Effect of drugs on the coagulation pathway
Anticoagulants
Anticoagulants are drugs that interfere with the process of fibrin plug formation, to reduce or prevent coagulation. This effect is used to reduce the risk of thrombus formation within normal vessels and vascular grafts. The injectable anticoagulants are also used to prevent coagulation in extracorporeal circuits and in blood product storage. There are two main types of anticoagulants: oral anticoagulants and injectable anticoagulants (heparins).
Oral anticoagulants
Oral anticoagulants inhibit the reduction of vitamin K. Reduced vitamin K is required as a cofactor in γ-carboxylation of the glutamate residues of the glycoprotein clotting factors II, VII, IX and X, which are synthesised in the liver. During this γ-carboxylation process, vitamin K is oxidised to vitamin K 2,3-epoxide. The oral anticoagulants prevent the reduction of this compound back to vitamin K in the liver, and they do this by virtue of their structural similarity to vitamin K. Their action depends on the depletion of these factors, which decline according to their individual half-lives (Figure 41.3).
Factor | Half-life (hours) |
---|---|
II | 60 |
VII | 6 |
IX | 24 |
X | 40 |
There are two groups of oral anticoagulants:
Coumarins (warfarin and nicoumalone)
Inandiones (phenindione)
Warfarin has the most widespread use. Phenindione is more likely to cause hypersensitivity, but is useful when there is intolerance to warfarin.
Warfarin sodium
Warfarin is administered orally as a racemic mixture. It is rapidly absorbed, reaching a peak plasma concentration within 1 hour with a bioavailability of 100%. However, a clinical effect is not apparent until the clotting factors become depleted after 12–16 hours, reaching a peak at 36–48 hours. Warfarin is 99% protein-bound (to albumin) in the plasma, resulting in a small volume of distribution. Warfarin is metabolised in the liver by oxidation (l-form) and reduction (d-form), followed by glucuronide conjugation, with a half-life of about 40 hours.
Warfarin crosses the placenta and is teratogenic during pregnancy. In the postpartum period it passes into breast milk, which is a particular problem as the gut flora responsible for producing vitamin K2 and hepatic function in the newborn are not fully developed.
Warfarin has a low therapeutic index and is particularly prone to interactions with other drugs. Interactions increasing the effect of warfarin occur in several ways:
Competition for protein binding sites
Increased hepatic binding
Inhibition of hepatic microsomal enzymes
Reduced vitamin K synthesis
Synergistic antihaemostatic actions
Drugs such as NSAIDs, chloral hydrate, oral hypoglycaemic agents, diuretics and amiodarone displace warfarin from albumin binding sites, resulting in higher free plasma levels and greater effect. This effect is made more significant because normally only 1% of warfarin is free and a small change in protein binding has a dramatic effect on free warfarin levels. d-Thyroxine increases the potency of warfarin by increasing hepatic binding. Ethanol ingestion may inhibit liver enzymes responsible for warfarin elimination. The effect of warfarin may also be increased by acute illness, low vitamin K intake and drugs such as cimetidine, aminoglycosides and paracetamol. Broad-spectrum antibiotics reduce the level of gut bacteria responsible for vitamin K2 synthesis, and may enhance the effect of warfarin where the diet is deficient in vitamin K. Other anticoagulants, and particularly antiplatelet drugs, increase the clinical effects of warfarin.
Interactions decreasing the effect of warfarin can occur in several ways, notably:
Induction of hepatic microsomal enzymes
Drugs that increase levels of clotting factors
Binding of warfarin
Increased vitamin K intake
The effect of warfarin may be reduced by induction of hepatic enzymes by barbiturates and phenytoin. Oestrogens increase the production of vitamin-K-dependent clotting factors (II, VII, IX, X). Cholestyramine binds warfarin, reducing its effect. Carbamazepine and rifampicin reduce the effect of warfarin, but the mechanism of this effect is not clear.
Heparins
Heparins are injectable anticoagulants that act by binding to antithrombin, resulting in a profound increase in antithrombin activity.
Structure
Heparins are a group of sulphated acid glycosaminoglycans (or mucopolysaccharides) comprising alternate monosaccharide residues of N-acetylglucosamine and glucuronic acid and their derivatives. The glucuronic acid residues are mostly in the iduronic acid form and some are ester-sulphated. The N-acetylglucosamine residues may be deacylated, N-sulphated and ester-sulphated in a random manner. This results in a chain of 45–50 sugar residues of variable composition based on the above units. The molecules are attached by the sulphated components to a protein skeleton consisting entirely of glycine and serine amino acid residues. The molecular weight of heparin ranges from 3000 to 40,000 daltons, with a mean of 12,000–15,000. Endogenous heparin is located in the lungs, in arterial walls and in mast cells as large polymers of molecular weight 750,000. It is present in the plasma at a concentration of 1.5 mg per litre.
Heparin has a strong negative charge and is a large molecule, so there is minimal absorption following oral administration. It is supplied as heparin sodium and heparin calcium.
Mechanism of action
Heparin has the following effects:
Inhibition of coagulation by enhancing the action of antithrombin on the serine protease coagulation factors (IIa, Xa, XIIa, XIa and IXa)
Reduced platelet aggregation
Increased vascular permeability
Release of lipoprotein lipases into plasma
The negatively charged heparin binds to the lysine residue in antithrombin, an α2-globulin, which in turns increases the affinity of the arginine site of antithrombin for the serine site of thrombin (factor II). This increases the inhibitory activity of antithrombin 2300-fold. This reversible bond is the feature of a specific antithrombin binding site comprising five particular residues. This particular pentasaccharide sequence is present randomly in about one-third of the heparin molecules. For full activity of heparin on thrombin (IIa), a heparin molecule must have at least 13 extra sugar residues in addition to the pentasaccharide antithrombin binding site sequence. The covalently bonded thrombin–antithrombin complex is inactive, but once it is formed the heparin is released and the complex is rapidly destroyed by the liver. The active heparin section is then free to act on more antithrombin. Heparin acts in a similar way on the other activated serine protease coagulation factors (XIIa, Xa and IXa). The binding of heparin to both the clotting factor and antithrombin is important in the above enhancement of antithrombin. The activity of heparin on factor Xa is also mediated by increasing the affinity of antithrombin for the clotting factor, but heparin does not bind to factor Xa. Factor Xa inhibition is enhanced with lower levels of heparin than those required for thrombin inhibition. Heparin reduces platelet aggregation secondary to the reduction in thrombin (a potent platelet aggregator). The increase in plasma lipase results in an increase in free fatty acid levels.

Full access? Get Clinical Tree
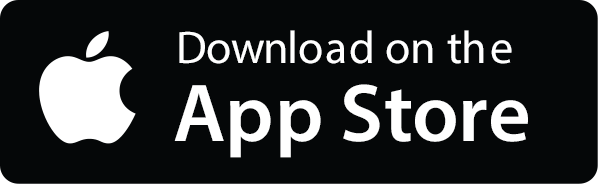
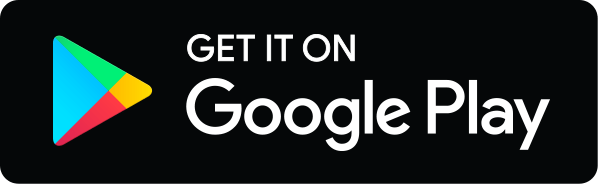