The kinetics of inhaled anesthetics is fundamental to the clinical practice of anesthesia.1 This subject is often called uptake and distribution of anesthetics.2 It explains the time course of anesthetic movement from the delivery system to the site of action, the patient’s central nervous system. Although the site of action of inhaled anesthetic agents includes the brain and spinal cord, “brain” will be used alone in the remainder of this chapter. See Chapter 1 for further discussion of anesthesia site of action.
Inhaled agents are either gases or vapors, depending on their physical state at room temperature and pressure. Nitrous oxide, cyclopropane, and xenon are gases. For these agents, the anesthetic source is a flow controller with flow meter. Halothane, isoflurane, sevoflurane, and desflurane, as well as the historical agents diethyl ether, methoxyflurane, fluroxene, and enflurane are vapors. In this chapter, the agent source for all of these will be called a vaporizer. The physical properties of most of these agents are listed in Table 2–1.
Ether | Halothane | Enflurane | Isoflurane | Sevoflurane | Desflurane | N2O | Xenon | |
---|---|---|---|---|---|---|---|---|
MAC (%) | 1.9 | 0.8 | 1.7 | 1.1 | 2.1 | 6.0 | 110 | 70 |
Blood/gas solubility | 12.1 | 2.47 | 1.90 | 1.30 | 0.65 | 0.42 | 0.47 | 0.13 |
VRG/blood solubility | 1.10 | 1.94 | 1.47 | 1.62 | 1.69 | 1.29 | 0.89 | 1.30 |
Mus/blood solubility | 0.90 | 4.01 | 2.42 | 3.46 | 3.69 | 2.31 | 1.15 | 2.00 |
Fat/blood solubility | 5.00 | 60.7 | 33.2 | 53.8 | 52.3 | 31.0 | 2.30 | 10.0 |
Alveolar plateau height | 0.06 | 0.24 | 0.30 | 0.38 | 0.55 | 0.66 | 0.63 | 0.86 |
VRG (brain) Tau (minutes) | 1.7 | 3.1 | 2.3 | 2.6 | 2.7 | 2.0 | 1.4 | 2.1 |
Muscle Tau (hours) | 0.6 | 2.4 | 1.5 | 2.1 | 2.3 | 1.4 | 0.7 | 1.2 |
Fat Tau (hours) | 4 | 49 | 27 | 43 | 42 | 25 | 2 | 8 |
The measure of anesthetic level in a compartment or location is the partial pressure. Partial pressure is also called tension. Tension is a generic term that applies to variables that equalize in connected locations. Examples are hydrostatic tension (water height) and electrical tension (voltage). The interchangeable terms “high-tension wires” and “high-voltage wires” are familiar examples.
Anesthetic partial pressure or tension could be expressed in common pressure units such as mm Hg, Pa, or kPa. However, the most commonly used unit for anesthetic partial pressure is % atm (percent of one sea level atmosphere). One percent partial pressure represents 1% × 760 mm Hg = 7.6 mm Hg. The anesthetic tension is then said to be 1%. Commercial vaporizers state their delivered tension in percent and thus are consistent with this description.
Equilibrium is achieved when the tensions in compartments are equal. The locations or compartments of interest for inhaled agents are breathing circuit (inspired gas), lungs (alveolar gas), arterial blood, and the idealized body compartments: vessel-rich group (spinal cord, brain, heart, kidneys [VRG]), muscle, fat, and mixed venous blood. Figure 2–1 shows the Gas Man3,4 model that shows the compartments and their partial pressures. The model has been validated for induction and emergence of anesthesia5 and has been used to elucidate fine points of inhalation kinetics.6
Figure 2–1
Gas Man apparatus. The illustration shows a schematic representation of the model for inhalation kinetics. The upper part shows compartments in which anesthetic partial pressure will rise from left to right. The lower part shows the flows that link the compartments. ALV, alveolar tension; ART, arterial tension; CKT, circuit; CO, cardiac output; DEL, tension delivered; FAT, fat; FGF, fresh gas flow; MUS, muscle; VA, alveolar ventilation; VEN, venous; VRG, spinal cord, brain heart, kidneys.

Although equilibrium is achieved when the anesthetic tensions in compartments are equal, anesthetic concentrations in connected compartments differ at this equilibrium according to agent solubility (λ) in each location. For blood and gas in equilibrium, the ratio of concentration in blood to concentration in gas is the blood-to-gas (blood/gas) solubility ratio or blood/gas solubility, expressed as λb/g. The following example explains this.
A 10-mL syringe is filled with 5 mL blood and 5 mL air. A small amount of liquid or vapor anesthetic is added to the syringe, which is then capped and shaken. In the syringe, the tension of anesthetic in the blood and gas compartments equalize while the concentrations equilibrate. At equilibrium, the ratio of concentration in the blood to concentration in the gas is the blood-to-gas solubility ratio or blood/gas solubility. The ratio of drug quantity in these 2 equally sized compartments also equals the blood/gas solubility, λb/g. When the term solubility is used by itself, it usually refers to blood/gas solubility or blood/gas solubility ratio.
As blood passes through the capillaries of the lungs, anesthetic equilibrium is achieved across the alveolar-capillary membrane. Thus, arterial tension equals alveolar tension. The concentration of anesthetic in blood is equal to the product of the partial pressure and the solubility.
Arterial blood perfuses each tissue and tissue tension rises toward arterial tension. During this period, anesthetic tension in venous blood leaving each tissue equals the partial pressure in that tissue, itself. Eventually, anesthetic tension in the tissue equals that in arterial blood. At this time, venous tension equals arterial tension and there is no longer anesthetic uptake into that tissue. The tissue is in equilibrium with blood. All tissues reach equilibrium, some after a few minutes and some after many hours. When final equilibrium is reached, anesthetic tensions in all gas, liquid, and tissue compartments are equal. This takes many days.
To understand the kinetics of inhaled agents, we analyze the time course of anesthetic tension in the patient’s lungs or alveoli (alveolar tension [Pa]) in response to a step change in inspired tension (Pi). This was first described by Kety in 1950. This is called the alveolar step response or the alveolar tension curve. The alveolar tension curve describes the time course of Pa in response to a step change in Pi.
The alveolar tension curve has the same general shape for all inhaled agents because they share the same physiology of drug delivery and removal. The alveolar tension curve components are named in accordance to their shape as described by Kety. They are the initial rise, plateau, knee, and tail. The alveolar tension curve for isoflurane is shown in Figure 2–2.
The first portion of the curve is called the initial rise. See Figure 2–3. This is how the shape would look if there were no removal of anesthetic from the alveoli by blood. This would happen in the following imaginary situations: cardiac output is zero, lungs were not connected to the cardiovascular system, and agent solubility in blood (λb/g) is zero. The initial portion of the alveolar tension curve follows this shape for all agents. The curve shape of the initial rise is an exponential curve that is shown in Figure 2–3 and expressed mathematically as
Figure 2–3
The rise in alveolar tension in response to a step change in inspired tension in the absence of uptake into blood. Small circles represent values at 1, 2, 3, and 4 time constants. See text for details.

Full access? Get Clinical Tree
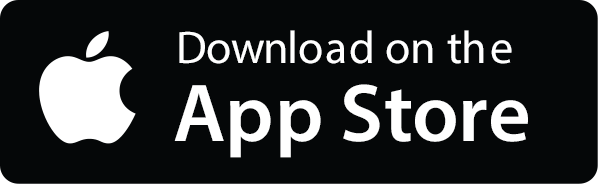
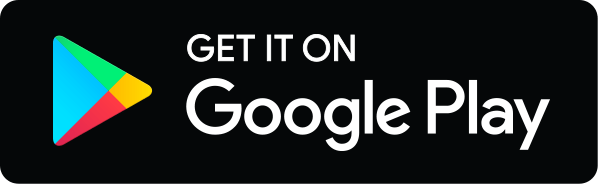
