Abstract
Plasma expanders are colloidal solutions that expand the circulating blood volume more than isotonic crystalloids and are among the most commonly used fluids in critical care and perioperative medicine worldwide. We begin our review of these agents by introducing the modified Starling model, which incorporates the fundamental physiologic role of the endothelial glycocalyx in transcapillary fluid movement. Next, we individually discuss each fluid, starting with their relevant pharmacological properties before delving into the trials on their clinical use. Human trial data on gelatins and dextrans are scarce, making their routine use difficult to justify outside resource-poor settings. Trials on the use of hydroxyethyl starch in critical care imply increased risk of kidney injury, coagulopathy and, possibly, mortality; whether these harms apply to the surgical setting remains an area of debate and ongoing study, but there is no compelling evidence to support the routine use of hydroxyethyl starch in the operating room. Multiple trials suggest that albumin is a safe, albeit more expensive, alternative to crystalloid use in the intensive care unit, with secondary analyses suggesting harm in traumatic brain injury and benefit in septic shock. Finally, more data are required to better define the role of albumin in perioperative medicine.
After reading this article, you should be able to:
- •
describe the role of the endothelial glycocalyx in the physiology of transcapillary fluid movement and how it mediates the possible benefits and limitations of colloid use
- •
compare the pharmacologic properties of the four most commonly used plasma expanders and relate these properties to their clinical use
- •
summarize the clinical trial data on the use of the four most commonly used plasma expanders in critical care and perioperative medicine and determine the appropriate clinical roles and possible adverse effects of each fluid in both settings
Introduction
Plasma expanders are colloidal solutions that expand the circulating blood volume more than isotonic crystalloids. In a 2010 cross-sectional study of 391 intensive care units (ICUs) across 25 countries, colloids were used more commonly overall than crystalloids for resuscitation, with considerable variation in the specific fluids used by country. This review will focus on the four most common types of colloidal plasma expanders used in critical care and perioperative medicine: albumin, gelatins, hydroxyethyl starch (HES) and dextrans. The properties of some of the available agents are shown in Table 1 and Figure 1 .
Plasma expander | Composition | MWw (kDa) | MWn (kDa) | Intravascular T1/2 (hours) | Volume effect (hours) | Maximum daily dose |
---|---|---|---|---|---|---|
Albumin 4.5% | Protein | 69 | 69 | >24 | – | – |
6% Hetastarch (450/0.7) | Amylopectin derivative | 450 | 70 | >24 | 5–6 | 20 ml/kg (max. 1.5 litres) |
6%/10% Pentastarch (200/0.5) | Amylopectin derivative | 200 | 60 | 10 | 3–4 | (6%) 33 ml/kg (max. 2.5 litres) (10%) 20 ml/kg (max. 1.5 litres) |
6% Tetrastarch (130/0.4) | Amylopectin derivative | 130 | – | – | 2–3 | 50 ml/kg |
6% Dextran 70 | Polysaccharide | 70 | 39 | 6–8 | 5 | 1.5 g/kg |
10% Dextran 40 | Polysaccharide | 40 | – | 5 | 3–4 | 1.5 g/kg |
4% Gelofusine | Protein | 30 | 23.2 | 1–3 | 1–3 | – |
3.5% Haemaccel | Protein | 35 | 24.5 | 1–3 | 1–3 | – |
HyperHAES | 7.2% NaCl þ 6% HES 200 | 200 | 60 | – | 1 | 4 ml/kg as single dose |
RescueFlow | 7.5% NaCl þ 6% dextran 70 | 70 | 39 | – | 1 | 4 ml/kg as single dose |

Physiologic principles of colloid use
Colloids are held in solution by polar covalent bonds that attract water molecules. The effect of colloids on plasma volume was traditionally explained via the classical Starling model of hydrostatic and oncotic pressures which determine the rate of transcapillary fluid movement (see Figure 2 a). In this model, large organic molecules such as colloids do not cross the vascular endothelium easily, thus exerting oncotic pressure (also known as colloid osmotic pressure), attracting extravascular fluid into the circulation. In general, the oncotic pressure and initial volume expansion produced by a colloid is proportional to the number of molecules or ions of solute. A corollary of the classic Starling model is that colloid use carries a lower risk of pulmonary or peripheral edema than crystalloid, though data to support this theory are limited and may not apply to critically ill patients. In fact, a variety of clinical trials in critical care and perioperative medicine have demonstrated that the difference in fluid balance achieved by use of colloids over crystalloids is generally fairly modest and often transient.

These discrepancies have led to the development of a revised Starling model, which incorporates the role of the endothelial glycocalyx in transcapillary fluid flow (see Figure 2 b). , The glycocalyx consists of an inner, dense matrix layer of membrane-attached glycoproteins that serves as the primary selective barrier to plasma macromolecules. It is the low oncotic pressure of the sub-glycocalyx space, not the interstitium, that is the principal determinant of transcapillary fluid flow. Furthermore, interstitial fluid is returned to the circulation primarily via the lymphatic system rather than by reverse transcapillary flow driven by plasma oncotic pressure. In this model, the increased capillary permeability and fluid extravasation characteristic of sepsis, burns and polytrauma are explained by damage to the glycocalyx barrier. This model also nicely explains why isolated hypoalbuminemia, even if moderately severe, rarely produces peripheral edema. Finally, though data to support this theory derive primarily from animal or in vitro studies, the clinical benefit derived from colloid use may be due to said colloid’s ability to restore the integrity of, and/or prevent damage to, the glycocalyx and thereby restore microcirculatory function.
Pharmacologic principles of colloid use
Non-albumin colloids are a dispersion of organic molecules of variable molecular weight (MW). As such, colloids have a weight-averaged molecular weight (MWw; arithmetic mean) that differs from the number-averaged molecular weight (MWn; median), with the exception of albumin solution as the albumin molecules have the same MW (i.e. MWw = MWn). In general, MWw determines the viscosity of a solution while MWn correlates more closely with duration of action.
Protein colloids
Albumin
Pharmacology: Albumin is the predominant naturally occurring plasma protein. Human albumin solution (HAS) is derived from pooled human plasma, serum, or normal placentas and is sterilized by heating and ultrafiltration to prevent disease transmission. Because of concerns related to prion transmission, HAS is sourced from the U.S. HAS is prepared from whole blood but contains no clotting factors, blood group antibodies, or plasma cholinesterases; therefore, blood grouping is not required.
The MW of HAS is approximately 69 kDa. Iso-oncotic (4–5%) and concentrated solutions (20–25%) are available. Infusion of 100 mL 25% HAS typically increases intravascular volume by 450 mL, but this expansion depends on blood volume, serum protein concentration, capillary permeability and extravascular water availability. In addition to being the primary determinant of oncotic pressure, the clinical effects of albumin administration may also be mediated by restoration of the damaged glycocalyx. Indeed, animal models suggest that albumin is superior to saline or HES in stabilizing an injured glycocalyx.
Side effects of HAS are rare; the incidence of anaphylactoid reactions is approximately 1 in 1000.
Clinical use:
Albumin in the ICU – The use of albumin in the ICU has been studied in three large multicenter randomized controlled trials (RCTs). The 2004 Saline versus Albumin Fluid Evaluation (SAFE) trial randomized 6997 ICU patients to receive albumin or saline for fluid resuscitation and found no difference in rates of mortality, ICU or hospital length of stay (LOS), rates of organ failure, or duration of renal replacement therapy (RRT) or mechanical ventilation. In predefined subgroup analyses, there were trends with albumin use towards decreased mortality in patients with severe sepsis and increased mortality in those with trauma. A post-hoc subgroup analysis of the SAFE study revealed a statistically significant increase in mortality in 460 patients with traumatic brain injury (TBI), an effect which was attributed to increased intracranial pressure. This has led to the recommendation to avoid albumin, especially hypo- or iso-oncotic albumin, in TBI.
More recently, the 2014 Albumin Italian Outcome Sepsis (ALBIOS) trial randomized 1818 ICU patients with severe sepsis or septic shock to crystalloid or 20% albumin (titrated to a serum albumin of ≥3.0 g/dL) until ICU discharge or up to 28 days. The primary outcome, 28-day mortality, was not different in either study arm but there was a decrease in duration of vasopressor use in the albumin arm. A post-hoc subgroup analysis also revealed a statistically significant improvement in 90-day mortality in the 1121 patients with septic shock.
The third RCT, the French Early Albumin Resuscitation During Septic Shock (EARSS) study, has been published thus far only in abstract form. It randomized 798 patients with septic shock to 100 mL of 20% albumin every 8 hours for 3 days or to the same volume of saline and found no difference in the primary outcome of all-cause 28-day mortality and multiple secondary outcomes apart from an increase in days without catecholamine support in the albumin arm.
Despite these three individual RCTs each having negative primary mortality outcomes, most (but not all) meta-analyses that have included these trials have concluded that albumin use is associated with reduced mortality in sepsis. Additional RCTs evaluating the role of albumin in the treatment of sepsis, including specifically albumin in early sepsis or albumin as endothelial rescue therapy, are ongoing ( NCT01337934 , NCT00819416 , NCT03654001 , NCT03869385 ).
Of note, albumin has been validated in the treatment of liver disease. Specifically, when added to antibiotic therapy, albumin has been shown to decrease the rates of renal dysfunction or death from spontaneous bacterial peritonitis. Likewise, albumin has been shown to decrease the rates of renal dysfunction, hyponatremia or circulatory dysfunction after large-volume (i.e. ≥5-litre) paracentesis. Albumin is often first-line therapy in the treatment of renal dysfunction in patients with advanced liver disease, as a lack of response to volume expansion with albumin is part of the diagnostic criteria of hepatorenal syndrome. There have been two small RCTs which, along with a subgroup analysis of the SAFE trial, suggest that adding albumin to furosemide (frusemide) can be useful for augmenting diuresis and improving oxygenation in acute lung injury without any impact on mortality, but similar trials of albumin use to enhance diuresis in nephrotic syndrome and cirrhosis have resulted in modest or no benefit on fluid balance. Finally, albumin is also considered the replacement fluid of choice for therapeutic plasma exchange.
Apart from these specific indications, currently available data demonstrate that albumin is a safe alternative to crystalloids in ICU patients, with some suggestive but inconclusive data that it may be particularly beneficial in patients with sepsis. However, there are no compelling data of benefit to justify the routine use of albumin over crystalloid given its increased cost.
Albumin in the OR – In a 2005 study of 13 Canadian hospitals, general surgery was the third most common specialty utilizing HAS, after nephrology and internal medicine. Many extrapolate the findings of critical care trials to conclude that albumin is the safest colloid to use perioperatively, a conclusion which is supported by two meta-analyses of from the early 2000s. However, concerns regarding other colloids, namely the risk of acute kidney injury (AKI), impaired hemostasis, and mortality, have also been raised about the perioperative use of albumin. Unfortunately, data specific to the use of albumin in the OR is limited to observational studies and small single-center RCTs of varying designs, comparator fluids, and populations, making firm conclusions elusive.
The RCT data comparing the use of albumin to other fluids in the OR include four small trials in renal transplant ( n = 44), cystectomy ( n = 40, 100), and major GI surgery ( n = 127) in which there were no differences in outcomes (blood loss, renal/graft function, or hospital LOS). In contrast, in a 2015 retrospective multivariate analysis of over one million elective arthroplasty cases in the US, the use of albumin compared to crystalloid was associated with an increased risk of AKI, thromboembolism, ICU admission, and cardiac or pulmonary complications.
Albumin has traditionally been considered the colloid of choice in cardiac surgery based on a 2003 multivariate analysis of 19,578 coronary bypass (CABG) patients in which the use of albumin versus starch or crystalloid was associated with a 25% relative and 0.5% absolute risk reduction for death. However, subsequent observational studies and small ( n = 220–240) RCTs in adults have had conflicting results, with variable impacts on rates of blood transfusion, AKI, RRT, ischemic events, and death. Small RCTs ( n = 60–119) comparing albumin and HES as either resuscitation or cardiopulmonary bypass priming fluid in pediatric cardiac surgery showed no difference in outcomes.
Liver transplantation is another surgery in which albumin is felt by some to be superior, but the literature to support its use, primarily small RCTs or observational studies, have had equivocal or conflicting results.
Overall, the mixed data suggest that albumin may be an acceptable fluid alternative in the perioperative setting, but larger prospective trials are needed before it can be routinely recommended over less expensive crystalloids.
Gelatins
Pharmacology: Gelatins are polypeptides (MWw 30–35 kDa) produced by hydrolysis of collagen from cattle or other animals. Further modification produces the final products, including urea-linked gelatins (e.g. Haemaccel) and succinylated or modified fluid gelatins (e.g. Gelofusine). These agents are pyrogen- and preservative-free. Gelatins are rapidly removed by glomerular filtration and protease catabolism within the reticuloendothelial system (RES) and therefore their intravascular half-life (1–3 hours) is significantly shorter than the other colloids.
Clinical use: Though in use for over a half-century, data on the risks and benefits of gelatins are relatively scant, with no large RCTs available. Their primary advantage stems from their low cost and stability at room temperature for up to 2–3 years. The various types of gelatins have variable effects on in vitro whole blood coagulation, with Gelofusine (relative to Haemaccel) shown to increase the time to peak clot strength formation, reduce the rate of fibrin formation, and inhibit platelet aggregation. Overall, limited data in humans suggest that gelatins are less likely to cause coagulopathy than HES and the effect on coagulation parameters appears not to translate into increased need for transfusion. Gelatins were traditionally thought to not cause renal dysfunction, though observational studies and recent small RCTs in surgical patients suggest the opposite.
Severe anaphylactoid reactions were traditionally reported at 1 in 350–2000 for urea-linked solutions and 1 in 1800–13,000 for succinylated preparations, but newer data suggest severe reactions may be underreported as many reactions present in a delayed fashion. A recent meta-analysis of 60 studies found a statistically significant increase in risk of anaphylaxis compared to crystalloid or albumin. Overall, gelatin preparations have the highest risk of anaphylactoid reactions among the four major colloid types. For this reason, gelatins have been withdrawn from the U.S. market.
A 2018 Cochrane Systematic Review of colloid use in the ICU identified six RCTs comparing the use of gelatin versus crystalloid (totaling 1698 patients with 407 receiving gelatin) and found no difference in the rates of death, RRT, need for transfusion, or serious reactions, but these conclusions were considered of low or very low level of certainty. There are no compelling data to currently recommend the use of gelatins over other fluids in the ICU or perioperative settings, but the ongoing Gelatin in ICU and Sepsis (GENIUS) trial (NCT02715466) may provide new useful data.
Non-protein colloids
Hydroxyethyl starch
Pharmacology: HES is derived from amylopectin, a branched natural polymer of glucose which is derived from waxy maize or potato starch. Amylopectin is rapidly hydrolyzed in vivo by amylase; therefore, HES molecules are stabilized by substituting hydroxyethyl groups for hydroxyl groups at the C2, C3 and C6 positions of the glucose residues. The multibranched nature of HES results in a globular structure and lower viscosity that are more similar to albumin than other synthetic colloids.
HES is available as 3, 6 and 10% solutions, with varying MWw: low (70 kDa), medium (130–270 kDa) and high (≥450 kDa). Molar substitution (MS) is the ratio of substituted hydroxyethyl groups and the total number of glucose units, ranging from low MS tetrastarch (0.4) or pentastarch (0.5) to high MS hexastarch (0.6) or hetastarch (0.7). By convention, HES is classified by adding the suffix MWw/MS; e.g. 6% HES 130/0.4 is a 6% solution of HES with a MWw of 130 kDa and MS of 0.4.
Hydroxyethyl residues at C2 very effectively hinder hydrolysis. A high C2:C6 ratio and a high MS both prolong the duration of action of HES. HES has a water-binding capacity of 20–30 mL/g and the volume effect varies from up to 150% for hyperoncotic 10% HES 200/0.5 to about 100% for 6% HES 130/0.4. Small HES molecules (<60 kDa) are rapidly cleared in urine whereas larger fragments first undergo hydrolysis by amylase before being excreted renally within 24 hours. A fraction of HES enters the RES, interstitium, and tissues; this fraction was initially felt to be quite small for modern lower-MWw HES, though further data suggest this may not be the case.
In animal studies, HES has been found to attenuate capillary leakage during inflammation, restore macrophage function after major hemorrhage, and improve microcirculatory oxygen flux by reducing blood viscosity. High-MS HES impairs coagulation by reducing the concentration of factor VIII and von Willebrand factor and decreasing platelet activity via glycoprotein IIb-IIIa receptor blockade, effects which translate into clinically significant bleeding. HES 130/0.4 causes measurable but smaller coagulation defects and less bleeding, comparable to or less than that of albumin. Most clinical studies of HES 130/0.4 have reported no differences, but some small RCTs have still noted increased estimated blood loss relative to crystalloid. Low-MS starch causes latent, dose-dependent itching typically of the upper torso due to tissue sequestration. The incidence of severe anaphylactoid reactions is 1 in 5000–16,000, the lowest of the four major colloid types.
HES has also been associated with AKI via a process termed osmotic nephrosis characterized by vacuolization, swelling, and colloid accumulation in renal tubular cells. Nephrotoxicity was first linked to high-MS high-MWw HES, but has subsequently been found to occur in at-risk paints with the modern low-MS lower-MWw HES formulations.
Clinical use:
HES in the ICU – The use of HES in the ICU has been studied in multiple large multicenter RCTs. The first was the 2008 Efficacy of Volume Substitution and Insulin Therapy in Severe Sepsis (VISEP) trial, in which 537 patients were randomized to 10% pentastarch (‘second-generation’ HES 200/0.5) or modified lactated Ringers solution (LR) for resuscitation. The trial was ultimately stopped early as HES use resulted in increased rates of coagulopathy, red cell transfusion, AKI, and RRT use and a trend towards higher 90-day mortality.
Subsequently, two trials were published in 2012 on the use of ‘third-generation’ HES. First, the Scandinavian Starch for Severe Sepsis/Septic Shock (6S) trial randomized 804 patients with severe sepsis to resuscitation with HES 130/0.42 or Ringer’s acetate, and the HES group had statistically significant increases in 90-day mortality, need for RRT, and bleeding. Subsequently, the Crystalloid versus Hydroxyethyl Starch Trial (CHEST) randomized 7000 ICU patients to resuscitation with 6% HES 130/0.4 or saline until ICU discharge or up to 90 days. Though 90-day mortality rates were similar and the HES group had lower rates of shock, HES use resulted in increased rates of AKI, RRT, hyperbilirubinemia, rash, and pruritus.
The most recent RCT of HES use in the ICU was the 2013 Colloids versus Crystalloids for the Resuscitation of the Critically Ill (CRISTAL) trial, which randomized 2857 ICU patients with hypovolemic shock, over half with concurrent sepsis, to resuscitation with either colloids or crystalloids. The RCT had a pragmatic design allowing for the unblinded use of any locally available colloid or crystalloid, with the most commonly used crystalloid (in 86%) being saline and the most commonly used colloid (in 69%) being HES. There was no difference in the primary outcome of all-cause mortality at 28 days, although colloids were associated with a statistically significant reduction in secondary outcomes including days alive without vasopressor support or mechanical ventilation and all-cause 90-day mortality.
However, though controversial, most experts do not interpret the favorable secondary outcomes of the CRISTAL trial as sufficient evidence to support the use of HES in the ICU. A 2018 systematic Cochrane review identified 24 RCTs with 11,177 patients comparing the use of starches versus crystalloid and found no significant difference in mortality but increases in need for transfusion, need for RRT, pruritus, and rash with HES. In 2013, both the FDA and European Medicines Agency issued warnings against the use of HES in patients with critical illness, renal dysfunction, burns, or sepsis. Though the decisions to restrict HES use were not without controversy, virtually all experts agree that HES should not be given to critically ill patients.
HES in the OR – While some have recommended avoiding perioperative HES, based in part on the critical care trials referenced above, others have argued that there is a role for the protocolized use of third-generation HES at lower doses and for shorter durations in selected surgical patients.
Previously, RCTs on the use of 6% HES 130/0.4 versus other fluids in non-cardiac surgery had been largely limited to approximately a dozen small ( n = 21–202) RCTs and several meta-analyses which generally reported insufficient data or minimal impact on outcomes, apart from two RCTs with increased blood loss.
A notable exception is a 2018 RCT of 160 major abdominal surgeries which compared 6% HES 130/0.4 to PlasmaLyte, both delivered by an automatic ‘closed-loop’ system based on non-invasive cardiac output monitoring. HES resulted in a decrease in the primary composite outcome of postoperative morbidity measured at postoperative day 2, driven primarily by decreased vasopressor use and fewer anastomotic leaks.
However, a 2019 trial of 1057 moderate-to high-risk abdominal surgeries, which randomized patients to receive intraoperative 6% HES 130/0.4 or LR by an algorithm based on stroke volume as estimated by esophageal Doppler probe, found no difference in the primary outcomes of serious postoperative complications or renal function.
Most recently, the Fluid Loading in Abdominal Surgery: Saline versus Hydroxyethyl Starch (FLASH) trial was published in January 2020. The multicenter double-blind RCT randomized 818 patients undergoing abdominal surgery to resuscitation with 6% HES 130/0.4 versus 0.9% saline by a hemodynamic algorithm targeting stroke volume during surgery and up to 24 hours postoperatively. Apart from a 250-mL difference in median amount of fluid required on day 1, a difference which was gone by day 2, there was no difference in the primary outcome, a composite of death or major postoperative complications at 14 days (in which there was a trend towards harm with HES), or in any prespecified secondary outcome. In post-hoc analyses, HES was statistically associated with increased intraoperative transfusions and AKI by day 28. As such, some have interpreted the FLASH trial as evidence against the use of HES in the OR.
Importantly, cardiac surgery carries a substantially higher risk of AKI than most types of non-cardiac surgery. There is significant debate about whether HES is safe to use in cardiac surgery, but the available data comparing HES to other fluids are limited to small trials, observational studies, and meta-analyses that have been contradictory, with two studies showing no difference, one showing decreased blood loss, and three others showing harm from HES with an increase in bleeding or the need for transfusion, reoperation, and/or RRT.
Likewise, the data on HES use in pediatric surgery are limited, with six RCTs comparing HES versus albumin or crystalloid without significant differences in efficacy or adverse effects, with the exception of some increased need for transfusion with HES use in two of the studies.
Overall, the data do not support the perioperative use of HES. The bulk of data on HES in non-cardiac surgery demonstrates no benefit with a suggestion of harm. In cardiac surgery, the data are even poorer, but there is also no clear signal of benefit; if anything, the potential for AKI is likely to be higher in this high-risk population. Certainly, perioperative HES, if used at all, should be restricted to non-septic patients without pre-existing renal dysfunction. Two ongoing RCTs of HES in trauma (NCT03338218) and abdominal surgery (NCT03278548) patients may provide further clarity.
Dextrans
Pharmacology: Dextrans are highly branched polysaccharides produced by the bacterium Leuconostoc mesenteroides acting on sucrose. Acid hydrolysis and subsequent ethanol fractionation produce the final products: dextran 40 (MWw = 40 kDa) and dextran 70 (MWw = 70 kDa). Dextran 40 and 70 bind 30 mL/g and 20–25 mL/g of water, respectively. The kidneys rapidly eliminate 70% of dextran 40 and 50% of dextran 70 unchanged, while the remainder is metabolized to glucose. A small proportion of dextran enters the RES via the interstitium or is eliminated via the gut. The intravascular half-life is determined by particle size, with dextran 70 lasting longer than dextran 40. Dextran produces similar microcirculatory effects to HES, attenuating ischemia-reperfusion injury in rodents by reducing activated leukocyte–endothelial interaction. Dextran solutions have similar effects on coagulation to HES, promoting fibrinolysis and inhibiting platelet aggregation, which limits their use to 1.5 g/kg/day. AKI caused by renal tubular obstruction or osmotic nephrosis has been associated with dextran, particularly in hypovolemia, oliguria, or preexisting renal dysfunction.
Clinical use: The primary advantages of dextrans are low cost and prolonged stability at room temperature. Dextran use is uncommon in high income countries and is generally declining worldwide. Dextrans have been withdrawn from the market in multiple countries. Clinical trials on the use of dextrans in the ICU or the OR are limited, with many studies being small, low quality, and/or published before 2000. The 2018 systematic Cochrane review identified 19 studies with 4736 participants comparing dextrans to crystalloid and found no impact on mortality, but they were unable to come to any conclusions about the impact on bleeding, RRT, or severe reactions. Overall, there is a lack of evidence to support the use dextrans in the ICU.
However, anaphylactoid reactions to dextran are considered relatively common (up to 1 in 500–2000), though can be decreased significantly by hapten prophylaxis and are anecdotally less common in patients with preexisting shock. Dextrans can also interfere with erythrocyte cross-matching.
Dextrans have traditionally been used in reconstructive microsurgery, in which the antithrombotic and antiplatelet effects were thought to help prevent thrombosis of microvascular anastomoses, though this practice has largely fallen out of favor and been replaced with traditional antiplatelet or antithrombotic therapy due to the risks associated with dextran use.
Conclusions
In conclusion, there are no compelling data to recommend the routine use of colloid plasma expanders over crystalloids in either critical care or perioperative medicine. While the possible benefit of plasma expansion with lower volumes can be readily demonstrated in healthy subjects, this effect is significantly reduced in acute illness or major surgery in which fluid extravasation occurs primarily due to disruption of the normal endothelial glycocalyx rather than changes in oncotic pressure. The safest colloid appears to be albumin, the most expensive agent. The suggestion of benefits with albumin use, especially in sepsis, noted in some secondary analyses require confirmation from large ongoing RCTs. Likewise, trials are needed to define the role of albumin in perioperative medicine. HES, in contrast, appears to be harmful to critically ill patients due to increased rates of AKI, coagulopathy and, possibly, death. In surgical patients, though the overall risk of harm may be lower than in ICU patients, HES has no clear benefit and may still carry an increased risk of AKI or bleeding. Finally, data to support the use of gelatins and dextrans are highly limited and both carry higher risks of anaphylaxis than other fluids. With the possible exception of patients unresponsive to crystalloids in resource-poor settings, the use of HES, dextrans, or gelatins is presently hard to justify outside of clinical trials.
References

Full access? Get Clinical Tree
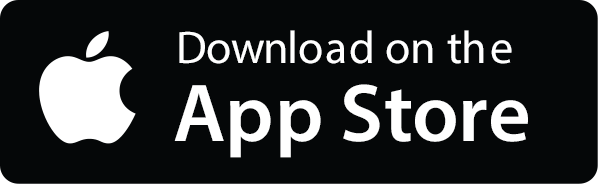
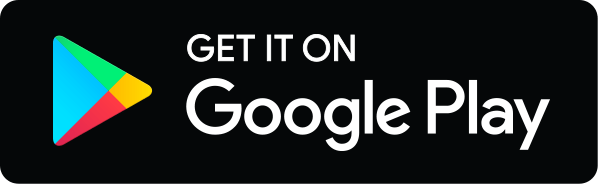
