Humans can hear sound waves with frequencies between 20 and 20,000 hertz. Frequencies higher than this range are called ultrasound (US). A sound wave can be described as a mechanical, longitudinal wave composed of cyclic compressions and rarefactions of molecules in a medium. This is in contrast to electromagnetic waves, which do not require a medium for propagation. Three acoustic variables identify sound waves:
Pressure: force within an area (measured in pascals)
Density: mass within a volume (measured in kg/cm3)
Distance: motion measured in length (e.g., millimeters, centimeters)
Three parameters can be used to describe the absolute and relative strength (“loudness”) of a sound wave:
Amplitude: The amount of change in one of the previously mentioned acoustic variables. Amplitude is equal to the difference between the average and the maximum values of an acoustic variable (or half the “peak-to-peak” amplitude).
Power: The rate of energy transfer, expressed in watts (joules/second). Power is proportional to the square of the amplitude.
Intensity: The energy per unit cross-sectional area in a sound beam, expressed in watts per square centimeter (W/cm2). This is the parameter used most frequently when describing the biological safety of US.
The operator can modify the three parameters described, but it should be noted that modifying these parameters is not the same as adjusting receiver gain, which is a postprocessing function.
Changes (usually in intensity) can also be expressed in a relative, logarithmic scale known as decibels (dB). In common practice, the lowest-intensity audible sound (10-12 W/M2) is assigned the value of 0 dB. An increase of 3 dB represents a two-fold increase in intensity, and an increase of 10 dB represents a ten-fold increase in intensity. This means that a sound with an intensity of 120 dB is 1 trillion (1012) times as intense as a sound of 0 dB.
Four additional parameters that are inherent to the sound generator (transducer) and/or the medium through which the sound propagates are also used. When referring to a single transducer (piezoelectric) element in a pulsed US system, these cannot be manipulated by the operator:
Period: The duration of a single cycle. Typical values for clinical US range from 0.1 to 0.5 microseconds (μs).
Frequency (f): The number of cycles per unit time. One cycle per second is one hertz (Hz). US is defined as a sound wave with a frequency greater than 20,000 Hz. Values that are relevant in clinical imaging modalities such as echocardiography and vascular ultrasound range from 2 to 15 megahertz (MHz).
Period and frequency are reciprocals. Period = 1/f.
Wavelength (λ): The distance traveled by sound in one cycle (0.1 to 0.8 mm).
Wavelength and frequency are inversely proportional and are related by propagation speed through the formula λ = c/f.
Propagation speed (c): The speed of sound in a medium, determined by the characteristics of the medium through which it propagates. Propagation speed does not depend on the amplitude or frequency of the sound wave. It is directly proportional to the stiffness and inversely proportional to the density of the medium.
Sound propagates at 1540 m/s for average human soft tissue, including heart muscle, blood, and valve tissue. Other useful values are 330 m/s for air and 4080 m/s for skull bone.
Continuous waves are not useful for structural imaging. Instead, US systems use brief pulses of acoustic signal. These are emitted from the transducer during the “on” time and are received during the “off” time. One pulse typically consists of three to five cycles.
Pulsed US can be described by five parameters (Fig. 1-1):
Pulse duration: The time a pulse is “on,” which is very short (0.5 to 3 μs).
Pulse repetition period: The time from the start of one pulse to the start of the next pulse, which includes the listening time. Typical values are 0.1 to 1 ms.
Spatial pulse length: The distance from the start to the end of a pulse (0.1 to 1 mm).
Duty factor: The percentage of time the transducer is actively transmitting US, usually 0.1% to 1%. This means that the transducer element acts as a receiver over 99% of the time.
Pulse repetition frequency (PRF): The number of pulses that occur in 1 second, expressed in Hz. PRF is reciprocal to pulse repetition period. Typical values are 1000 to 10,000 Hz (not to be confused with the frequency of the US within a pulse, which is many times greater).
PRF is inversely proportional to imaging depth. Because sound takes time to propagate, a deeper image requires more listening time. Therefore, with a deeper image, the transducer can emit fewer pulses per second. This concept will also be important for the discussion of Doppler ultrasound.
The relation between the depth of a reflector and the time it takes for an US pulse to travel from the transducer to the reflector and back to the transducer (time-of-flight) is called the range equation:
This allows the US system to calculate the distance to a certain structure by measuring only the time-of-flight. Assuming that soft tissue has a uniform propagation speed of 1540 m/s, or 1.54 mm/μs, this means that time-of-flight increases by 13 μs for every 1 cm of depth of the reflector. This value is important for imaging and for Doppler US.
The most important effect of a medium on the US wave is attenuation—the gradual decrease in intensity (measured in dB) of an US wave. Attenuation results from three processes:
Absorption: conversion of sound energy to heat energy.
Scattering: diffuse spread of sound from a border with small irregularities.
Reflection: return of sound to the transducer from a relatively smooth border between two media. It is reflection that is important for imaging.
Different tissues attenuate by different processes and at different rates:
Air bubbles reflect much of the US that engages them and appear very echo dense (bright). Because sound attenuates the most in air, information distal to an air bubble is often lost as a result.
Lung, being mostly air filled, causes much scatter and results in the most attenuation of US by tissue.
Bone absorbs and reflects US, resulting in somewhat less attenuation than lung.
Soft tissue and blood attenuate even less than bone.
Water attenuates sound very little, mostly by absorption, with very little reflection. It is therefore very echo lucent (appears black on images).
Within soft tissue, attenuation is proportional to both the US frequency and path length, and can be expressed by the following equation:
Therefore, one may conclude that high-frequency US has greater attenuation, has poorer penetration, and is less effective at imaging deeper structures.
Less than 1% of the incident US is usually reflected at the boundary between different soft tissues. The interfaces between air and tissue and between bone and tissue are strong reflectors and can result in several types of artifacts (see Chapter 6).
As the US beam strikes a boundary between two media, three phenomena may occur:
Reflection: can be further broken down into specular reflection and diffuse reflection or backscatter
Transmission
Refraction
Reflection of the transmitted US signal is the basis of US imaging. It can occur only if there is a difference in the acoustic impedance (measured in MRayls) between the two media, and is dependent on the angle of incidence of the US beam at the interface. Acoustic impedance is a property of the media, not of the US beam. It is directly proportional to both density and propagation speed of the material.
Specular reflectors have large, smooth surfaces, or have irregularities that are larger than the wavelength of the US beam. They are angle dependent, reflecting US best at normal incidence (90 degrees, or perpendicular to the boundary).
Scatter reflectors (the “signal” used in US imaging) have irregularities that are about the same size or smaller than the wavelength of US that strikes the boundary. Scatter reflectors are also not angle dependent. A special type of scattering is termed Rayleigh scattering, and this occurs when US strikes an object much smaller than the beam’s wavelength (such as a red blood cell). Sound is scattered uniformly in all directions.
Refraction is a process associated with transmission and refers to the change of wave direction upon crossing the interface between two media. Refraction can occur only when the propagation speeds in the two media are different and the incident angle is oblique (Fig. 1-2). Refraction is described by Snell’s law:
Thus, if the speed of sound in medium 2 is less than the speed of sound in medium 1, then the transmission angle is less than the incident angle. Similarly, if the speed of sound in medium 2 is greater than the speed of sound in medium 1, then the transmission angle is greater than the incident angle.
Because it violates the assumption that US travels in a straight line, refraction may result in image artifacts (e.g., a second copy of a true reflector).
Simply put, an ultrasound transducer (Fig. 1-3) is a device that converts electrical energy into high-frequency acoustic energy, and vice versa. US transducers contain crystals that change shape when an electrical potential is applied (reverse piezoelectric effect), as during sound transmission, and also create voltage when mechanically deformed (piezoelectric effect), as during sound reception. The most common crystals in US systems are composed of lead, zirconate, and titanate (PZT). The frequency of the US generated by each piezoelectric element is related to the thickness and the propagation speed of the crystal by the formula:

Full access? Get Clinical Tree
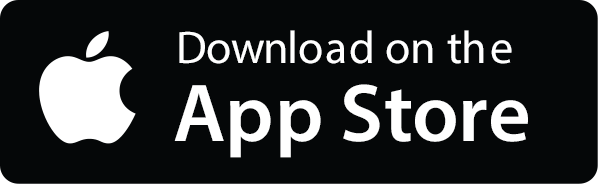
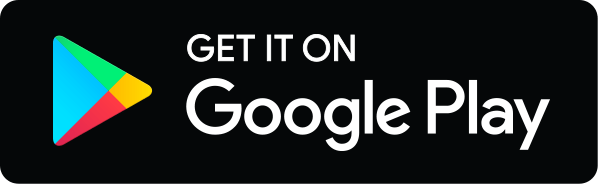
