© Springer International Publishing Switzerland 2016
Marinella Astuto and Pablo M Ingelmo (eds.)Perioperative Medicine in Pediatric AnesthesiaAnesthesia, Intensive Care and Pain in Neonates and Children10.1007/978-3-319-21960-8_88. Perioperative Care of the Pediatric Neurosurgical Patient
(1)
Anesthesiology Institute, Cleveland Clinic Abu Dhabi (CCAD), Swing Wing L7-207, Swing Wing L7-207, # 112412, Al Maryah Island, Abu Dhabi, United Arab Emirates (UAE)
The improvement in diagnosis, treatment, and outcome in infants and children treated for neurosurgical procedures has increased in the last years due to the new technologies available for neuromonitoring, standardized protocols for anesthesia maintenance, improved postoperative acute care, and highly specialized anesthesiologist taking care of these patients. The anesthesiologist taking care for neurosurgical patients has to keep in mind that neurological diseases are often long-term diseases and their management has to include since the beginning patients’ families, psychologists, and an integrated social care system as these diseases can be associated with mild to severe disabilities.
The developing brain of a child has specific aspects to be considered such as age-dependent differences in anatomy, metabolism, cerebrovascular physiology, and locations of neurologic lesions. The perioperative management of children undergoing neurosurgical procedures is based on the acknowledgments of the differences on the pediatric neurophysiology, the neurosurgical procedures, and treatment of related complications.
8.1 Neurophysiology of the Pediatric Brain
8.1.1 Cerebral Blood Flow
Cerebral blood flow (CBF) varies with age. CBF of newborns and premature infants is lower than adults (40–42 ml 100 g−1 min−1), while in term infants and older children, values become higher than in adults. From 6 months to 3 years, the CBF is thought to be 90 ml 100 g−1 min−1 and from 3 to 12 years at 100 ml 100 g−1 min−1 [1, 2].
8.1.2 Brain Energy Metabolism
The brain requires large amounts of energy to maintain its cellular integrity and support neurotransmission. The pediatric brain has a higher glucose consumption (6.8 mg of glucose per 100 g−1 min−1) compared to an adult brain (5.5 mg per 100 g 100 g−1 min−1). The brain has no reserve of glucose to face this large requirement. Glucose is stored as glycogen and enters the glial cells (mostly astrocytes) using a facilitated ATP Na + −K+ transport system. This membrane transport system is limited by its kinetic constant, and the amount of glucose transported into the astrocytes actually decreases when the plasma glucose level increases. This could provide some protection to the brain tissue against excessive intracellular hyperglycemia in the normal brain. However, the glucose transport system could be altered in case of brain injury leading to hyperglycemia and secondary brain tissue damage. As a consequence a tight glycemic control is mandatory to prevent and treat the injured brain [3, 4].
8.1.3 Cerebral Oxygen Metabolism
With the only exception of the glomic cells in the carotid body, the brain is the organ with the highest rate of oxygen consumption. It is 3.5 ml O2 × min−1 × 100 g−1 in adult and 5.5 ml O2 × min−1 × 100 g−1 in children. When this is compared with the overall oxygen consumption rate of the body (0.3 ml O2 × min−1 × 100 g−1), it is more evident why the brain is very sensitive to hypoxia. Most of the oxygen consumption in the brain is used to maintain cellular integrity and electrogenesis and sustain cellular transport mechanism such as reuptake of neurotransmitters. When the supply of oxygen decreases, electrogenesis is impaired and quickly ceases. Cerebral autoregulation and cerebrovascular reactivity are impaired and the neuronal integrity is jeopardized. In the presence of hypoxia, ion pump impairment prevents normal membrane repolarization and leaves the neuron in a constant refractory state. Neuronal integrity is initially maintained, and if oxygen supply is restored, neuronal function resumes rapidly (zona pellucida). With ongoing lack of oxygen, however, neuronal viability and integrity deteriorate with increasing duration of hypoxia (zona penumbra). In this brain tissue area, neuronal damage will become irreversible if hypoxia persists at normothermia.
8.1.4 Arterial Carbon Dioxide/Oxygen Tension (PaCO2/PaO2)
Arterial PaCO2 has a major vasodilatory effect on cerebral blood vessels, leading to an increase in CBF, which is linear between a PaCO2 of 3.5 and 8 kPa. At birth, the cerebrovascular response to changes in PaCO2 is incompletely developed. For this reason, moderate hypocapnia has mild effects on the newborn brain compared to adults and CBF has only moderate changes until severe hypocapnia occurs. Brain ischemia due to hypocapnia is thought to be caused by the effect of pH and CO2 on cerebral vascular tone. The association between hypocapnia and clinical adverse outcome in various studies in adults and children has resulted in the advice to apply therapeutic hypocapnia only after careful consideration of the risks and the potential benefits. Special attention should be given to avoid accidental hypocapnia [5].
Capnography is standard in anesthesia monitoring and to prevent hypo- and hypercapnia. It is of crucial importance to be aware of the limitations of the end-tidal measurement of carbon dioxide (ETCO2) in the neonate and small infants. Although the ETCO2 value is in general lower than the corresponding PaCO2 value, in some patients, the ETCO2 overestimates PaCO2. The frequent evaluation of the capillary or arterial blood CO2 trend is mandatory for the prevention of clinical hypocapnia in long procedures, major surgery, and compromised neonates.
The cerebral vasculature of adults is less sensitive to changes in PaO2 and CBF does not increase until PaO2 decreases below 50 mmHg, when it increases exponentially. In neonates, CBF is more sensitive to hypoxia and increases even in response to smaller decreases in PaO2 [6].
8.1.5 Blood Pressure and Cerebral Autoregulation
The lower limits of cerebral autoregulation in neonates are not precisely known, and there is likely a wide range of variability in infants. Several studies have shown that the lower limit of cerebral autoregulation for some infants is indeed fairly close to the definition of hypotension using the infant’s age in gestational weeks although there is also evidence that some premature infants are able to demonstrate cerebral autoregulation at a MAP level considerably lower than their gestational age in weeks [7, 8]. Vavilala et al [9] found that in infants older than 6 months of age undergoing a sevoflurane anesthesia, the lower limit of cerebral autoregulation for mean arterial pressure (MAP) was found to be 59 mmHg, which was 11 mmHg lower than baseline blood pressures. The lower limit of cerebral autoregulation of older children was also found to be 60 mmHg, but was 22 mmHg lower than baseline blood pressures. A study of children younger than 2 years undergoing sevoflurane anesthesia found that in infants <6 months of age, the lower limit of autoregulation occurred at 38 mmHg or a 20 % decrease from baseline awake MAP [10]. In contrast the same authors found that, in infants older than 6 months, the lower limit of autoregulation did not occur until blood pressure had decreased 40 %. These studies demonstrate that infants have less cerebral autoregulatory reserve and may be at the risk of inadequate cerebral perfusion following a decrease in blood pressure after anesthetic induction.
Inadequate perfusion from hypotension can lead to partial asphyxia. Most general anesthetics are associated with some degree of hypotension, which can be ameliorated by surgical stimulation. Prolonged inductions or surgical preparation times may lead to prolonged periods of hypotension in neonates. Ideally, general anesthesia should decrease cerebral metabolic rate and thus decrease the need for intraoperative neuronal substrate. However, it is not known whether common volatile and intravenous general anesthetics, which are gamma-aminobutyric acid (GABA) receptor agonists, lead to a lowered cerebral metabolic rate in young infants.
The difficulties of anesthetizing young infants can be compounded by inaccurate blood pressure monitoring. It is essential to assure a proper noninvasive blood pressure monitoring during induction and maintenance or before an invasive arterial monitoring is placed.
8.1.6 Intracranial Pressure
The Monro-Kellie doctrine states that the skull is a closed box containing the brain, blood, and cerebrospinal fluid (CSF). An increase in volume of one of these components, with an increase in intracranial pressure (ICP), will result in a compensatory reduction in the other components to counteract the change. In the infant, before cranial suture fusion, decompression can occur through an increase in skull size. The posterior fontanelle closes at about 6 months of age, and the anterior fontanelle at around 12–18 months. The final cranial suture closure may be as late as 10 years old. Increases in intracranial volume can only be accommodated if the change is gradual. Acute increases, such as after traumatic brain injury, will still result in raised ICP as in adults.
The infant may not demonstrate signs typically associated with intracranial hypertension, because the cranium can substantially expand in response to an expanding intracranial brain mass or process causing hydrocephalus, before coming to clinical attention. Early in the course, infants and young children often do not exhibit the traditional signs of intracranial hypertension such as bradycardia, elevated systemic blood pressure, dilation of the pupils, and papilledema. If present, these signal severe progression with poor outcomes. Neonates and infants may present with increased head circumference, bulging fontanelles, widened cranial sutures, “sundowning” of the eyes, irritability, drowsiness, poor feeding, or lower motor deficits.
8.1.7 Brain and Inflammation
The brain was thought not to be involved in the inflammatory processes. New studies suggest that in traumatic brain injury [11] subarachnoid hemorrhage [12], a severe cerebral inflammatory reaction, is activated. Systemic inflammatory reactions affect the brain while cerebral inflammatory processes lead to significant systemic effects. Cerebral ischemia and reperfusion injury are the main causes of brain damage because of the inflammatory reactions they start.
Currently, there is no clinical evidence to suggest that direct inflammatory modulation reduces the incidence of mortality or morbidity in patients affected with a cerebral insult [13]. The secondary effects of an inflammatory reaction such as hypoxia, hypotension, hyperthermia, and hyperglycemia are known to induce secondary brain injury and should be prevented.
8.1.8 Pediatric Electrophysiology
Children develop different pathological conditions from adults that are often age specific. The electroencephalogram (EEG) recording is different in children than adults because the brain, meninges, skull, scalp, head size, and the child’s behavior and ability to cooperate all change over time.
Therefore, pediatric EEGs must be recorded and interpreted with special attention given to the child’s age and developmental level. An EEGer must be aware of normal age-specific characteristics. For example, the normal pediatric EEG activity has more variation than the adult EEG. Focalities are not always abnormal but lack of change between states is abnormal in infants. As EEG varies greatly by age, its interpretation needs to be conceptually age specific [14–17].
8.1.9 Anesthesia and Neurotoxicity
Data supporting anesthetic neurotoxicity have been presented more than 10 years ago [18, 19]. Researchers found that exposure of developing rodents to ethanol, a known N-methyl-D-aspartate (NMDA) receptor antagonist and gamma-aminobutyric acid (GABA) receptor agonist, during a critical period of development resulted in widespread neuroapoptosis of the central nervous system [20]. Most anesthetic agents were studied for their potential negative effects via these receptors. Further studies tried to confirm the potential role of general anesthetics in neurodegeneration [21] and long-term deficits in developing monkeys [22–24]. The histologic changes induced by anesthetics exposure not only damage neurons but even oligodendrocytes [22].
Although there are evidences, it should be almost impossible to extrapolate those results to humans as any randomized controlled trial would deserve ethical consequences if the same methods should applied in neonates and children. All studies reported in the literature on general anesthetics’ neurotoxicity in children are retrospective. Most studies supporting the association between neurocognitive outcome and exposure to anesthetics showed hazard ratios less than 2 [25–28]. At the current time, retrospective human data remain hypotheses generating, rather than conclusion generating.
8.2 Perioperative Management
8.2.1 Preoperative Assessment
The preoperative assessment of the pediatric neurosurgical patient includes understanding of the underlying neurological pathology and a thorough assessment of any coexisting diseases, medications, intravascular volume status, and anesthetic history.
Children with brain tumors can have a variety of signs and symptoms that may affect the conduct of anesthesia such as drowsiness, lethargy, seizures, cranial nerve palsies, focal muscle weakness, hypothalamic-pituitary axis hormonal deficiencies, nausea, and vomiting.
Intracranial hypertension should be kept great consideration, as it can become a life threatening during induction and early phases of the anesthesia. Intense crying or screaming or fighting can cause significant elevations in ICP. Premedication with oral midazolam has shown significant benefits with no respiratory depression or change in PaCO2. Opioids may produce respiratory depression and should be used with caution in patients with elevated ICP. Ketamine should be avoided in patients with elevated ICP as it increases both cerebral blood flow (CBF) and cerebral metabolic rate.
The intravascular blood volume could be contracted because of poor intake or recurrent vomiting. Children may have abnormal airways because of associated craniofacial abnormalities. Pediatric patients have a higher risk for perioperative respiratory and cardiovascular morbidity and mortality than adults [29].
8.2.2 Induction
Infants and children without intravenous access will undergo inhalational induction. As volatile anesthetics can increase in CBF, it is important to support or control the ventilation to prevent the PaCO2 increase and offset the rise in CBF. Non-depolarizing neuromuscular blocking agent could also be administered to facilitate endotracheal intubation and prevents the increase of ICP.
Patients with lethargy or nausea and vomiting are at risk for aspiration of gastric contents and will benefit from a modified rapid sequence induction technique.
8.2.3 Monitoring
Intracranial surgery may be associated with sudden cardiovascular changes and the potential for rapid blood loss. Routine monitoring includes capnography, pulse oximetry, electrocardiography, temperature, and invasive arterial pressure. Urethral catheterization and the measurement of urine output are necessary for prolonged procedures and especially those associated with diabetes insipidus or the requirement for mannitol. A central venous catheter (CVC) provides large-bore access and allows for central administration of vasoactive drugs and potentially treatment of venous air embolism (VAE). Readings can be unreliable in small children in the prone position but trends may be useful.
Precordial Doppler ultrasonography could be useful for VAE detection.
Neurophysiological monitoring may be utilized with the aim to improve outcome and reduce morbidity by early detection of neurological injury at a point when damage can be limited or reversed. In brief, the modalities for monitoring include EEG, somatosensory evoked potentials (SSEPs), motor evoked potentials (MEPs), and transcranial Doppler (TCD).
8.2.4 Positioning
Proper position of the patient for pediatric neurosurgical procedures is imperative to ensure both patient safety and comfort. Most patients undergoing neurosurgery are in a supine position. The flexion of the neck may result in downward migration of the endotracheal tube or occlusion of jugular venous drainage, causing cerebral venous hypertension and increased intracranial volume and pressure. The prone position raises the risk of eye injury from direct ocular pressure and hypoperfusion. The park-bench position is utilized for lateral or midline incisions and when quick access to the patient is needed. Appropriate padding and stabilization is required to prevent stretch, ischemia, and pressure injury to the axilla as well as other parts of the body. The sitting position requires careful attention to padding pressure points as well as securing the patient on the bed to ensure patient safety and surgical stability.

Full access? Get Clinical Tree
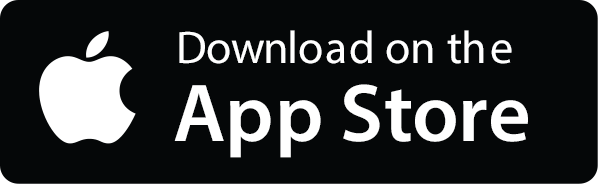
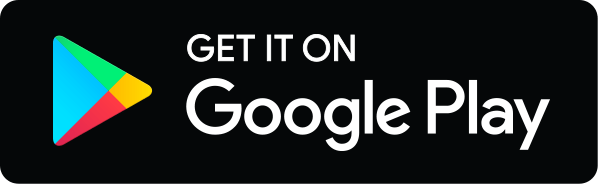