Perioperative pain control remains one of the primary concerns for surgical patients, surgeons, and anesthesiologists. Traditionally, opiates have been the medications used for the treatment of perioperative pain. However, as our knowledge of molecular nociception has expanded, it has become apparent that multiple receptor subtypes are involved in the neurochemical basis for pain (Figure 11–1). As perioperative physicians, anesthesiologists exploit this knowledge by using pharmacologic agents in addition to opiates to control surgical pain.
This multimodal approach to perioperative pain control has gained popularity. Common perioperative pain adjuncts include ketamine, gabapentin, pregabalin, clonidine, and dexmedetomidine, neuraxial blocks, peripheral nerve blocks, systemic nonsteroidal anti-inflammatory drugs, tricyclic antidepressants, and local anesthetics.
In addition to opiate-sparing effects, adjuncts may be useful in 2 clinical scenarios: (1) achieving safe and effective analgesia for the opiate tolerant surgical patient, and (2) reducing patients’ risk of having chronic postsurgical pain syndromes after undergoing procedures that place them at risk for chronic pain.
The recent increased interest in multimodal pain control is in part a consequence of the steady rise in opiate use since the late 1990s. A review examining the trends of opiate use in the United States from 1997 to 2007 revealed some alarming trends.1 While constituting only 4.6% of the world’s total population in 2007, Americans were consuming 80% of the global prescription opioid supply. The average sale of opioids per person in the United States increased 402% from 1997 to 2007. Opiate use, both prescribed and illicit, may be more prevalent in surgical patients than these numbers suggest.
In tolerant patients, opiates used to treat surgical pain are less effective and potentially more dangerous, because patients often require excessive doses to achieve analgesia that are associated with adverse effects in opioid-naive patients. Thus, the benefits of pain control adjuncts are of particular interest to perioperative physicians. The evidence regarding the use of these agents in opiate-tolerant surgical patients will be discussed below.
There is evidence that perioperative pain adjuncts may aid in the prevention of chronic postsurgical pain. Certain surgeries, such as thoracotomies and limb amputations, can produce chronic pain syndromes in as many as 30% to 50% of patients who undergo these procedures.2 The limited data pertaining to the use of pain control adjuncts for the prevention of chronic pain syndromes after surgery will be reviewed for selected agents.
Ketamine was invented in the early 1960s as part of an effort to find a safer alternative to phencyclidine for the induction of anesthesia. It was first given to humans in 1963.3 As an anesthetic agent, it was unique in many ways. In doses of 1 to 2 mg/kg, it produced general anesthesia with minimal respiratory depression and often caused tachycardia and hypertension. Ketamine was commonly used as an induction agent, but its tendency to cause dysphoria and hallucinations in large doses made it unpopular. Ketamine was supplanted first by thiopental and then by propofol as a routine induction agent for humans. In addition to ketamine’s current use as a perioperative pain adjunct, ketamine is also used today for sedation of pediatric and burn patients, as a secondary treatment for bronchospasm, and as an agent for the induction of anesthesia in patients with decompensated hemorrhagic or septic shock.
Ketamine is primarily a N-methyl d-aspartate (NMDA) receptor antagonist. NMDA receptors are ion channels that have excitatory properties. Specifically, it is a noncompetitive NMDA receptor calcium channel pore antagonist and also interacts with phencyclidine (PCP) binding sites that inhibit NMDA receptor function.4 It also interacts with selected opioid receptors (μ, d, and κ), muscarinic receptors, voltage-gated calcium channels, and monoaminergic receptors.4 As a pain adjunct, ketamine reduces both nociceptive and opioid-based hyperalgesia, likely at the spinal cord level.5 It has S and R enantiomers, both of which are pharmacologically active and can produce anesthesia, dysphoria, analgesia, and dissociation.3 The S enantiomer is up to 3 times as analgesic as the R enantiomer. At high doses, ketamine also behaves as a local anesthetic by blocking sodium channels in a comparable fashion to lidocaine or procaine.6
In addition to its acute effects, perioperative ketamine may reduce pain over prolonged periods of time.7 Preliminary work indicates that when administered to chronic pain patients, some feel relief with ketamine for up to 24 hours after receiving ketamine while others do not. The mechanism is not well defined.
In general, ketamine has a rapid onset of effect, reaching peak effect-site concentrations within 5 minutes of a bolus dose. Because ketamine is often prepared as a racemic mixture and has active metabolites, it is difficult to truly characterize its pharmacokinetics and even more so its pharmacodynamics. Pharmacokinetic models exist but are only able to predict plasma concentrations of ketamine and its metabolites. Effect-site concentrations and predictions of drug effect are difficult to estimate given that drug effect can be from either the parent drug, its metabolites, or both.
Intravenous ketamine has a short α and b half-life, approximately 7 minutes and 2 to 4 hours, respectively, when given as a bolus. Consider the simulation of a low-dose intravenous bolus, 0.2 mg/kg, typical of ketamine use as an adjunct. It reaches peak effect-site concentrations (near 0.4 mcg/mL) within 5 minutes and then has a relatively slow decline in concentration (Figure 11–2). With bolus dosing, ketamine rapidly distributes to peripheral tissues (the initial rapid drop in concentration) followed by a redistribution from peripheral tissues back into the plasma and secondarily by hepatic biotransformation.8 As a continuous infusion, ketamine’s kinetic profile is described as an initial rapid rise in effect-site concentrations followed by a long, slow rise to reach near steady-state conditions. A typical continuous infusion–dosing scheme for ketamine used as an adjunct (0.2 mg/kg/h) is presented in Figure 11–3. In this simulation, the effect-site concentrations from a 2- and 8-hour infusion illustrate the slow rise to near steady-state concentrations (ie, not much change in concentration over time). Peak plasma concentrations for the 2- and 8-hour infusion are 0.15 and 0.2 mcg/mL respectively. Also of note with ketamine is its notable back-end kinetics. Following 2- and 8-hour infusions, its context sensitive half-time ranges from 20 to 100 minutes. Thus, for long infusions, patients may have persistent effect-site concentrations well into their recovery period.
Figure 11–2
Simulation of an induction bolus dose of ketamine (1 mg/kg) to a 100-kg, 183-cm male. Concentrations were predicted using previously published pharmacokinetic parameters. Ce, effect-site concentration. (Ihmsen H, Geisslinger G, Schuttler J. Stereoselective pharmacokinetics of ketamine: R(–)-ketamine inhibits the elimination of S(+)-ketamine. Clin Pharmacol Ther. 2001;70(5):431-438; Persson J. The ketamine enigma. Acta Anaesthesiol Scand. 2008;52(4):453-455; Persson J, Hasselstrom J, Maurset A, et al. Pharmacokinetics and non-analgesic effects of S- and R-ketamines in healthy volunteers with normal and reduced metabolic capacity. Eur J Clin Pharmacol. 2002;57(12):869-875; and Voss, LJ, Baas CH, Hansson L, Steyn-Ross DA, Steyn-Ross M, Sleigh JW. Investigation into the effect of the general anaesthetics etomidate and ketamine on long-range coupling of population activity in the mouse neocortical slice. Eur J Pharmacol. 2012;689(1-3):111-117). Simulation limitations: ketamine is an enantiomer and has active metabolites. This simulation does not account for differences between R and S isomers, which are known to have different pharmacologic properties (White PF, Schuttler J, Shafer A, et al. Comparative pharmacology of the ketamine isomers. Studies in volunteers. Br J Anaesth. 1985;57(2):197-203) nor does it account for the active ketamine metabolite, norketamine.

Ketamine is metabolized by hepatic microsomal enzymes cytochrome P CYP3A4, and to a lesser extent by CYP2B6 and CYP2C9, into pharmacologically active metabolites, primarily norketamine and to a lesser extent dehydronorketamine. The S enantiomer of ketamine is more rapidly metabolized than the R enantiomer.9 The kidney excretes 90% of ketamine metabolites. Ketamine and its metabolites are 50% to 70% protein bound.10
Ketamine is commercially available under multiple names (Ketalar, Ketaject, Ketaset, Vetalar) and comes prepared as a hydrochloride salt in doses of 10 or 100 mg/mL. As a pain adjunct, ketamine can be administered via intravenous and intramuscular routes.
A wide range of dosing strategies has been studied. Common dosing regimens include a preincision 0.1- to 0.5-mg/kg bolus followed by repeated hourly intraoperative 0.1- to 0.3-mg/kg boluses or a 0.1- to 0.5-mg/kg/h infusion. Postoperative ketamine administered as a continuous infusion or via patient-controlled analgesia has also been studied.11 Interestingly, a Cochrane meta-analysis review of ketamine as a perioperative pain adjunct reported a ceiling to the morphine-sparing effect for ketamine; doses of ketamine greater than 0.5 mg/kg over the first 24 hours did not result in additional opiate sparing.
The US Food and Drug Administration (FDA) has approved ketamine for induction and maintenance of general anesthesia but not as a perioperative pain adjunct. Perioperative subanesthetic doses of intravenous ketamine, administered as boluses or as continuous infusions, represent an off-label application. Epidural ketamine has been studied as a pain adjunct but does not have FDA approval. Spinal administration is not recommended given potential concerns for neurotoxicity.
Low-dose ketamine has little to no hemodynamic effect. The effect of low-dose ketamine on the minimum alveolar concentration (MAC) of volatile anesthetics is unknown. From the author’s personal experience, intravenous ketamine given in doses exceeding 0.5 mg/kg intraoperatively or given as a bolus near the end of a general anesthetic may delay emergence from anesthesia, particularly in the opiate-naive patient.
While the intraoperative effects of low-dose ketamine may be subtle, the postoperative effects of subanesthetic ketamine are more pronounced. A Cochrane meta-analysis showed that perioperative ketamine administration decreased rescue analgesic requirements over the first 24 hours by an average of 30% to 50%.11 Ketamine may also suppress hyperalgesia after high-dose intraoperative opiate infusions.4
Despite these apparent advantages, the Cochran review did not demonstrate a reduction in postoperative acute pain scores with low-dose ketamine.11,12, and 13 This may be attributed to the subjectivity of pain scoring systems used and the heterogeneity of studies included in the meta-analyses. Anecdotal experience suggests that perioperative ketamine may improve a patient’s satisfaction with pain management, even if the reported pain scores (Visual Analog Score [VAS]) remain unchanged.
Although ketamine as a pain adjunct has been extensively studied, only a few studies have explored its effectiveness in managing perioperative pain in opiate-dependent patients. Most notably, Loftus et al found that a preincision of 0.5 mg/kg bolus followed by an intraoperative continuous infusion of 10 mcg/kg/min in opiate-tolerant surgical patients undergoing spine surgery reduced morphine use by 30% over the first 48 hours following surgery. This study also reported that this regimen reduced pain scores by 25% in the postanesthesia care unit.14
Some investigators have explored the use of ketamine to minimize the risk of developing chronic pain after surgery. Whether ketamine directly or indirectly, via its opiate-sparing and hyperalgesia-reducing effects, lowers the risk of developing chronic postsurgical pain is unknown.
For example, in a randomized study of patients undergoing total hip arthroplasty, one group received a 0.5-mg/kg bolus prior to incision followed by a 24-hour ketamine infusion of 2 mcg/kg/min. A second group received a saline bolus and infusion. The ketamine group used fewer opiates over the first 24 hours and reported less pain in the operative hip at both 30 days and 180 days postoperatively.15 Subanesthetic doses of intravenous ketamine used in patients undergoing surgery for rectal adenocarcinoma produced similar reductions in pain scores even at 12 months postoperatively but only in the patients who received intraoperative total intravenous ketamine doses that exceeded 0.5 mg/kg.13 Lastly, the Loftus study of perioperative ketamine for opiate-tolerant patients undergoing complex spine surgery not only demonstrated the acute pain benefits mentioned above but also showed patients in the ketamine arm had reduced pain scores at the 6-week postoperative visit.14
Limited data regarding perioperative ketamine for the prevention of chronic pain in other high-risk surgical patients has not shown benefit. Perioperative ketamine has been specifically studied for the prevention of chronic pain after thoracotomy and limb amputation, and it failed to reduce the incidence of chronic postoperative pain following these procedures.16,17
Ketamine is well known for its psychomimetic adverse effects. For example, large bolus doses of 1 to 2 mg/kg of intravenous ketamine produce dysphoria, emergence delirium, or hallucinations in 30% of patients.3 However, with low-dose ketamine (< 0.5 mg/kg), adverse psychomimetic effects are uncommon, although sedation on emergence and diplopia may occur.11 The incidence of postoperative nausea and vomiting was reduced with ketamine in studies that specifically measured that end point.
No known contraindications exist for low-dose ketamine. However, possible risks of increased sympathetic tone, increased intracranial pressure from cerebral vasodilation, and increased emergence delirium must be weighed against the potential benefits of using low-dose ketamine to improve perioperative pain control.
Gabapentin and pregabalin are lipophilic γ-aminobutyric acid (GABA) analogs and were initially developed as anticonvulsant drugs. Through various case reports in the 1990s and early 2000s, both drugs were found to have analgesic properties. Further studies proved that these drugs were particularly useful for the treatment of neuropathic pain. Consequently, gabapentin received FDA approval in 2002 for the treatment of postherpetic neuralgia, and pregabalin received FDA approval in 2004 for the treatment of pain associated with diabetic peripheral neuropathy as well as for postherpetic neuralgia. In 2007, pregabalin became the first drug specifically approved by the FDA for the treatment of fibromyalgia.18
Although gabapentin and pregabalin are structurally derived from the inhibitory neurotransmitter GABA, they do not directly bind to GABA receptors in the central nervous system. Instead, they bind to the α-2-δ subunit of voltage-dependent calcium channels in the periphery, spinal cord, and brain. The resultant decrease in calcium influx reduces the release of several neurotransmitters, including glutamate, norepinephrine, dopamine, and serotonin.19 It is through this α-2-δ calcium channel mechanism that gabapentin and pregabalin are thought to exert their anticonvulsant, anxiolytic, and analgesic effects.
Gabapentin is maximally absorbed through the gastrointestinal tract 3 hours after ingestion. Its oral bioavailability after a single dose of 300 to 600 mg is approximately 50%. This absorption is limited by a saturable, dose-dependent active transport mechanism, such that the percentage of the drug that is bioavailable decreases with increasing doses. Onset of action following consumption by mouth is within 1 to 3 hours. It is less than 3% protein bound with a volume of distribution of 0.6 L/kg.
Pregabalin is rapidly absorbed through the gastrointestinal tract, resulting in peak blood concentrations within 1 hour of ingestion. Bioavailability exceeds 90% and is independent of dose. Onset of action following consumption by mouth is also within 1 to 3 hours. It is not protein bound and has a volume of distribution of 0.5 L/kg. Pregabalin’s elimination half-life ranges from 4 to 7 hours. It is important to note that pregabalin’s binding affinity for the α-2-δ receptor is 6 times greater than that of gabapentin.19
Gabapentin is not metabolized, and instead is removed almost completely by renal excretion, with an elimination half-life of 5 to 9 hours.20
Pregabalin, like gabapentin, is not metabolized and is eliminated almost exclusively by renal excretion.
Gabapentin exists as its brand name formulation, Neurontin, or as a generic equivalent. It is formulated in oral capsules and tablets, with strengths ranging from 100 to 800 mg per pill. An oral solution of 250 mg/5 mL is also available. As a perioperative pain adjunct, the appropriate dose for gabapentin is largely unknown. Investigational strategies using a single preoperative dose range from 300 to 1200 mg. Multidose studies have most commonly used 600 mg 3 times a day, beginning on the day of surgery and continuing until postoperative day 2 or 3.
Pregabalin can be found only as its brand name formulation, Lyrica. It is available in oral capsules, with strengths ranging from 25 to 300 mg per capsule. The appropriate dose for pregabalin as a perioperative pain adjunct is unknown. Investigational preoperative doses range from 75 to 600 mg, but single preoperative doses of 150 to 300 mg are most common.20
As described above, both gabapentin and pregabalin are eliminated almost exclusively by renal excretion. Consequently, both drugs should be given in decreased doses in patients with impaired creatinine clearance.
Gabapentin and pregabalin have been approved for the treatment of partial seizures and for a variety of chronic pain conditions. The administration of gabapentin or pregabalin as a perioperative adjunct for the treatment of acute pain constitutes an unlabeled use.
The intraoperative effects of preoperative gabapentin and pregabalin administration are largely unknown. These drugs appear to have little to no effect on intraoperative hemodynamics. No data on alterations of MAC with gabapentin or pregabalin exist. Limited data suggest that the addition of preoperative gabapentin at doses greater than 400 mg can partially blunt the sympathetic response to laryngoscopy.20 No data on gabapentin’s or pregabalin’s effect on the timing of emergence from anesthesia exist.
The preoperative administration of gabapentin and pregabalin has a measurable opiate-sparing and analgesic effect postoperatively. Meta-analyses of perioperative gabapentin and pregabalin suggest their use results in a 20% to 60% reduction in opiate requirements over the first 24 hours postoperatively and a significant reduction of pain scores (Visual Analog Score; VAS).21,22 There are also data to suggest that perioperative use of gabapentin and pregabalin reduces movement-evoked pain in the postoperative period, which may lead to accelerated postoperative functional recovery.
At present, there are no studies that specifically examine the effectiveness of using gabapentin or pregabalin to control perioperative pain in opiate-tolerant patients. Nevertheless, the analgesic and opiate-sparing effects of gabapentin and pregabalin make these drugs theoretically appealing for the management of opiate-tolerant surgical patients, especially because of the decreased analgesic benefit and narrowed therapeutic index of opiates in this patient population.
Several studies have shown gabapentin or pregabalin to be effective in reducing the risk of chronic pain after particular types of surgery. This has been demonstrated with gabapentin in patients undergoing hysterectomy, and with pregabalin in patients undergoing total knee arthroplasty, mastectomy, and lumbar discectomy.23,24, and 25 However, a study in which a 30-day perioperative course of gabapentin was administered to patients having surgical limb amputations failed to show a reduction of stump pain and/or phantom limb pain at 3-month and 6-month follow-up appointments.26 No other data that specifically pertains to the use of perioperative gabapentin or pregabalin for the prevention of chronic pain in high-risk surgery patients currently exist.
The long-term use of gabapentin and pregabalin is associated with somnolence and dizziness, with reported incidences as high as 25%.20 These adverse effects are less common when gabapentin and pregabalin are used over a brief duration in the perioperative setting. The majority of the randomized control trials examining perioperative gabapentin or pregabalin show no difference in their side-effect profiles when compared to placebo.20 A few perioperative trials did report more frequent sedation with gabapentin, and a single trial reported more frequent headache and dizziness with pregabalin.20
In their capacity as perioperative pain adjuncts, the contraindications for the use of gabapentin and pregabalin are not well defined. When deciding whether to use these medications, the small increased risk of sedation or other adverse effects must be weighed against the possible benefit of improved pain control for the individual patient.
Clonidine was first synthesized in 1965. It was used initially as a nasal decongestant and subsequently as an antihypertensive.27 Over the past 4 decades, clonidine has been used for a wide variety of clinical applications, including the treatment of opiate withdrawal, prevention of myocardial ischemia, and as a pain adjunct. As an analgesic, clonidine has been administered intrathecally, epidurally, orally, topically, intramuscularly, and intravenously. It is also used as an additive to local anesthetics to enhance the duration and efficacy of peripheral nerve blocks.27
Dexmedetomidine is the pharmacologically active R enantiomer of medetomidine, a drug that has been used for decades in veterinary medicine as a sedative.28 Dexmedetomidine differs from clonidine in its high-binding specificity for the α-2-adrenoreceptor. In 1999, the FDA approved the use of dexmedetomidine infusion for sedation in intubated patients in the intensive care unit.28 Dexmedetomidine received FDA approval in 2008 for use in nonintubated patients as a sedative prior to and/or during surgery or other procedures.

Full access? Get Clinical Tree
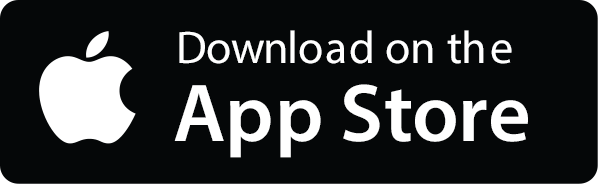
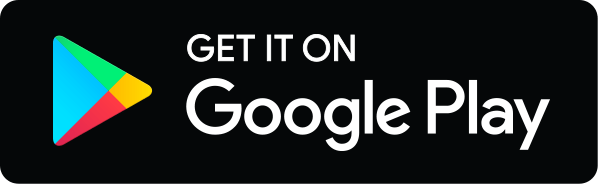