Pharmacodynamics
Pamela Kirwin
Pharmacodynamics is the relationship between the plasma drug concentration and the pharmacologic effect of the drug on the body, or more simply put, pharmacodynamics is the study of what drugs do to the body. Pharmacodynamics can be divided into three general areas:
The transduction of biologic signals: how drugs act at a cellular level to affect what is happening within the cell. A major component in understanding transduction is understanding how cellular receptors work.
Molecular pharmacology: the molecular properties of drugs and how they interact with organisms at a molecular level.
Clinical pharmacology: the clinical effects drugs have on an organism or organ system.
▪ TRANSDUCTION AND RECEPTORS
Receptors are external components of cells (in the cell membrane) that interact with compounds such as drugs or biochemical signals to start an intracellular cascade of reactions. There are three main components of the receptor theory. These include the quantitative actions of drug binding and the resulting effect, the selectivity of drugs and their ability to activate the cell, and the pharmacologic activity of those drugs at the receptor.
The ability of drugs to bind to receptors is critical to their action. The specific receptor is an important determinant of what intracellular cascade gets triggered. Drugs that have similar biochemical structures often adhere to the same receptor. When a drug interacts or binds to a receptor, there is a quantitative relationship between the dose of the drug and the resulting effect of the intracellular cascade it sets off. In addition to the quantity of drug binding to receptors, drugs may bind with variable strength to a receptor. The biologic activity of a drug that strongly binds may be different than the activity of a drug that weakly binds. Drugs are sometimes developed to bind selectively to certain subsets of receptors in order to either enhance drug effect or avoid drug side effects. A drug that binds to a receptor to initiate a cellular process is called an “agonist.” It is important to be aware that not all drugs bind to receptors to activate a process. Some drugs bind to receptors in order to inhibit a process from happening within the cell. These drugs are called “antagonists.”
A cornerstone to receptor theory emerged from the work of Paul Erhlich. Erhlich studied the activity of curare on parts of the central nervous system and developed the concept that “agents cannot act unless they are bound.” This is the foundation on which the receptor theory was built.
Classical receptor theory is best described through the mathematical relationship shown in Figure 5.1.
In this equation, L is the ligand (the agent binding to the receptor), R is the receptor, Kon is the speed at which the ligand binds to the receptor, Koff is the speed at which the ligand is released from the receptor. The speed at which drugs bind and are released from receptors greatly impacts their effect on the receptor and the cell. This equation can be rearranged to form an equation that describes the relationship between the speed at which binding and release from the receptor takes place. This is called “Kd,” the dissociation constant (Fig. 5.2).
From the dissociation constant, you can extrapolate the activity of the molecule and how it binds to a receptor. A low Kd means that the speed at which the drug binds to the receptor is much greater than the speed at which it is released from the receptor; therefore, not many molecules of the drug are required to occupy the majority of the target receptors. A low Kd indicates the drug tightly binds to the receptor. A higher Kd means that more molecules of a drug are required to occupy the majority of the receptors. Therefore, the drug is weakly bound to the
receptor and falls off quickly. To have an equivalent activity to a drug with a high Kd, a drug with a low Kd would require many more drug molecules to keep attempting to bind to the receptor to make up for the weak binding.
receptor and falls off quickly. To have an equivalent activity to a drug with a high Kd, a drug with a low Kd would require many more drug molecules to keep attempting to bind to the receptor to make up for the weak binding.
A great deal can be learned about a drug by measuring the amount of drug necessary to produce an effect on a cellular process. For example, how much drug is necessary to add to a group of cells in a test tube to get them to produce a certain quantity of hormone? How is receptor binding measured in actual humans? This is often accomplished by measuring an indirect action (secondary endpoint) as a result of receptor binding. A secondary endpoint is used to derive information about the way molecules bind to receptors. For example, to measure how a blood pressure medicine binds to a receptor and then compare it to another blood pressure medicine, which targets the same receptor, you might measure the percent and duration of a change in blood pressure not the actual binding of the molecule to the receptor. Measurement of drug activity at a receptor in actual humans can be very complicated. It may be difficult to find or measure secondary endpoints, the drug may require metabolism or shifting into other compartments of the body in order to finally bind to its receptor, or there may be physiologic delay in the actual effect.
Receptor Agonists and Antagonists
Drugs are agonists of receptors if they attach to a receptor and create an effect. The effect may be excitatory or inhibitory on a process within the cell. In other words, an agonist may cause an effect that is inhibitory and still be considered an “agonist” of that effect. Agonists of receptors fall into two common categories: partial agonists and full agonists. A drug that is highly effective at activating a receptor is called a full agonist. Any drug that activates the same receptor but has a significantly less effect on activating the receptor is considered a partial agonist. Buprenorphine is a partial opioid agonist that only partially activates the euphoria associated with opioids. Some believe partial agonists do not bind strongly to the receptor and this may be why their effect is more weakly produced. In addition, the partial agonist may be incompletely bound to the receptor or is unable to maintain the receptor’s activated shape after it is bound. Even at increasingly higher doses, partial agonists cannot fully activate a receptor.
Antagonists bind to a receptor and prevent the receptor from causing a process to occur. Some antagonists bind irreversibly to the receptor, and in some cases this can be for the life of the cell. Others bind and release from the receptor (see disassociation constants above) and are called competitive antagonists. Competitive antagonists inhibit either endogenous molecules or other drug molecules from binding to the target receptor. Because there is a competition between agonists and antagonists for binding to the receptor, the competitive antagonists can be overwhelmed by giving higher and higher doses of an agonist. Noncompetitive antagonists, those that bind irreversibly to the receptor, cannot be overwhelmed by increasing the amount of agonist. An example of a noncompetitive antagonist is aspirin, which fully antagonizes the blood clotting function of the platelets after it binds to the cyclooxygenase receptor. Once aspirin binds to the platelet cyclooxygenase receptor, it is irreversible. This is why aspirin is usually withheld for 5 to 7 days prior to surgery. The body needs 5 to 7 days to generate new platelets that have not been exposed to aspirin and can help the patient clot during surgery.
The use of competitive antagonists can be found in the administration of anesthesia. One example is nondepolarizing muscle relaxants (e.g., rocuronium, cis-atracurium, and vecuronium). These drugs are frequently used in the operating room to create muscle paralysis. Muscle paralysis may be necessary to intubate a patient or to create relaxed muscles that will make it easier for the surgeon to perform surgery.

Full access? Get Clinical Tree
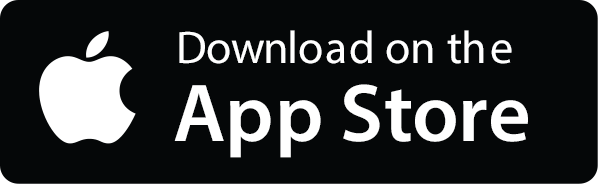
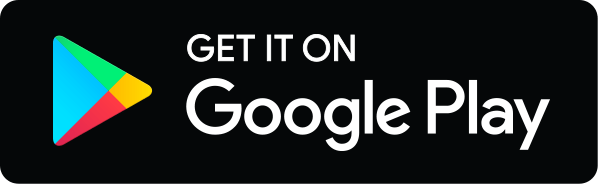