Two-dimensional representation of atomic structure
Each atom has a nucleus (central core) of neutrons and protons, surrounded by a cloud of negatively charged electrons. Figure 25.3 shows the properties of these components. The charge of an atom is the number of protons minus the number of electrons. It is clear that almost all the mass of an atom is in the nucleus. The number of protons (the atomic number) defines the element, and the atomic mass is close to the combined masses of protons and neutrons in the atom. Elements may exist with different numbers of neutrons in the nucleus while having the same atomic number. These are called isotopes, and those that release particles (radioactive) are called radioisotopes. Carbon provides an example: it has atomic number 6, and therefore six protons, though 12C (carbon 12) has six protons and six neutrons, while 14C (carbon 14) has six protons and eight neutrons (radioactive). Conventionally, the mass number is shown as a prefix superscript and the atomic number is shown as a prefix subscript, for example for carbon and
for sodium (11 protons and 12 neutrons).
Nucleus | |||
---|---|---|---|
Proton | Neutron | Electron | |
Mass number | 1 | 1 | 1/1836 |
Charge | +1 | 0 | –1 |
Electrons are arranged in orbital shells around the nucleus, from the innermost K shell outwards to L, M, N, O, P and Q shells (Figure 25.4). These are not precise concentric rings, but the conceptual model helps to predict molecular behaviour. Shells are filled in their alphabetical order. Complete electron shells, pairs and octets of electrons confer stability. Each orbital shell has a maximum number of electrons, and some stability is conferred when this is achieved.
Shell | Maximum number of electrons |
---|---|
K | 2 |
L | 8 |
M | 18 |
N | 32 |
Valency
Valency may be defined as the number of atoms of hydrogen that one atom of an element can combine with or replace.
Each element has at least one valency state, and the valency is used to establish possible intermolecular bonds. Each group in the periodic table has a characteristic valency. For example, group IA elements have a valency of +1. The valency can be predicted from the electron shell configuration, as shown in Figure 25.5. Atoms of a particular element lose or gain electrons to achieve the stability described above. This loss or gain of electrons may be complete (in ionic bond) or by sharing with other atoms (in covalent bond). In this way, they adopt the electron configuration of the closest (by atomic number) rare or inert gas (for example helium, neon, argon). The inert gases are already at their most stable and do not gain or lose electrons. Consequently, they have minimal interaction with other atoms and exist as gases. Carbon is the single most important element in organic chemistry because it is chemically versatile – it can either lose or gain electrons to achieve electron stability.
Element | Protons | Neutrons | Electrons in shell | Valency | ||||
---|---|---|---|---|---|---|---|---|
K | L | M | N | O | ||||
Hydrogen | 1 | 0 | 1 | 1 | ||||
Helium | 2 | 2 | 2 | 0 | ||||
Carbon | 6 | 6 | 2 | 4 | 4 | |||
Nitrogen | 7 | 7 | 2 | 5 | 3 | |||
Oxygen | 8 | 8 | 2 | 6 | 2 | |||
Sodium | 11 | 12 | 2 | 8 | 1 | 1 | ||
Magnesium | 12 | 12 | 2 | 8 | 2 | 2 | ||
Chlorine | 17 | 18 | 2 | 8 | 7 | 1 | ||
Argon | 18 | 18 | 2 | 8 | 8 | 0 | ||
Potassium | 19 | 20 | 2 | 8 | 8 | 1 | 1 | |
Calcium | 20 | 20 | 2 | 8 | 8 | 2 | 2 | |
Xenon | 54 | 77 | 2 | 8 | 18 | 18 | 8 | 0 |
Ionic bonds
The ionic bond (also termed an electrostatic bond) relies on the fact that certain elements (termed electrovalent) have a tendency to lose or gain electrons to form charged atoms or molecules called ions. Like charges repel, but opposite charges attract, and therefore create a bond. This is illustrated by the formation of sodium chloride in Figure 25.6.
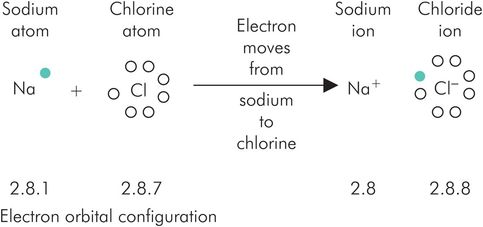
Ionic bonding illustrated by the formation of sodium chloride. In Figures 25.6, 25.7, 25.8 and 25.10 the colouring of electrons enables identification of their origin when considering the end result.
With respect to the process shown in Figure 25.6, the movement of an electron from the sodium atom to the chlorine atom results in both ions having stable outermost shells. The sodium ion now has the electron configuration of neon, and chloride that of argon. The resultant change in charge causes a strong attraction between the sodium and chloride ions (the ionic bond) which is sufficient to maintain the precise crystalline structure of solid sodium chloride. Figure 25.7 shows another example of ionic bonding with the relevant electron configurations.
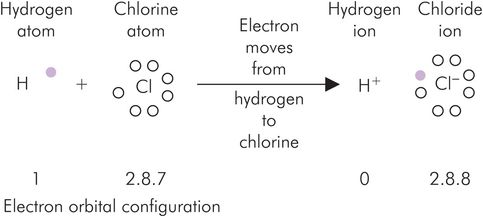
Ionic bonding illustrated by the formation of hydrochloric acid
Covalent bonds
Covalent bonds are similar to ionic bonds in that they depend on forming stable electron shells. In contrast with ionic bonding, the atoms share electrons rather than donate them completely. Carbon, nitrogen, hydrogen and oxygen frequently behave in this way. The electron configuration of carbon is 2.4 (see Figure 25.5). Stability is achieved either by losing four electrons or by gaining four electrons, resulting in a pair or an octet of electrons in the outer shell, respectively. Covalent bonds may be single, double or triple, depending on the number of electron pairs that are shared. Methane is an example of covalent bonding with four single covalent bonds (Figure 25.8). The carbon atom of methane adopts the electron configuration of neon (2.8) while hydrogen adopts the configuration of helium, both being inert gases with stable atomic configurations.
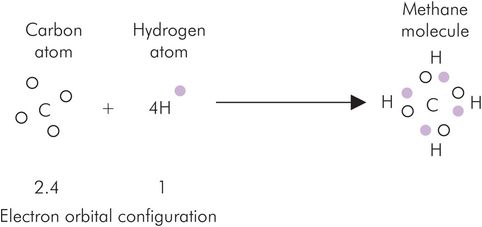
Covalent bonding illustrated by the formation of methane
A comparison of the properties of ionic and covalent bonds is given in Figure 25.9.
Ionic | Covalent |
---|---|
No sharing of electrons, therefore non-directional bond, therefore no particular shape | Electrons shared, therefore directional bond, therefore definite shape, so isomerism and stereoisomerism possible |
Usually solid (crystalline) | Usually highly volatile liquids or gases |
Not easily vaporised | Easily vaporised |
Fused state | |
Melt form is a conductor | Poor conductor |
Readily dissolves in water | Not readily soluble in water |
Forms electrolyte in water |
Dative bonds
The dative bond is a type of covalent bond in which both electrons of the shared pair are from the same atom. This bond is a feature of atoms that have complete pairs of electrons in their outer shells in the non-combined state. Typical examples are oxygen, nitrogen, phosphorus and sulphur. The formation of ammonium chloride from ammonia and hydrogen chloride illustrates this bond, as well as ionic and covalent bonds (Figure 25.10).
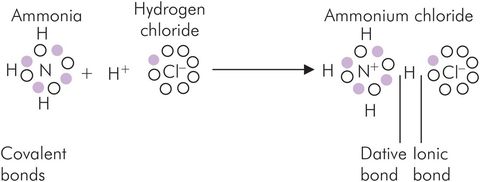
Mixed bonds illustrated by the formation of ammonium chloride
Van der Waals forces
Because electrons are not rigidly fixed relative to the nucleus, but move in characteristic orbitals, the resulting electron cloud has a characteristic shape. Highly electronegative atoms such as oxygen attract electrons so that the distribution of the electron cloud of the molecule is uneven. This produces dipoles where component atoms of a molecule are not electrostatically neutral. The value of these charges is much less than the +1 or –1 of ions, and the molecule may have a polarity. Van der Waals forces are the attraction and repulsion of these weakly charged areas to similar areas in neighbouring molecules. Graphite is an example of sheets of covalently bonded carbon in which the layers are held together by van der Waals forces, and they are also essential in receptor and enzyme bonding.
Hydrogen bonds
A hydrogen bond is a weak electrostatic bond between the positive nucleus of a covalently bonded hydrogen atom in one molecule and the unshared pair of electrons of a highly electronegative atom of another molecule. Oxygen, sulphur and nitrogen are highly electronegative atoms, and water provides an example of hydrogen bonding (Figure 25.11). The hydrogen bonding in water is responsible for the relatively high boiling point (compared with non-polar liquids) and the structure of ice crystals.
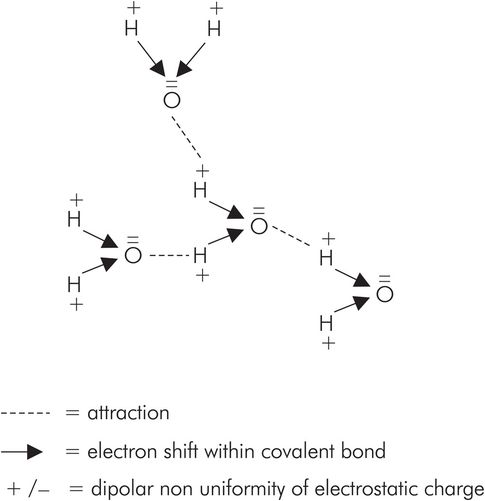
Hydrogen bonding in water. Dotted lines represent hydrogen bonds and arrows represent covalent bonds, with the arrow head showing direction of electron donation
Hydrophobic bonds
In water, individual molecules are attracted to each other by hydrogen bonds. Any molecules added to the water will disrupt this loosely held ‘structure’. If the electron distribution of the additive is not uniform (it has polarity) or if it is readily ionised, then it too will form bonds with the water molecules. This will allow it to spread readily and evenly throughout the containing vessel. If, however, the electron distribution is even, then the energy required to break the hydrogen bonds of water will be greater than that released by the formation of the new bonds. In this case, the most stable arrangement exists when the additive collects together, leaving as much of the water together as possible. This is easily seen when oil is added to water, and is a feature of lipids. Its density relative to water determines where the oil subsequently distributes. Vigorous mixing will provide the energy to break the hydrogen bonds and disperse the fat molecules, temporarily. This is the basis of the hydrophobic bond, which is very important physiologically, occurring at a local level around proteins and other molecules. Areas of membranes and proteins that do not have polarity do not attract water molecules. The water molecules therefore tend to maintain bonds with other water molecules, leaving these hydrophobic areas vacant. This promotes the movement of non-polar hydrophobic molecules to these sites. Looking at this situation in overview gives the impression that the hydrophobic areas and molecules are attracted to each other, but in fact there is little or no attraction at all between the two. It is, in effect, the result of displacement of the hydrophobic molecules by the attraction of water and other hydrophilic molecules.
Emulsions
Emulsions are used in pharmacology to provide solutions of fat-soluble drugs such as propofol. An emulsion is a mixture of two (or more) immiscible liquids. This means that one exists in the form of miscoscopic particles within the other. In propofol the drug is dissolved in the lipid of the emulsion. Using water alone provides insufficient propofol to achieve an effective dose (see Phenols in Chapter 30). In the case of propofol the lipid is contained within small envelopes (micelles) formed by a layer of molecules (e.g. phospholipid) that have both a hydrophilic end and a hydrophobic end (Figure 25.12).