Key Points
- 1.
In patients, the observed acute effect of a specific anesthetic agent on the cardiovascular system represents the net effect on the myocardium, coronary blood flow (CBF), and vasculature; electrophysiologic behavior; and neurohormonal reflex function. Anesthetic agents within the same class may differ from one another quantitatively and qualitatively. The acute response to an anesthetic agent may be modulated by the patient’s underlying pathology or pharmacologic treatment, or both.
- 2.
Volatile agents cause dose-dependent decreases in systemic blood pressure. For halothane and enflurane, this mainly results from depression of contractile function, and, for isoflurane, desflurane, and sevoflurane, pressure changes result from decreases in systemic vascular responses. Volatile anesthetics cause dose-dependent depression of contractile function that is mediated at a cellular level by attenuating calcium currents and decreasing calcium sensitivity. Decreases in systemic vascular responses reflect various effects on endothelium-dependent and endothelium-independent mechanisms.
- 3.
Volatile agents determine CBF by their effect on systemic hemodynamics, myocardial metabolism, and coronary vasculature. When these variables were controlled in studies, the anesthetics exerted only mild direct vasodilatory effects on the coronary vasculature.
- 4.
In addition to causing acute coronary syndromes, myocardial ischemia can manifest as myocardial stunning, preconditioning, or hibernating myocardium. Volatile anesthetics can attenuate myocardial ischemia development through mechanisms that are independent of myocardial oxygen supply and demand and can facilitate functional recovery of stunned myocardium. Volatile agents also can simulate ischemic preconditioning, a phenomenon described as anesthetic preconditioning, the mechanisms of which are similar but not identical.
- 5.
Intravenous induction agents (ie, hypnotics) belong to various drug classes, including barbiturates, benzodiazepines, N-methyl- d -aspartate receptor antagonists, and α 2 -adrenergic receptor agonists. Although they all induce hypnosis, their sites of action and molecular targets are different, and their cardiovascular effects partially depend on the class to which they belong.
- 6.
Studies of isolated cardiac myocytes, cardiac muscle tissue, and vascular tissue have demonstrated that induction agents inhibit cardiac contractility and relax vascular tone by inhibiting the mechanisms that increase intracellular calcium ion (Ca 2+ ) concentration. This may be offset by mechanisms that increase myofilament Ca 2+ sensitivity in the cardiac myocyte and vascular smooth muscle, which can modulate cardiovascular changes. However, the cumulative effects of induction agents on contractility, vascular resistance, and vascular capacitance are mediated predominantly by their sympatholytic effects. Induction agents should be used judiciously and with extreme caution in patients with shock, heart failure, or other pathophysiologic circumstances in which the sympathetic nervous system is paramount in maintaining myocardial contractility and arterial and venous tone.
- 7.
Opioids have diverse chemical structures, but all retain an essential T-shaped component necessary stereochemically for the activation of the µ-, κ-, and δ-opioid receptors. These receptors are not confined to the nervous system and have been identified in the myocardium and blood vessels where endogenous opioid proteins can be synthesized.
- 8.
Acute exogenous opioid administration modulates many determinants of central and peripheral cardiovascular regulation. However, the predominant clinical effect is mediated by attenuation of central sympathetic outflow.
- 9.
Activation of the δ-opioid receptor can elicit preconditioning, which is mediated by signaling pathways that involve G-protein–coupled protein kinases, caspases, nitric oxide, and other chemicals. In contrast with ischemia in homeotherms, hibernation is well tolerated in certain species. This phenomenon may partially depend on mechanisms that are activated by opioids or opioid-like molecules.
An enormous body of literature has described the effects of different anesthetic agents on the heart and the pulmonary and systemic regional vascular beds. More publications have been spawned by the great interest in anesthesia-induced preconditioning (APC). In this chapter, volatile agents, intravenous anesthetics, and opioids are discussed in terms of their acute and delayed effects on the cardiovascular system (CVS). The acute effects on myocardial function, electrophysiology, coronary vasoregulation, systemic and pulmonary vasoregulation, and the baroreceptor reflex are described. Discussion of the delayed effects focuses on APC.
Volatile Agents
Acute Effects
Myocardial Function
The influence of volatile anesthetics on contractile function has been investigated extensively in several animal species and in humans using various in vitro and in vivo models. It is widely agreed that volatile agents cause dose-dependent depression of contractile function ( Box 7.1 ). Different volatile agents are not identical in this regard, and the preponderance of information indicates that halothane and enflurane exert equal but more potent myocardial depression than isoflurane, desflurane, or sevoflurane, in part because of reflex sympathetic activation with the latter agents. In the setting of preexisting myocardial depression, volatile agents have a greater effect than in normal myocardium. At the cellular level, volatile anesthetics exert their negative inotropic effects mainly by modulating sarcolemmal L-type Ca 2+ channels, the sarcoplasmic reticulum (SR), and contractile proteins. However, the mechanisms by which anesthetic agents modify ion channels are not completely understood.
All volatile anesthetic agents cause dose-dependent decreases in systemic blood pressure, which for halothane and enflurane predominantly result from attenuation of myocardial contractile function; and which for isoflurane, desflurane, and sevoflurane predominantly result from decreases in systemic vascular resistance.
Volatile agents obtund all components of the baroreceptor reflex arc.
The effects of volatile agents on myocardial diastolic function are not well characterized and await the application of emerging technologies that have the sensitivity to quantitate indices of diastolic function.
Volatile anesthetics lower the arrhythmogenic threshold to catecholamines. However, the underlying molecular mechanisms are not well understood.
When confounding variables are controlled (eg, systemic blood pressure), isoflurane does not cause coronary steal by a direct effect on coronary vasculature.
The effects of volatile agents on systemic regional vascular beds and on the pulmonary vasculature are complex and depend on many variables, including the specific anesthetic, precise vascular bed, and vessel size, and whether endothelial-dependent or endothelial-independent mechanisms are being investigated.
Cardiac Electrophysiology
Volatile anesthetics reduce the arrhythmogenic threshold for epinephrine. For volatile agents, the order of sensitization is halothane > enflurane > sevoflurane > isoflurane = desflurane. The molecular mechanisms underlying the effect of volatile anesthetics are poorly understood.
Coronary Vasoregulation
Volatile anesthetics modulate several determinants of myocardial oxygen supply and demand. They also directly modulate the myocyte’s response to ischemia.
The effect of isoflurane on coronary vessels was controversial and dominated much of the relevant literature in the 1980s and early 1990s. Several reports indicated that it caused direct coronary arteriolar vasodilatation in vessels with diameters of 100 µm or less and that isoflurane could cause coronary steal in patients with steal-prone coronary anatomy. Several studies in which potential confounding variables were controlled found that isoflurane did not cause coronary steal. Studies of sevoflurane and desflurane showed similar results that were consistent with a mild direct coronary vasodilator effect of these agents.
Systemic Vascular Effects
All volatile anesthetics decrease systemic blood pressure (BP) in a dose-dependent manner. With halothane and enflurane, the decrease in systemic BP primarily results from decreases in stroke volume (SV) and cardiac output (CO), whereas isoflurane, sevoflurane, and desflurane decrease overall systemic vascular resistance (SVR) while maintaining CO.
Baroreceptor Reflex
All volatile agents attenuate the baroreceptor reflex. Baroreceptor reflex inhibition by halothane and enflurane is more potent than that observed with isoflurane, desflurane, or sevoflurane, each of which has a similar effect. Each component of the baroreceptor reflex arc (eg, afferent nerve activity, central processing, efferent nerve activity) is inhibited by volatile agents.
Delayed Effects
Reversible Myocardial Ischemia
Prolonged ischemia results in irreversible myocardial damage and necrosis ( Box 7.2 ). Depending on the duration and sequence of ischemic insults, shorter durations of myocardial ischemia can lead to preconditioning or myocardial stunning ( Fig. 7.1 ). Stunning, first described in 1975, occurs after brief ischemia and is characterized by myocardial dysfunction in the setting of normal restored blood flow and by an absence of myocardial necrosis. Ischemic preconditioning (IPC) was first described in 1986 and is characterized by an attenuation of infarct size after sustained ischemia if the period of sustained ischemia is preceded by a period of brief ischemia ( Fig. 7.2 ). This effect is independent of collateral flow. Short periods of ischemia followed by reperfusion can lead to stunning or preconditioning with a reduction in infarct size.
Volatile anesthetic agents can attenuate the effects of myocardial ischemia (ie, acute coronary syndromes).
Nonacute manifestations of myocardial ischemia include hibernating myocardium, stunning, and preconditioning.
Halothane and isoflurane facilitate the recovery of stunned myocardium.
Preconditioning, an important adaptive and protective mechanism in biologic tissues, can be provoked by protean nonlethal stresses, including ischemia.
Volatile anesthetic agents can mimic preconditioning (ie, anesthetic preconditioning), which can have important clinical implications and provide insight into the cellular mechanisms of action of these volatile agents.
Anesthetic Preconditioning
Volatile agents can elicit delayed (ie, late) and classic (ie, early) preconditioning. APC is dose dependent, exhibits synergy with ischemia in affording protection, and perhaps not surprisingly in view of the differential uptake and distribution of volatile agents, requires different time intervals between exposure and the maintenance of a subsequent benefit that is agent dependent.
Volatile agents that exhibit APC activate mitochondrial K + ATP channels, and specific mitochondrial K + ATP channel antagonists block this effect. The precise contributions of sarcolemmal versus mitochondrial K + ATP channel activation to APC remain to be elucidated ( Fig. 7.3 ).
Volatile Agents
Acute Effects
Myocardial Function
The influence of volatile anesthetics on contractile function has been investigated extensively in several animal species and in humans using various in vitro and in vivo models. It is widely agreed that volatile agents cause dose-dependent depression of contractile function ( Box 7.1 ). Different volatile agents are not identical in this regard, and the preponderance of information indicates that halothane and enflurane exert equal but more potent myocardial depression than isoflurane, desflurane, or sevoflurane, in part because of reflex sympathetic activation with the latter agents. In the setting of preexisting myocardial depression, volatile agents have a greater effect than in normal myocardium. At the cellular level, volatile anesthetics exert their negative inotropic effects mainly by modulating sarcolemmal L-type Ca 2+ channels, the sarcoplasmic reticulum (SR), and contractile proteins. However, the mechanisms by which anesthetic agents modify ion channels are not completely understood.
All volatile anesthetic agents cause dose-dependent decreases in systemic blood pressure, which for halothane and enflurane predominantly result from attenuation of myocardial contractile function; and which for isoflurane, desflurane, and sevoflurane predominantly result from decreases in systemic vascular resistance.
Volatile agents obtund all components of the baroreceptor reflex arc.
The effects of volatile agents on myocardial diastolic function are not well characterized and await the application of emerging technologies that have the sensitivity to quantitate indices of diastolic function.
Volatile anesthetics lower the arrhythmogenic threshold to catecholamines. However, the underlying molecular mechanisms are not well understood.
When confounding variables are controlled (eg, systemic blood pressure), isoflurane does not cause coronary steal by a direct effect on coronary vasculature.
The effects of volatile agents on systemic regional vascular beds and on the pulmonary vasculature are complex and depend on many variables, including the specific anesthetic, precise vascular bed, and vessel size, and whether endothelial-dependent or endothelial-independent mechanisms are being investigated.
Cardiac Electrophysiology
Volatile anesthetics reduce the arrhythmogenic threshold for epinephrine. For volatile agents, the order of sensitization is halothane > enflurane > sevoflurane > isoflurane = desflurane. The molecular mechanisms underlying the effect of volatile anesthetics are poorly understood.
Coronary Vasoregulation
Volatile anesthetics modulate several determinants of myocardial oxygen supply and demand. They also directly modulate the myocyte’s response to ischemia.
The effect of isoflurane on coronary vessels was controversial and dominated much of the relevant literature in the 1980s and early 1990s. Several reports indicated that it caused direct coronary arteriolar vasodilatation in vessels with diameters of 100 µm or less and that isoflurane could cause coronary steal in patients with steal-prone coronary anatomy. Several studies in which potential confounding variables were controlled found that isoflurane did not cause coronary steal. Studies of sevoflurane and desflurane showed similar results that were consistent with a mild direct coronary vasodilator effect of these agents.
Systemic Vascular Effects
All volatile anesthetics decrease systemic blood pressure (BP) in a dose-dependent manner. With halothane and enflurane, the decrease in systemic BP primarily results from decreases in stroke volume (SV) and cardiac output (CO), whereas isoflurane, sevoflurane, and desflurane decrease overall systemic vascular resistance (SVR) while maintaining CO.
Baroreceptor Reflex
All volatile agents attenuate the baroreceptor reflex. Baroreceptor reflex inhibition by halothane and enflurane is more potent than that observed with isoflurane, desflurane, or sevoflurane, each of which has a similar effect. Each component of the baroreceptor reflex arc (eg, afferent nerve activity, central processing, efferent nerve activity) is inhibited by volatile agents.
Delayed Effects
Reversible Myocardial Ischemia
Prolonged ischemia results in irreversible myocardial damage and necrosis ( Box 7.2 ). Depending on the duration and sequence of ischemic insults, shorter durations of myocardial ischemia can lead to preconditioning or myocardial stunning ( Fig. 7.1 ). Stunning, first described in 1975, occurs after brief ischemia and is characterized by myocardial dysfunction in the setting of normal restored blood flow and by an absence of myocardial necrosis. Ischemic preconditioning (IPC) was first described in 1986 and is characterized by an attenuation of infarct size after sustained ischemia if the period of sustained ischemia is preceded by a period of brief ischemia ( Fig. 7.2 ). This effect is independent of collateral flow. Short periods of ischemia followed by reperfusion can lead to stunning or preconditioning with a reduction in infarct size.
Volatile anesthetic agents can attenuate the effects of myocardial ischemia (ie, acute coronary syndromes).
Nonacute manifestations of myocardial ischemia include hibernating myocardium, stunning, and preconditioning.
Halothane and isoflurane facilitate the recovery of stunned myocardium.
Preconditioning, an important adaptive and protective mechanism in biologic tissues, can be provoked by protean nonlethal stresses, including ischemia.
Volatile anesthetic agents can mimic preconditioning (ie, anesthetic preconditioning), which can have important clinical implications and provide insight into the cellular mechanisms of action of these volatile agents.
Anesthetic Preconditioning
Volatile agents can elicit delayed (ie, late) and classic (ie, early) preconditioning. APC is dose dependent, exhibits synergy with ischemia in affording protection, and perhaps not surprisingly in view of the differential uptake and distribution of volatile agents, requires different time intervals between exposure and the maintenance of a subsequent benefit that is agent dependent.
Volatile agents that exhibit APC activate mitochondrial K + ATP channels, and specific mitochondrial K + ATP channel antagonists block this effect. The precise contributions of sarcolemmal versus mitochondrial K + ATP channel activation to APC remain to be elucidated ( Fig. 7.3 ).

Full access? Get Clinical Tree
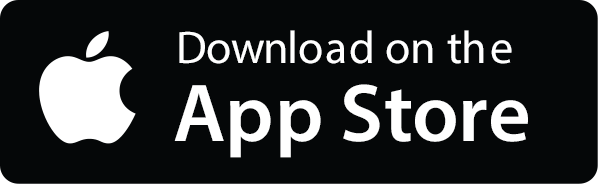
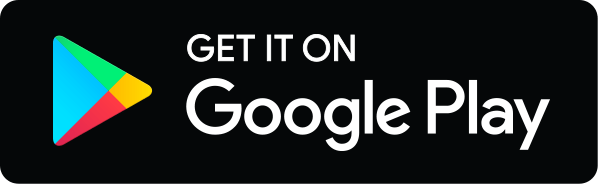
