where E = required stimulating current, K = constant, Q = minimal required stimulation current, and r= distance between electrode and nerve.
Empirically, motor response to stimulation with current below 0.5 mA with pulse duration of 0.1 ms signifies that the needle’s tip is sufficiently close to the nerve to translate to a high block success rate.
Clinical Pearls
According to Coulomb’s law, if a motor response can be elicited at very low amperage (<0.5 mA), then the stimulating electrode must be very close to the nerve. Stimulation at very low amperage maximizes specificity.
According to Coulomb’s law, if a motor response can be elicited at very low amperage (<0.5 mp), then the stimulating electrode must be very close to the nerve. Stimulation at very low amperage maximizes specificity. In contrast, using a higher amperage (eg, 2–5 mA), maximizes sensitivity. This principle is used when monitoring the neuromuscular function by cutaneous electrodes and comparably very high (≈50 mA) currents during general anesthesia. Here, specificity of electrode location relative to the nerve is of less importance. For peripheral nerve location, 2- to 5-mA currents increase sensitivity, providing clues at a distance, but ultimate specificity is achieved by successful stimulation at very low current (<0.5 mA). However, it should be noted that using current of high intensity has practical disadvantages in that (1) it is associated with patient discomfort and (2). higher current intensity (eg, >1.0 mA) is sufficient to elicit direct muscle stimulation, which may produce confusing twitches of the local muscles. For these reasons, many nerve localization procedures are best initiated using relatively lower initial stimulating current (eg, <1.5 mA).
Figure 45–1. Electrical current from the stimulating microelectrode tip dissipates quickly, to the inverse square to the distance from the nerve. A: Needle tip position within 1 mm of nerve at minimal current amplitude (amperage) required for stimulation. B: Movement of needle tip to position just 4 mm from nerve results in a 16-fold increase in the amount of current required for stimulation.
The surface area of the conductive electrode and its conductance are very important in nerve stimulation according to Ohm’s law: I = V/ R, where I = current flow (amperage), V = potential difference (voltage), and R = resistance (ohms).
The nerve stimulator varies current flow by altering the voltage. Most modern nerve stimulators are constant-current generators that automatically adjust the voltage output to maintain the set current flow despite changes in tissue resistance (within a certain range specified by the electrical design of the nerve stimulator).
Resistance, on the other hand, is related to the conductive area of the electrode by the equation R = ρL /A, where R = electrical resistance, ρ = tissue resistivity, and A = conductive area. An example of the clinical importance of this principle is the use of defibrillating paddles. Defibrillation paddles are characterized by a large surface area to minimize impedance or resistance. By contrast, the microelectrode tip of a shielded block needle serves to maximize resistance outside of the microconductive area. This ensures that the electrode tip must be very close to the nerve if a motor response is to be elicited, thereby enhancing specificity.
Clinical Pearls
If a motor response can be elicited at (1) very low amperage and by Ohm’s law, with a (2) microelectrode (small conductive area), then the stimulator electrode must be very close to the nerve.
If a motor response can be elicited at (1) very low amperage and, by Ohm’s law, with a (2) microelectrode (small conductive area), then the stimulator electrode must be very close to the nerve. This phenomenon has led to the clinical use of needles with electrically insulated shafts to ensure specificity of location to the microelectrode (needle’s tip) relative to the targeted nerve. Bashein and colleagues4 looked at the difference in the relative dispersion of current between electrically shielded and unshielded needles. Indeed, the ability to elicit a motor response to electrical stimulation following the initial elicitation of a mechanical paresthesia differs from 40%, using noninsulated needles, to 10% with insulated needles.5 The 30% increase in the ability to cause motor nerve stimulation with a noninsulated needle compared with an insulated needle can be explained by the difference in current dispersion between the two needles (see Chapter 5, Electrophysiology of Nerve Stimulation, for more information).
Electrical Pulse Duration
Electrical pulse duration refers to the duration of the periodic pulsed square wave generated by the nerve stimulator. For the purpose of nerve localization, short pulse duration ranging between 0.05 and 1 ms is typically used in clinical practice, with 0.1 ms being most common. Increasing electrical pulse duration increases the total flow of electrons (electrical energy) proportional to the calculated area under the curve (Figure 45–2). Increasing the duration of the electrical pulse therefore results in increased ability to stimulate the nerve without changing other variables. Similar to current flow (amperage), higher pulse durations of 0.3 to 1.0 ms also result in enhanced sensitivity for transcutaneous or initial invasive prelocation of the nerve. By contrast, by using a lower pulse duration, specificity is maximized. This principle has been demonstrated clinically when higher current amplitude (amperage) was needed to elicit a motor response at lower pulse duration (Figure 45–3).6
Figure 45–2. Graph of current in milliamperes (y-axis) versus time in milliseconds (x-axis). Increasing pulse duration from 0.1 to 1.0 ms at the same amperage (0.5 mA in this example) results in a larger area under the curve and a larger pulse of electrons, increasing the ability to stimulate the nerve transcutaneously without patient discomfort.
Tissue Electrical Impedance
Clinical Pearls
Condensing the tissues by indenting the overlying skin, adipose, etc toward the nerve serves to decrease electrical impedance, increasing conductance.

Full access? Get Clinical Tree
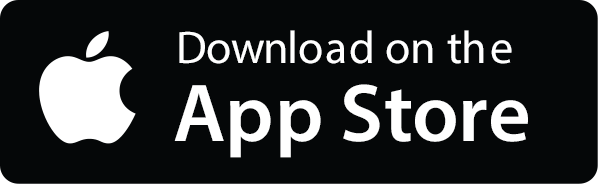
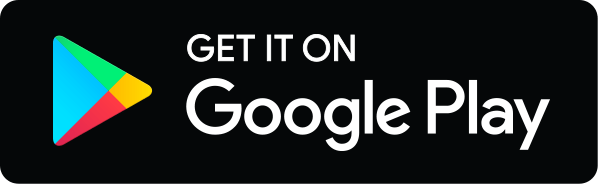