Abstract
CRPS patients are routinely prescribed a combination of interventional procedures, physical therapy, antidepressants, antiinflammatory medications, antiepileptics, and opiates. Historically, limited options existed when these modalities fail. Recently, there is heightened interest in intravenous ketamine, bisphosphonates, calcitonin, magnesium, and free radical scavengers for refractory CRPS. Several studies have produced promising results, but their utility is limited by the lack of consistent diagnostic criteria for CRPS. While ketamine has analgesic properties in the acute setting, its pain relief in CRPS is likely secondary to its neuromodulatory effects, possibly through desensitization of the NMDA receptor within the spinal cord or via restoration of inhibitory sensory control within the brain. Consistent with this physiology, magnesium also offers analgesic properties as an NMDA receptor blocker and thus stabilizes abnormal nerve transmission involved in central sensitization and hyperalgesia. Other potential therapies for CRPS patients are the bisphosphonates, which inhibit bone resorption. Hyperactive osteoclasts have been hypothesized as a potential trigger of chronic pain in CRPS patients. Calcitonin is believed to possess analgesic properties and to lower serum calcium levels through direct inhibition of osteoclast activity, which may be helpful in relieving pain for CRPS patients. Lastly, inflammation plays a critical role in the development of CRPS through tissue ischemia, hypoperfusion, and microcirculation dysregulation. It is thus understandable that tadalafil, mannitol, and other free radical scavengers (DMSO and N-acetylcysteine) may offer relief in patients with CRPS.
Keywords
Budapest Criteria, Complex Regional Pain Syndrome, sympathetically mediated pain
The treatment options for Chronic Regional Pain Syndrome (CRPS) have dramatically evolved since Dr. Weir Mitchell first described the disease in 1986. He noted a subset of patients who experienced persistent pain despite healing of their gunshot wounds. Over the past 30 years, the disease has been referred to by several different names: causalgia, Sudeck atrophy, reflex sympathetic dystrophy, and now CRPS. Although hundreds of studies and case reports have been published about CRPS, their utility is limited given the numerous classification systems utilized to diagnose the syndrome. Ultimately, researchers applied the International Association of Pain diagnostic criteria. Recently, the Budapest Criteria was proposed to assist practitioners in diagnosing this challenging disease. While the International Association for the Study of Pain (IASP) criteria offered good sensitivity (1.00), it lacked specificity (0.4). The Budapest Criteria showed high sensitivity (0.99) and improved specificity (0.68). This allowed providers a more accurate tool to diagnose patients properly 88% of the time.
CRPS patients are routinely prescribed a combination of interventional procedures, physical therapy (PT), antidepressants, antiinflammatory medications, antiepileptics, and opiates. However, little has been available to patients when these modalities fail to control their pain. In terms of pharmacologic management, there has been increased interest in the use of ketamine, bisphosphonates, calcitonin, magnesium, and free radical scavengers for CRPS. Several studies on these various agents have produced promising results. However, given the lack of consistent diagnostic criteria and treatment algorithms, interpretation of these results is challenging.
A review of the pathophysiology of chronic pain can clarify the roles each agent plays in the treatment of CRPS. Our current understanding of the disease stems from the notion that central sensitization leads to neuropathic pain. Localized trauma causes a release of reactive oxygen particles, inflammatory mediators, and neuropeptides, which create a cascade of events leading to central sensitization or wind up. Local structures, such as C and A-delta fibers, become sensitized and continuously release glutamate, which activate the alpha-amino-3-hydroxy-5-methyl-4-isoxazolepropionic acid receptor (AMPA) via calcium release. As calcium influx occurs, the cell is depolarized and releases the voltage-dependent magnesium block on the N-methyl- d -aspartate (NMDA) receptors. Once magnesium is displaced from the NMDA receptor, signal propagation is activated. The NMDA receptor plays a key role in the induction and propagation of peripheral and central sensitization. This results in an amplified response even to benign peripheral stimuli, known as allodynia or hyperalgesia. It is clear why NMDA antagonists, such as ketamine, may have critical treatment potential. Additionally, calcium plays an integral role in this cascade and its levels are modified through calcitonin and bisphosphonate applications.
Intravenous Ketamine
Ketamine has analgesic properties within the central and peripheral nervous system via interactions with several receptor subtypes including NMDA, opioid, and AMPA. Additionally, ketamine plays a role in the inhibition of serotonin and dopamine reuptake. Whereas some studies have shown complete relief of pain with anesthetic doses of ketamine, the utility of this treatment is limited by adverse effects associated with high doses of ketamine and the financial burden of inpatient hospitalization required for the treatment. Subanesthetic doses of ketamine have offered short-term pain relief for a subset of patients.
Pharmacology
Ketamine is a dissociative anesthetic that offers analgesia and amnesia. It is a phenylepiperidine derivative that is structurally similar to phencyclidine (PCP) with a 2(2-chlorophenyl)-2-(methylamino)-cyclohexane ring. Ketamine has a chiral center that creates two stereoisomers, S(+) and R(−) ketamine. Currently, there are two commercially available forms of ketamine: the S(+) ketamine (S-ketamine or ketanest-S) or the racemic mixture (Ketalar). The S(+) enantiomer is preferable as it has twice the analgesic potency, better cardiovascular profile, and may confer neuroprotective properties.
Intravenous (IV) ketamine has a redistribution half-life of 7–15 minutes, clearance 15 mg.kg −1 .min −1 , and elimination half-life 2–3 hours. Ketamine readily crosses the blood-brain barrier, which allows for rapid onset of acute analgesic effect. It is metabolized in the liver by CYP3A4, CYP2B6, and CYP2C9 to its metabolite norketamine via N-demethylation. Norketamine is produced within minutes of IV ketamine injection and may outlive the parent compound. Ultimately, norketamine undergoes glucuronidation in the liver and is eliminated by the kidneys and bile. Upon discontinuation of ketamine infusion, the concentration of ketamine rapidly drops. However, when utilized for chronic pain, the analgesic effects outlast serum concentrations, likely due to ketamine’s neuromodulatory effects on the NMDA receptor (NMDAR).
Ketamine plays a role in both acute and chronic pain but through different mechanisms. Sigtermans et al. performed an observational study investigating the analgesic effects of ketamine in acute experimental pain versus chronic spontaneous pain in CRPS patients. Ten patients with CRPS underwent seven IV low-dose S(+) ketamine infusions in 5-minute infusion periods. Each 5-minute infusion was followed by a 20-minute break before another infusion (of an increased dose) was initiated. Spontaneous pain ratings and visual analogue scale (VAS) to experimental heat stimulation was recorded during the infusion and 3 hours later. Additionally, serum ketamine levels were monitored throughout the infusions. The authors concluded that ketamine produced profound analgesia (VAS decreased from 6.2 ± 0.2 to 0.4 ± 0.3) by the end of the infusion. However, the analgesic effects of ketamine, with experimental thermal pain, ended when the infusion stopped. Pain relief persisted beyond the termination of infusion (175 minutes after infusion) and when ketamine serum levels were very low (<100 ng/mL). Thus, in the acute setting, ketamine’s effect on experimental pain is dictated by its pharmacokinetics while its effects on CRPS pain persisted despite lower serum concentrations. The authors concluded that, even with a short-lived infusion, ketamine likely plays a neuromodulatory role in CRPS, possibly via desensitization of the NMDA receptor within the spinal cord or via restoration of inhibitory sensory control within the brain.
Mechanism of Action
The pathophysiology of CRPS may involve a combination of the following mechanisms: NMDAR phosphorylation and upregulation, loss of descending inhibition, activation of the immune cells within the spinal cord which release proinflammatory cytokines, and plastic changes in the spinal cord. The NMDAR is an excitatory glutamate receptor located at the dorsal horn of the spinal cord and in supraspinal structures. Central sensitization occurs when prolonged nociceptive input causes activation and upregulation of the NMDAR, resulting in amplified signaling of the pain pathway to the brain. Over time, this pathway is continuously activated, regardless of the stimulus, and can contribute to the perseverance of chronic pain states. As a noncompetitive NMDA antagonist, ketamine blocks the NMDAR and decreases neuronal hyperexcitability. Ketamine has traditionally been thought to antagonize the NMDAR but also interacts with opioid receptors, muscarinic, and monoaminergic receptors.
NMDA receptors are highly expressed in the temporal cortex, hippocampus, basal ganglia, cerebellum, and brainstem. Ketamine may also affect the descending inhibitory pathways involved in chronic pain states. In an elegant study, resting-state functional magnetic resonance imaging (fMRI) was performed before, during, and after subanesthetic ketamine infusions. The investigators showed activation of the anterior cingulate cortex, orbital frontal cortex, insula, and brainstem after the infusion; these areas are involved in the descending inhibitory pain pathway.
Use in Chronic Pain
Pain relief has been reported with anesthetic doses of ketamine. Kiefer et al. conducted an open-labeled phase II study in 20 patients treated with anesthetic doses of ketamine requiring intubation. They noted complete pain relief in all patients at 1 month and in 16/20 patients at 6 months. Additionally, they showed improvement in quality of life, associated movement disorder, and ability to work in the majority of patients. Upon emergence from ketamine anesthesia, the majority of patients reported psychotropic side effects of anxiety, dysphoria, nightmares, and sleep difficulties. Seven patients developed respiratory infections associated with mechanical ventilation. Sixteen patients experienced elevations in their liver function tests, noted on day 5 of the treatment. Unfortunately, similar salutary effects have not been replicated with subanesthetic doses of ketamine. A recent meta-analysis by Collins et al. was not able to show significant pain relief with ketamine infusions in CRPS (−0.65 [CI 95% −1.47, 0.16], P = .11). They noted that while the data are encouraging, further studies are necessary.
The majority of the studies on the effects of ketamine included patients diagnosed according to the IASP criteria who were allowed to continue their home medications throughout the trials. Most studies excluded patients with serious medical issues, drug or alcohol abuse, history of psychosis, increased intracranial pressure, major opioid use, pain scores less than 5 on a 0–10 scale, or pregnancy/lactation. Two randomized controlled, double-blinded, placebo-controlled trials further investigated the benefits of subanesthetic doses of ketamine in the treatment of CRPS. Both showed significant improvement in VAS scores over the treatment duration and in 3-month follow-up despite varying infusion rates. However, neither showed any improvement in functional capacity.
Sigtermans et al. evaluated 60 patients with severe, long-standing CRPS (up to 9 years’ duration of symptoms) where 30 inpatients were treated with ketamine infusions for 4.2 days (1.2 μg.kg −1 .min −1 or 5 mg/hour with up-titration to a maximum of 7.2 μg.kg −1 .min −1 or 30 mg/hour). VAS scores were recorded prior to treatment and then weekly for 11 weeks. VAS scores were dramatically improved within the first week (7.2–2.6) and continued until the 11th week. At 12 weeks, ketamine’s treatment effects subsided. However, while patients reported decreased pain scores, their functional capacity did not improve. Interestingly, this study did not find an association with the duration of symptoms and response to ketamine infusion. The ketamine group complained of more nausea/vomiting, psychotomimetic effects, but there were no differences with respect to headache, blood pressure, and liver function when compared to controls.
A similar study was conducted by Schwartzman et al. in an outpatient clinic setting where 19 patients with severe, long-standing, intractable CRPS received ketamine infusions at a rate of 100 mg/4 hours, which was increased halfway through the study to 200 mg/4 hours for 10 days (5 days on, 2 days off, 5 days on). Patients were pretreated with midazolam 2 mg, clonidine 0.1 mg PO, and an additional midazolam 2 mg posttreatment. Follow-up occurred at 2 weeks, and then monthly for 3 months. This study showed statistically significant improvement in pain scores that lasted until the 12-week posttreatment period, with none of the parameters returning to their pretreatment levels. Similarly to the Sigtermans’ study, this study was not able to show a statistically significant improvement in activity levels or quality of life. Side effects of ketamine included nausea, headache, tiredness, or dysphoria. None of the patients reported hallucination, delusions, or out-of-body experiences.
Although neither randomized control trial (RCT) showed a statistically significant improvement in functional capacity, an open-label prospective study by Goldberg et al. did show improvement in initiation of activity. Forty patients received outpatient infusions of ketamine 40–80 mg/4 hours for 10 days. Similar to Schwartzman’s study, patients were pretreated with midazolam 2–4 mg and clonidine 0.1 mg PO. Goldberg et al. reported a significant reduction in pain scores over the 10-day period and showed a statistically significant improvement in movement initiation by the tenth day of infusion. The authors suggested that longer treatment periods may produce a more pronounced effect. Additionally, they reported 10% of patients had return of “worst pain” by 2 weeks, 62% had at least 70% reduction in worst pain at 6 weeks and were back to baseline pain 9 weeks after treatment. Twenty percent of the patients reported greater than 70% reduction in pain for 11–12 weeks; 8% of the patients were pain-free at 15 months.
Although Sigtermans et al. and Schwartzman et al. evaluated the efficacy of ketamine infusions in patients with CRPS, Gustin et al. examined the role of combination therapy using an NMDA antagonist and morphine in alleviating pain in CRPS patients. The rationale for their study was that NMDA antagonism may reduce the body’s adaptation to morphine. When morphine binds to the μ-receptor, it can release mediators that bind to the NMDA receptor and enhance pain sensitivity. NMDA antagonism may blunt this response. CRPS patients may exhibit central neuroplastic changes involving an alteration of the representation zones in the somatosensory and motor cortexes. Gustin et al. used fMRI to detect cerebral activity changes while treating patients with combination therapy. This double-blinded, randomized controlled trial included 20 patients randomized to either a group treated with an NMDA antagonist and morphine or a group treated with placebo and morphine. Patients were diagnosed using IASP criteria, had symptoms for greater than 6 months (average 16 months), and had a baseline VAS pain score of at least 3. They excluded patients who were left-handed, had contraindications to fMRI, and who had no demonstrable motor deficits on neurological exam.
All patients underwent a 2-day washout period for existing CRPS medications. Morphine was administered 3 times per day for 56 days (titrated from 10 mg for 5 days up to 30 mg for the remaining 51 days). Memantine was started on day 8 at 5 mg per day and titrated up to 40 mg by the end of the study. They used fMRI during movement of both upper extremities, before and after treatment of 56 days. Only the combination group of memantine/morphine showed a statistically significant reduction in pain at rest and during movement. After 49 days of treatment, MRI findings showed reduced activation of the contralateral primary somatosensory and anterior cingulate when the affected limb was moved but not at rest. These reduced activation areas also correlated to pain relief during the treatment period. Overall, they showed that combination therapy significantly affected the cerebral processing of nociceptive information in CRPS patients. Additionally, patients experienced improvement in mood and disability. Side effects of fatigue, drowsiness, nausea, akathisia, vertigo, head pain, and anxiety were mildly reported.
The results of studies on IV ketamine are in Table 54.1 .
Study | Type of Study; Medications Compared; Duration of Follow-Up | Outcome Measures | Results | Comments |
---|---|---|---|---|
Ketamine | ||||
Sigtermans et al. ; 60 patients with CRPS | DB, PC, parallel group. Ketamine 1.2–7.2 μg.kg −1 .min −1 × 4.2 days. 30 pts per group. 12-week follow-up. | VAS, functional capacity. | VAS improved until 11th week. Treatment effect subsided by 12 weeks. No change in functional capacity. | No association with duration of symptoms and response to ketamine. |
Schwartzman et al. ; 19 patients with severe, long-standing CRPS | DB, PC. 9 patients received ketamine IV 100 mg/4 h increased to 200 mg/4 h × 10 days vs. 10 patients with placebo (NS IV). 3-month follow-up. | VAS, functional capacity. | Improvement in VAS past 3 months. No change in functional capacity. | Patients pretreated with midazolam 2 mg before and after treatment, Clonidine 0.1 mg PO. No psychotomimetic side effects noted. |
Gustin et al. ; 20 patients with CRPS | R, DB, PC. 10 patients with memantine 5 mg–40 mg QD + morphine 10–30 mg QD × 8 days. 10 patients with morphine alone. 56-day follow-up. | VAS, fMRI during affected/unaffected hand (before and after treatment) at 49 days for both groups. | fMRI showed reduced activation of contralateral primary somatosensory and anterior cingulate cortexes with affected limb movement. This correlated with improved VAS. Patients had improvement in mood and disability. | NMDA antagonism may reduce the body’s adaption to morphine. |
Bisphosphonates | ||||
Maillefert et al. ; 11 patients with CRPS UE/LE | OL, P. Pamidronate 30 mg in NS 500 mL over 4 h QD × 3 days. Follow-up at 1 and 3 months. | VAS pain scores and physician global assessment based on clinical evaluation (hyperhidrosis, vasomotor changes, joint stiffness), blood cell count, and serum calcium measure | VAS pain scores significantly decreased at 3 months. Global assessment: four patients—no improvement, one patient—moderate improvement, six patients—significant or excellent improvement at 3 months. | Diagnosed by Doury’s criteria, duration of symptoms >6 months, refractory to calcitonin and PT. |
Robinson et al. ; 27 patients UE/LE | PC, R, DB. Pamidronate 60 mg single dose vs. NS. 14 patients given pamidronate vs. 13 patients on placebo. | VAS pain scores, global assessment of disease severity scores, SF-36 at 1 and 3 months. | Overall improvement in VAS global assessment of disease severity score and SF-36 at 3 months. | There was great variability of responses among individuals. Duration of symptoms 3 months–6 years. Used IASP criteria. |
Adami et al. ; 20 patients with CRPS of foot and hand. | R, DB, PC. 10 patients each received alendronate 7.5 mg in 250 NS IV vs. placebo with NS × 3 days. 2 weeks later all patients underwent open label alendronate infusion. Follow-up 12 months. | Pain, tenderness, improvement in motion. | Diminished pain, tenderness, swelling and improvement in motion noted in alendronate group at 2–4 weeks. Similar results during open label phase of placebo patients treated with alendronate. Statistically significant rise in bone mineral density at 6 weeks. At 12 months 9 patients in remission, 7 relapsed. | 10 patients had failed nasal calcitonin therapy. Duration of symptoms 5–34 weeks. |
Manicourt et al. ; 40 patients with LE CRPS | R, DB, PC. 19 patients received alendronate vs. 19 who received placebo. Alendronate 40 mg tablet × 8 weeks. Optional open label phase. | VAS pain score, joint mobility, LE edema, LE tolerance to pressure, levels of spontaneous pain. | Significant decrease in VAS pain score at 4, 8, 12 weeks. Improved joint mobility. The group that received two courses of alendronate treatments showed further improvement in symptoms without plateau effect. | Mean duration of symptoms was 7 months. Excluded patients with previous bisphosphonate therapy, sympathetic blocks, calcitonin therapy. Allowed PT. |
Varenna et al. ; 82 patients with CRPS of hand or foot | PC, R, DB: Neridronate 100 mg QID × 10 days vs. NS. 41 patients per group. Open extension phase where placebo group was treated. 20-month follow up. | VAS pain scores, clinical assessment (edema, pain at motion, allodynia, hyperalgesia) SF-36, McGill Pain Questionnaire | Significant decrease in VAS pain scores and improved clinical signs/functional status. | Most patients were in the early phase of the disease. |
Varenna et al. ; 32 patients with CRPS | R, DB, PC. 15 patients received clodronate 300 mg QD × 10 days vs. placebo (15 patients). After 40-day washout, placebo group received clodronate treatment. Follow-up 180 days. | VAS, clinical global assessment, efficacy verbal score. | Significantly better relief of pain and global assessment. | |
Breuer et al. ; 9 patients of hand and foot CRPS | Observational; 9 patients ibandroante 6 mg IV QD × 3 days. Follow-up 4 weeks. | VAS, neuropathic pain qualities. | Improvement in average and worst pain scores after 4 weeks. 30% of patients with improvement activity levels. | CRPS for at least 1 month. Used to justify a randomized trial. Hand CRPS more common than foot CRPS. |
Magnesium | ||||
Collins et al. ; 8 patients CRPS of UE/LE | R, PC. 6 patients received MG++ 70 mg/kg in 50 mL over 4 h × 5 days vs. 2 patients who received NS. | Pain assessment (11-point box scale and McGill Pain Questionnaire at baseline, 1, 3, 6, 12 weeks). | Pain significantly reduced at all measured time points and McGill sensory subscale was significantly improved at 1 week. At 12 weeks, there was a statistically significant improvement in impairment level and quality of life. | All patients continued PT. No change in skin sensitivity and functional limitations. Diagnosis: IASP criteria. |
Fischer et al. ; 56 CRPS UE/LE | R, PC. 56 patients, 29 patients received MG 70 mg/kg over 4 h × 5 consecutive days. 27 patients received NaCl. | Pain assessment (11-point box scale and McGill Pain Questionnaire at baseline, 1, 3, 6, and 12 weeks). | Statistically significant improvement on level of impairment at 1 week, improvement in McGill pain scores at 6 weeks, improvement in perceived job participation at 12 weeks. | Mean duration of symptoms 16 months. |
Gabapentin and Pregabalin | ||||
Serpell ; 307 patients with neuropathic pain symptoms (28% with CRPS) | R, DB, PC. 153 patients received gabapentin 2700 mg × 8 weeks vs. placebo (154 patients). | Neuropathic pain symptoms, quality of life via SF-36. | Statistically significant reduction in neuropathic pain. | Participants had a wide range of neuropathic symptoms (28% with CRPS). Used IASP criteria. |
Van de Vusse et al. ; 58 patients with CRPS of UE/LE | R, DB, Crossover study. 29 patients treated with gabapentin 1800 mg QD × 3 weeks vs. placebo. 2-week washout with crossover to other treatment group. 8-week follow-up. | VAS, allodynia, edema, discoloration, range of motion, quality of life. | No greater pain relief with Gabapentin over placebo. Sensory deficits significantly reversed in Gabapentin users. No change in allodynia, edema, discoloration, range of motion, quality of life. | Used IASP criteria. Interestingly, first half of the study showed a benefit with Gabapentin. |
Tadalafil | ||||
Groeneweg et al. ; 24 patients with cold LE CRPS | R, DB, PC. 12 patients received tadalafil 10 mg QD × 4 weeks then 20 mg QD × 8 weeks, vs. 12 patients who received placebo. | VAS pain score, temperature change, range of motion, standing, walking. | 15% reduction in VAS vs. placebo, small nonstatistically significant improvement in muscle strength over time, walking ability, and reduced need for crutches. No change in temperature. | Diagnostic criteria not defined. Minimal change in blood pressure. Allowed to do PT. |
Free Radical Scavengers | ||||
Zuurmond et al. ; 32 patients with acute CRPS (secondary to trauma) | P, R, DB, PC. 16 patients treated with 50% DMSO cream (applied five times daily) vs. 17 treated with placebo cream. 2-month follow up. | CRPS symptoms. | Significantly better CRPS symptom improvement than placebo | Diagnosed via Veldman and Dutch criteria. |
Perez et al. ; 41 patients with CRPS-LE | P, R, DB, PC. 22 patients treated with 10% mannitol in 1 L NS in affected limb over 4 h × 5 days vs. 20 patients treated with placebo NS infusion. 9-week follow-up. | Pain, function level, quality of life at 2, 6 and 9 weeks. | No difference in pain function level, or quality of life. | Diagnosed according to Bruehl criteria. Mean duration of symptoms 6.5 months. PT performed in all subjects. Concluded mannitol maybe more helpful during acute phase when more inflammatory mediators are present. |
Perez et al. ; 146 patients with CRPS in UE/LE | R, DB. DMSO 50% (5 times per day) vs. NAC 600 mg TID (both combined with placebo) × 17 weeks. 24-month follow-up. | Impairment level sum score, UE/LE skills/function. | NAC and DMSO were equally beneficial but subgroup analysis showed warm extremity CRPS responded better to DMSO while cold extremity CRPS responded better to NAC tablets. | Allowed rescue with Paracetamol 500 mg, Naproxen 250–500 mg and/or Tramadol. Diagnosed according to Veldman and Netherlands WHO collaboration Criteria. CRPS for >1 year. Allowed to do PT. |
Tan et al. ; 68 patients with CRPS UE/LE | Retrospective, consecutive group of 68 CRPS patients treated with 10% mannitol in 1 L NS per 24 h × 7–10 days. | CRPS symptoms (not specified). Overall improvement defined as <3 of the following symptoms: pain, swelling, edema, temperature, loss of range of motion, and increase in exercise. | 24% improvement after 1 week, 30% after 1 month. Warm CRPS may have responded better than cold CRPS. | Diagnosed via Veldman criteria. Median duration of symptoms 129 days with a range of 3–1046 days. |
Side Effects of Ketamine
Ketamine exposure has several associated risks that can affect the central nervous, cardiovascular, and hepatic systems. Much of our understanding of ketamine’s side effect profile was derived from recreational and chronic ketamine abusers. Given the known risk of central nervous system (CNS) related side effects, it may be prudent to withhold ketamine from patients with schizophrenia, manic depressive disorder, and a history of substance abuse.
Central Nervous System Effects
Ketamine is known to have psychotropic or psychedelic effects, which usually occur in a dose-dependent manner. Niesters et al. examined the effects of subanesthetic doses of ketamine on intrinsic functional brain connectivity in a placebo-controlled fMRI trial. They exposed 12 healthy males to a 2-hour infusion of S(+) ketamine (20 mg/70 kg for the first hour, then 40 mg/70 kg for the second hour). They found NMDAR expression to be high in the temporal cortex, hippocampus, basal ganglia, cerebellum, and brainstem, with the highest concentration noted in the cerebellum. The cerebellum is thought to play a critical role in emotional processing (mainly anxiety) and thought coordination. Niesters’ study showed alterations in connectivity of the cerebellum, which may be related to the psychedelic side effects during ketamine infusions. fMRI also showed large connectivity changes in the visual cortex and the optic radiation, which may explain the visual hallucinations, blurry vision, and double vision observed with ketamine infusion. These CNS effects resolved within 30 minutes of infusion termination. Blagrove et al. reported that unpleasant dreams could persist for up to 3 days after terminating infusion. Midazolam and clonidine have been critical in tempering these effects but do not completely prevent the occurrence of these unpleasant side effects.
Ketamine administration also offers amnestic properties, which are temporary in ketamine-naïve patients. However, limited data are available on memory impairment with long-term use of low-dose ketamine utilization. Koffler et al. studied the effects of anesthetic doses of ketamine on neurocognitive function following a 4.5-day infusion of ketamine (250–300 μg/dL level), which resulted in a medically induced coma. Nine patients with severe refractory CRPS underwent neuropsychological testing before the treatment and 6 weeks following the infusion. They reported no adverse cognitive effects of extended treatment with anesthetic doses of ketamine.
Cardiovascular Effects
Ketamine activates the sympathetic nervous system causing systemic release of catecholamines, inhibition of parasympathetic output, and inhibition of norepinephrine reuptake at peripheral nerves. However, it has direct myocardial depressant properties in high doses. Patients undergoing low-dose ketamine infusions may experience tachycardia, increases in pulmonary arterial pressure, systemic blood pressure, and increases in myocardial oxygen consumption. Thus, all patients undergoing ketamine infusion therapy require continuous monitoring of their heart rate, blood pressure, and oxygen consumption. Some studies have utilized clonidine 0.2 mg to blunt the sympathetic effects of ketamine.
Hepatic Effects
Ketamine-induced liver dysfunction has been reported with prolonged or repeat infusions within a short time frame. Noppers et al. reported six patients with CRPS who were exposed to two 100-hour infusions of S(+) ketamine (10–20 mg/hour) separated by 16 days. Three of the six patients exhibited elevated liver enzymes (up to three times normal values). These levels normalized over the subsequent 2 months. The exact mechanism of injury is unclear but may involve impaired hepatic oxygen delivery, formation of free radicals, or an allergic hepatitis.

Full access? Get Clinical Tree
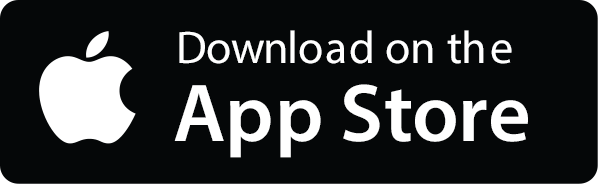
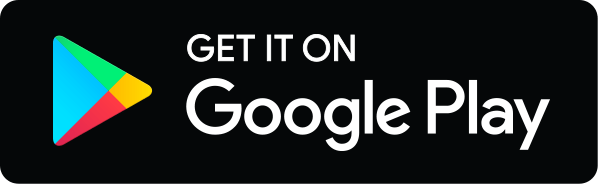