Chapter 86
Perioperative Approach to the High-Risk Surgical Patient 
Stress Response to Acute Injury
Acute injury results in a characteristic biphasic set of physiologic and metabolic changes, collectively described as the stress (or inflammatory) response (Figure 86.1). The initial period, which Cuthbertson termed the ebb phase in his original description, is associated with decreased cardiac output, a reduction in blood flow to organs other than the brain and central nervous system (CNS), hypothermia, and an overall decrease in the resting energy expenditure (REE). In modern parlance this is a stage of shock. As is most often the case with shock, the transition from the ebb phase is rapid when adequate resuscitation is provided. The subsequent period, or flow phase, is characterized by fever, an increase in blood flow to most tissues, and an increased REE. Virtually all variables associated with acute inflammation peak on or about postinjury day 2 and then decline to baseline by postinjury day 6 to 7 (Table 86.1). Deviations from this expected pattern indicate the influence of preexisting medical conditions or postoperative complications.
TABLE 86.1
Increases in Organ System Parameters after Major Surgery
The time course of these changes parallels the response trajectory described in Figure 86.1.
Figure 86.1 Changes in resting energy expenditure (REE) over time following injury. Other “stress” indicators follow a similar time course (see text and Table 86.1). The arrow indicates onset of injury or surgical procedure.
Hypermetabolic Phase
The increase in energy expenditure (hypermetabolism) that characterizes the flow phase reflects the initiation of processes to repair damaged tissue. The increase is driven primarily by the metabolic activities of inflammatory cells. These cells are obligate glucose users. A continuous supply of glucose is provided by enhanced hepatic gluconeogenesis and glycogenolysis. Skeletal (somatic) and visceral (smooth) muscles are catabolized into amino acids to be used as substrate for the synthesis of structural proteins and enzymes. The energy needs of other organs are primarily met by the oxidation of fatty acids. This global increase in metabolism is reflected in a rise in REE, oxygen consumption, and carbon dioxide production. In the flow phase, peripheral vascular tone decreases and cardiac output increases to provide flow to injured tissue and deliver nutrients to muscle and the liver. Minute ventilation rises in proportion to the increase in carbon dioxide production, keeping Paco2 in the normal range. However, vascular dilation and capillary recruitment are insufficient to support the delivery of substrate to damaged tissue. Most injured tissue is essentially avascular, and substrate delivery is primarily a function of diffusion across a concentration gradient into the interstitium. To increase substrate delivery to a wound, capillaries become “leaky” and fluid is lost to the extravascular compartment. This results in intravascular fluid depletion. To compensate there is an increase in renal salt and water retention and fluid translocation from the intracellular compartment (Figure 86.2).
Postoperative Issues
Concurrent diseases may have their greatest impact by limiting responses during the hyperdynamic phase. The magnitude of these changes depends on the extent of the initial injury as well as on preexisting diseases (Table 86.2). Three important factors have an impact on the patient’s ultimate outcome. The first factor is the ability of the patient to mount a hypermetabolic response. In some cases, this requires high levels of hemodynamic support. In others, however, the intensive care unit (ICU) physician’s role in management is simply to support the hypermetabolic response, particularly by replacing ongoing fluid losses (especially those lost into the “third space”). This allows the cardiovascular system to become hyperdynamic, which, in turn, supports blood flow and oxygen delivery to the wound and major organ systems such as the liver and kidneys.
TABLE 86.2
Disease States Compromising the Extent of the Hyperdynamic Response
Organ System | Disease |
Cardiovascular | Coronary artery disease |
Myocardial pump dysfunction | |
Valvular heart disease | |
Peripheral vascular disease | |
Rhythm disturbance with reduced cardiac output | |
Pulmonary | Emphysema |
Restrictive lung disease | |
Reactive airway disease | |
Neuromuscular | Altered mental status, confusion |
Stroke | |
Myopathies | |
Myasthenia gravis | |
Muscular deconditioning | |
Gastrointestinal: metabolic | Nutritional deficiencies |
Malabsorption syndromes | |
Renal insufficiency | |
Hepatic synthetic disorder, cirrhosis | |
Endocrine | Addison disease (or inadequate adrenal cortical function resulting from chronic corticosteroid use) |
Hypothyroidism |
Specific Perioperative Interventions
Correcting Volume Deficits
Assessing and achieving proper fluid balance in critically ill patients can be difficult (Chapter 7). This problem is amplified in patients with major preexisting medical disorders (see Table 86.2). Additionally, some patients appear to be in fluid balance or even overloaded when, in fact, a relative intravascular volume deficit exists. For example, the use of diuretics in patients with congestive heart failure or hypertension can lead to total body deficits of water, sodium, and potassium. These may result in an inability to mount an adequate postoperative hyperdynamic response.

Full access? Get Clinical Tree
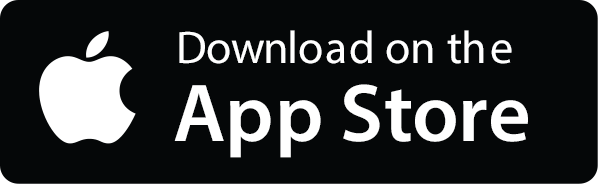
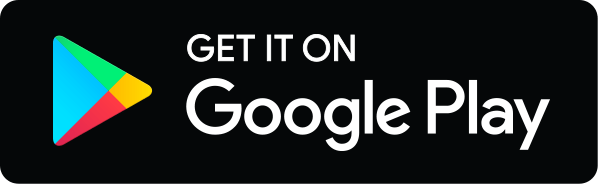