients.
One study compared induction of anesthesia with propofol based on LBW versus a loading dose (rapid propofol infusion) titrated to a target processed electroencephalographic (EEG) signal (bispectral index scale [BIS]). Patients induced using the LBW scalar required additional propofol doses to reach the same level of sedation compared with patients titrated to a BIS value of 50. The bolus technique required substantially less time to administer but was not as effective. The loading dose approach led to a more consistent induction of anesthesia and no need for supplemental doses. Thus titrating to a target BIS might be a useful approach in this patient group because of the lack of clear dosing guidelines on a per kilogram basis.
It is clear that significantly obese patients require a moderately higher dose of propofol to achieve the same propofol concentration compared with lean patients. As illustrated in the pharmacokinetic simulation shown in Fig. 5.3 , when an exceptionally obese patient receives the same absolute dose of propofol as a lean patient of the same height (i.e., a typical induction and infusion dose for a lean patient), lower propofol blood concentrations are produced. This conclusion is generally consistent with emerging knowledge regarding the impact of extreme obesity on the disposition of most intravenous anesthetic drugs.

With regard to TCI, there are several propofol pharmacokinetic models available to drive TCI pumps. Data used to build these models included a variety of patient groups (i.e., lean and obese patients, pediatric and elderly patients). When constructing these models, several patient variables (i.e., age, TBW, LBW, and sex) were introduced into selected models to assess whether they improve model performance as determined by how well the model predicted measured plasma propofol concentrations.
When using a TCI pump in an obese patient, two important questions emerge: which pharmacokinetic model is optimal in the obese patient and what weight should be used (i.e., IBW, LBM, TBW)? Cortinez and colleagues studied how well selected propofol pharmacokinetic models predicted plasma propofol concentrations in a morbidly obese patient group in an effort to identify the best model and weight scalar. They found that two models, the Schnider model and the Marsh model, performed best when using ABW. For propofol, using a modified weight scalar with the Schnider or Marsh model may be best suited for TCI in obese and morbidly obese patients. Both of these models are available in many commercial TCI pumps.
Perhaps the most versitile pharmacokinetic model for propofol was recently developed by Eleveld and colleagues. They combined data from 21 propofol pharmacokinetics datasets that included elderly adults, obese adults, children, and normal-sized adult patients and volunteers. From these data they built a propofol pharmacokinetic model incorporating age, sex, and weight as covariates that performs well compared to other propofol models designed for specific-patient populations. This generalizable propofol pharmacokientic model has the abilty to cover a wide range of patient groups. An important caveat to consider when selecting a pharmacokinetic model is that differences in model predictions are likely overshadowed by significant interindividual variability. Many of the available propofol pharmacokinetic models will likely yield similar clinical results if titrated to effect in conjunction with processed EEG monitoring.
Unlike propofol, the influence of obesity of the pharmacokinetic behavior of other sedatives is not as well studied. Weight scalars for thiopental and midazolam, but not ketamine and etomidate, have been reported. Work with midazolam in obese patients found that as patient size increases, so does volume of distribution. However, the time to peak plasma concentrations and metabolism were the same regardless of body size. Elimination half-life for midazolam more than doubled compared with normal-sized counterparts, probably because of the large volume of distribution in morbidly obese patients. The clinical implications of this long elimination half-life were not evaluated, but the authors recommended that bolus doses be scaled to TBW and continuous infusion be dosed to IBW.
Opioids
Similar to propofol, remifentanil is perhaps the most well-studied opioid in terms of its pharmacologic behavior in obese patients. Overall, the disposition of remifentanil in obese and lean patients is similar, but obese patients do indeed require a higher dosage. Researchers have identified that FFM provides a reasonable weight scalar for continuous infusions. With regard to TCI, several pharmacokinetic models have been devleoped from data collected in a wide range of patient sizes optimizing model predictions using weight, age, and sex as covariates. Many of the model parameters were scaled to LBM (Minto model), modifications of LBM (La Colla model) or an allometric scaling of TBW among other covariates (Kim model). Although the Minto model is the most widely used model for TCI administration of remifentanil, it is known to have serious problems when applied to very obese patients, in part because not many obese patients were included in the dataset from which the model was built (and because of faulty equations for the estimation of LBM). Because of these shortcomings in the Minto model, various work-arounds have been proposed that include entering “fictitious” body weights during initiation of TCI therapy; this approach can be useful but is obviously suboptimal. The Kim model addresses the problems associated with the Minto model by including numerous obese subjects into the model dataset and by using updated equations for estimation of body mass indices such as LBM. As the Eleveld model for propofol, the Kim model for remifentanil may prove advantageous in obese patients but has not been clinically validated.
Recent work has combined previously published datasets to refine pharmacokinetic models (see the “Emerging Developments” section ). Consider the series of simulations based on the model of Kim and colleagues to visualize the influence of weight on the pharmacologic behavior of remifentanil (see Figs. 5.4 through 5.6 ). In the first simulation, predicted remifentanil plasma concentrations are plotted over time for an equivalent bolus dose followed by a 60-minute infusion administered to a lean and obese individual of the same age and height ( Fig. 5.4 ). Predicted plasma concentrations were approximately 78% lower in the obese individual. In the second simulation ( Fig. 5.5 ), the authors explore what infusion rate (in micrograms per minute) would be necessary to maintain a target plasma concentration of 5 ng/mL for 30 minutes, a concentration known to provide analgesia across weights of 50 to 200 kg. Infusion rates were nearly doubled to achieve the target concentration over this weight range. Because of remifentanil’s rapid clearance, the required infusion rates stabilize after approximately 25 minutes. In a third simulation, the authors predicted the cumulative remifentanil dose for three individuals (lean young individual, lean elderly individual, and obese young individual) to maintain a target plasma concentration of 5 ng/mL for a 5-hour infusion ( Fig. 5.6 ). The cumulative dose for the young obese individual was approximately 125% of the young lean individual. This set of simulations provides a visual illustration of the increased dosing requirements for heavier patients.



Unlike propofol and remifentanil, relatively little work has explored how obesity affects fentanyl pharmacokinetics. Shibutani and colleagues characterized a nonlinear relationship between fentanyl clearance and TBW and recommended use of a modified weight, called the pharmacokinetic mass, which resulted in effect site concentrations in obese patients that were similar to effect site concentrations in lean patients.
For other opioids, even less information is available. Some work has explored sufentanil pharmacokinetics in obese patients, and similar to midazolam, a linear increase was reported in the volume of distribution with an increase in TBW but similar clearance between lean and obese individuals. The authors suggest that for bolus dosing TBW be used and continuous infusions be judiciously reduced. Use of a sufentanil pharmacokinetic model may be suitable for sufentanil TCIs in morbidly obese patients.
Inhaled Anesthetics
A common perception of potent inhaled agents is that they accumulate in obese more than lean patients and that this prolongs emergence. Research, however, has not confirmed this perception. Two pharmacokinetic factors contribute to this observation. First, the time required for adipose tissue to equilibrate with the inspired volatile anesthetic concentration is long; the times required to achieve 63% of the final equilibrium in adipose tissue for desflurane and isoflurane exceed 22 and 35 hours, respectively. Second, blood flow to adipose tissue decreases with increasing obesity, reducing the overall delivery of drug to this tissue bed.
Based on their pharmacokinetic profiles, there could be some advantage to choosing one potent inhaled agent over others. Desflurane has a faster washout and improved recovery compared with sevoflurane in obese individuals when no premedication is used. With a quicker recovery, desflurane might be well suited for use in this patient group to minimize adverse effects related to slow emergence in patients with limited oxygen reserves as a result of a reduced functional residual capacity. Given the pronounced interaction between volatile anesthetics and opioids or sedatives, addition of midazolam or fentanyl will likely reduce desflurane’s advantages over sevoflurane.
Neuromuscular Blocking Drugs
Formulating a rational dosage scheme for neuromuscular blockade in obese patients can be challenging given the increased likelihood of difficult intubation, the need for excellent intubating conditions on the first attempt, and the need to provide mask ventilation should intubation be unsuccessful. Prior work suggests that TBW is appropriate for succinylcholine and IBW is appropriate for nondepolarizing agents (see Table 5.6 ). As a rule of thumb, clinicians sacrifice onset time with a lower total dose but gain a faster recovery (see Chapter 22 ).
Succinylcholine was studied in 45 morbidly obese patients (BMI > 40 kg/m 2 ) comparing a dose of 1 mg/kg using three different weight scalars: IBW, LBW, and TBW. Across groups, there was no difference in onset time. Longer recovery time correlated with the larger overall dose (e.g., 1 mg/kg using TBW being the largest), although recovery times were relatively short compared with other longer-acting neuromuscular blocking drugs. Intubating conditions were adequate in all groups, but laryngoscopic view was better in the group dosed to TBW. The authors recommended dosing succinylcholine based on TBW, prioritizing the laryngoscopic view over duration of blockade.
Studies comparing TBW and IBW as the basis for dosage calculation for rocuronium in morbidly obese patients found that at a dose of 0.6 mg/kg using TBW led to a prolonged duration of action but did not improve intubating conditions compared to IBW. Similar results have been observed with vecuronium. Thus the consensus is to dose rocuronium and vecuronium to IBW to achieve a shorter duration of blockade without compromising intubating conditions.
For neuromuscular blockade reversal with sugammadex, weight scalar recommendations vary. Some authors recommend using IBW and others suggest using ABW, whereas a pooled analysis of 1418 adult obese patients led to a recommendation that sugammadex be dosed according to TBW. Given the favorable sugammadex safety profile, dosing to TBW is a reasonable approach. Reversal doses are a function of blockade depth. Use of a peripheral nerve stimulator capable of quantitative train-of-four monitoring allows accurate determination of blockade depth and the appropriate sugammadex dose. In brief, profound (no train-of-four, no posttetanic twitch), deep (no train-of-four, one or more posttetanic twitches), moderate (one to three train-of-four count), shallow (train-of-four ratio 0.1-0.4), and minimal (train-of-four ratio > 0.4) blockade are reversed with 16, 4 to 8, 2, 2, and 2 mg/kg of sugammadex, respectively.
An interesting feature of sugammadex reversal is that it can be used to provide rapid reversal after administration of high-dose rocuronium (e.g., 1.2 mg/kg) following a rapid sequence induction. In an emergency setting of “can’t intubate and can’t oxygenate,” deep blockade by rocuronium can be completely reversed faster than spontaneous recovery from an intubating dose of succinylcholine (1 mg/kg). Although sugammadex reversal is fast regardless of blockade depth, a simulation study concluded that residual effects from induction doses of sedatives and opioids may persist after blockade reversal. Thus, although they are no longer paralyzed, patients can remain unresponsive or have significant respiratory depression. If both unresponsiveness and respiratory depression exist, patients might not be able to self-rescue from a “can’t intubate–can’t oxygenate” scenario. Given that obese patients have reduced functional residual capacity and a shortened time to oxygen-hemoglobin desaturation when rendered apneic despite adequate preoxygenation, they are at increased risk for hypoxia. Another important consideration when administering a large sugammadex dose (e.g., 16 mg/kg) is that the dose might not be immediately available at the point of care without proper preoperative planning and preparation.
Local Anesthetics
The safe dose of local anesthetics varies with the route of administration, the properties of the local anesthetic, and the addition of epinephrine. Plasma concentrations are the highest after intercostal nerve block and decrease as follows: intercostal > caudal > epidural > brachial plexus > sciatic/femoral. Plasma concentrations of local anesthetics do not correlate well with body weight or BMI in adults but correlate better with weight in children. Local anesthetic toxic doses have not been well studied in obese patients. The widely recommended axiom of the smallest effective dose is appropriate in this patient population.
Pediatric Patients
When formulating an anesthetic dosing regimen for a pediatric patient, an important consideration is that children are not miniature adults. Anatomic and physiologic changes that occur between the newborn period and adult life have important pharmacologic implications. In part because the early drug development process is focused on adult volunteers and patients, these developmental changes are often not well accounted for in available dosing guidelines. Ideally, dosing recommendations should be based on research in children along all phases of development and maturation to characterize drug pharmacokinetics and pharmacodynamics properly. Unfortunately, detailed information along these lines is not available for most anesthetics.
Given the cost of drug development, pharmaceutical companies often seek approval from regulatory agencies for adults, but not children. As such, many anesthetics are administered “off-label” using adult doses scaled to pediatric patients. In response, the United States National Institute of Childhood Health and Human Development formed the Pediatric Pharmacology Research Unit Network in 1994, and in 1997, the U.S. Food and Drug Administration (FDA) developed the Modernization Act to incentivize drug research in pediatric patients by extending the duration of market exclusivity for approved drugs.
One challenge is to account for changes in body composition, metabolic capacity, and body surface area that occur with age. Several approaches have been explored (e.g., dosing based on body surface area or allometric scaling), but the most common approach is to use weight-normalized dosing according to age (e.g., where available milligrams per kilograms for neonates up to 44 weeks’ postconception in age), infants (1-12 months of age), and children (up to 12 years of age).
Physiologic Changes in Pediatric Patients
Neonates, infants, and children present anesthesiologists with challenges throughout the perioperative period. Selected physiologic changes such as differences in airway anatomy, chest wall and respiratory system function, and cardiovascular function place this patient group at increased risk for adverse airway and respiratory events as well as other pediatric issues ( Table 5.7 , Fig. 5.7 ). For many of these physiologic changes, inherent variability is associated with maturation and interpatient and intrapatient differences that influence anesthetic drug behavior and alter dosing considerations.
Neonate-/Infant-Related Condition | Challenge | Management Considerations |
---|---|---|
| Airway obstruction |
|
Difficult laryngoscopy |
| |
|
|
|
Airway closure at expiration and rapid respiratory muscle fatigue | Consider using positive end-expiration pressure, continuous positive airway pressure, or intermittent positive-pressure ventilation to stent open airway and reduce work of breathing | |
Apneas longer than 15 seconds or associated with hypoxia and bradycardia |
| |
| Prone to bradycardia and low cardiac output | Treat neonates with heart rate <100 beats/min with restoration of oxygenation and epinephrine 10 µg/kg |
| Dehydration | Maintain urine output 1-2 mL/kg |
| Decreased opioid metabolism | Prolonged opioid effect |
| Hypoglycemia | Glucose infusion in neonates at risk |
Hypothermia | Consider techniques to increase core body temperature (ambient temperatures of 32°C-34°C for neonates and premature infants) | |
| Prolonged and variable duration of action | Consider judicious dosing of opioids |

For example, neonates and infants have greater total body water than their adult counterparts, resulting in a larger volume of distribution with water-soluble drugs such as succinylcholine and many antibiotics. With a larger volume of distribution, a higher initial loading dose is required to achieve therapeutic plasma concentrations. Neonates also have lower serum albumin and total protein concentrations, resulting in higher free plasma levels of highly protein-bound drugs, such as bupivacaine, opioids, sedatives, and antibiotics. The kidneys and liver of neonates are immature compared with those of adults. The developmental physiology of the liver is complex, but in general hepatic enzymatic drug metabolism is decreased in neonates, and the neonatal liver receives disproportionately less cardiac output than an older child or adult. The neonatal kidney is less efficient, prolonging the half-life of drugs primarily excreted through the kidneys. Neonates also have a decreased proportion of body mass as fat and muscle, which can prolong drug effects depending on redistribution into those tissues.
Anesthetic Pharmacology in Pediatric Patients
In general, anesthesiologists can expect significant variability in anesthetic dosing of pediatric patients. Thus only general dosing guidelines are possible for most anesthetic drug classes. A brief discussion of dosing recommendations for selected anesthetics is provided in the following text and summarized in Table 5.8 .
Drug | Route of Administration | Dose |
---|---|---|
Sedatives | ||
Propofol | IV: Induction (age >3 yr) | 2.5-3.5 mg/kg |
IV: Sedation (infusion) | 125-150 µg/kg/min | |
Midazolam | PO: Premedication | 0.25-0.5 mg/kg (maximum 20 mg) |
Intranasal: Premedication | 0.2-0.3 mg/kg | |
IV: Premedication | 0.05 mg/kg | |
Intranasal: Sedation | 0.2-0.3 mg/kg (maximum 10 mg per dose) | |
IM: Sedation | 0.1-0.15 mg/kg | |
IV: Sedation (age 6 mo to 5 yr) | 0.05-0.1 mg/kg (maximum 6 mg) | |
PR: Sedation | 1 mg/kg | |
SL: Sedation | 0.5-0.75 mg/kg | |
IV infusion: Sedation (neonates <32 wk) | 0.03 mg/kg per hour | |
IV infusion: Sedation (neonates >32 wk) | 0.06-0.12 mg/kg per hour; titrate to effect | |
Etomidate | IV: Induction | 0.3 mg/kg (0.2-0.6 mg/kg) |
IV: Sedation | 0.1-0.3 mg/kg; repeat as needed | |
Ketamine | PO: Premedication | 6-10 mg/kg |
IM: Premedication | 3-7 mg/kg | |
IV: Sedation | 0.5-2 mg/kg per dose | |
IV: Induction | 1-2 mg/kg | |
IV: Analgesic | 0.1 mg/kg | |
IV: Analgesic (infusion) | 0.1-0.3 mg/kg per hour | |
Opioids | ||
Fentanyl | IV bolus: Analgesic | 1-2 µg/kg |
IV bolus: Loading dose | 5-10 µg/kg | |
IV: Infusion | 1-3 µg/kg per hour | |
Intranasal | 1-2 µg/kg | |
Oral transmucosal fentanyl | 5-15 µg/kg | |
Remifentanil | IV: Bolus | 1 µg/kg |
IV: Infusion | 0.25-3.0 µg/kg per minute | |
Sufentanil | IV bolus: Loading dose | 1-2 µg/kg |
IV: Infusion | 0.1-0.2 µg/kg per hour | |
Intranasal | 2 µg/kg | |
Hydromorphone | PO (children) | 0.05 mg/kg q4-6h |
PO: (adolescents) | 1-4 mg-dose q4-6h | |
IV: Bolus | 5-10 µg/kg q4-6h | |
IV: Continuous | 3-5 µg/kg per hour | |
Caudal | 10 µg/kg | |
Morphine | PO: Immediate release | 0.3 mg/kg per dose q4-6h |
PO: Continuous release | 0.3-0.6 mg/kg per dose q12h | |
IV: Infusion (neonates) | 0.01-0.02 mg/kg per hour | |
IV: Infusion (children) | 0.01-0.04 mg/kg per hour | |
Caudal | 30-50 µg/kg | |
Intrathecal | 5-7.5 µg/kg | |
Neuromuscular Blocking Agents and Reversal | ||
Succinylcholine | IV: Fast onset | 1.5-2 mg/kg |
IM | 4 mg/kg | |
Rocuronium | IV: Intubation | 0.3-1.0 mg/kg |
IV: Maintenance | 0.1 mg/kg | |
Vecuronium | IV: Intubation | 0.05-0.1 mg/kg |
IV: Maintenance | 0.02 mg/kg | |
Cisatracurium | IV: Intubation | 0.1-0.2 |
IV: Maintenance | 0.02-0.05 | |
Sugammadex | IV (train of four 1-2/4) | 2-4 mg/kg |
IV (profound paralysis) | 16 mg/kg |

Full access? Get Clinical Tree
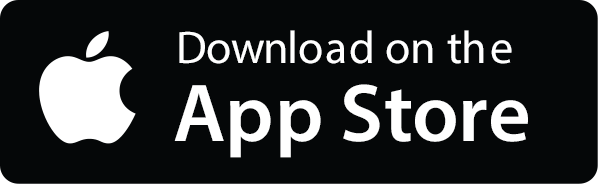
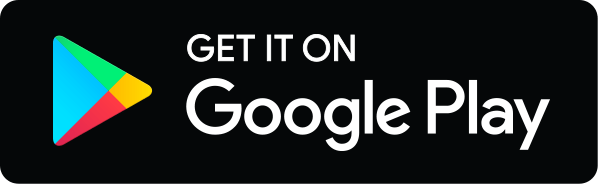
