Chapter 72 Pediatric Renal Replacement Therapy in the Intensive Care Unit
Renal replacement therapy has an established role in the pediatric intensive care unit (ICU).1 Volume overload and metabolic imbalance can complicate the course of critically ill patients, especially those with multiorgan dysfunction.2 Some patients with normal kidney function may have metabolic imbalance or intoxication that overwhelms baseline endogenous clearance capacity, necessitating extracorporeal clearance techniques. Improvement of techniques coupled with the realization that early supportive therapy may improve outcomes have led to expanded use of renal replacement therapy for critically ill pediatric patients.
Renal Failure and Other Indications for Renal Support
Acute Kidney Injury
Acute kidney injury (AKI) can be defined as an acute decrease in the glomerular filtration rate. Oligoanuria is a frequent, but not essential, component of AKI. Common causes of AKI in the critically ill patient include renal hypoperfusion (leading to so-called prerenal azotemia), hypotension or shock causing renal ischemic injury or acute tubular necrosis, nonspecific renal involvement as part of a sepsis syndrome or multiorgan dysfunction, and renal injury from nephrotoxins such as antibiotics and radiocontrast media (see Chapter 71).
Patients with even mild AKI can have volume overload, electrolyte imbalance, and metabolic derangement with associated poor outcomes.3–5 Such complications can be particularly detrimental to the care of the critically ill patient. While all modalities of renal replacement therapy can correct these abnormalities, certain modalities may be better suited for specific pediatric clinical situations.
Acute Intoxication and Metabolic Disorders
Hemodialysis can rapidly remove many exogenous toxins (e.g., as the result of poisonings and drug overdoses) and endogenous toxins (such as those seen in persons with inborn errors of metabolism), and it is often the therapy of choice for severe life-threatening intoxication. Hemodialysis can be followed by continuous renal replacement therapy (CRRT) for intoxications where a rebound phenomenon may occur (see Chapters 76 and 105).
Renal Support
Diminished renal function is a common component of multiorgan dysfunction syndrome. AKI is highly correlated with poor outcome in hospitalized adults and children.4,6 In pediatric patients, studies demonstrate increased mortality in critically ill children with excessive levels of fluid overload in the setting of concomitant AKI.7–10 These observations, coupled with advanced capabilities for renal replacement therapy, lead many clinicians to consider early initiation of renal support. In this model, traditional indicators for dialysis, such as profound azotemia, oligoanuria, massive fluid overload, or significant electrolyte imbalance, may occur too late in the clinical course to alter outcome. Earlier intervention, either with renal replacement therapy or perhaps through careful conservative management, may prevent complications associated with serious metabolic disarray and volume overload and permit vigorous nutritional and medical support. The concept of early “renal support” for patients in the ICU to limit metabolic derangement and prevent volume overload has gained wider acceptance in both the adult and pediatric critical care arenas.
Conservative Management
Conservative management of AKI includes optimization of clinical status with the maintenance of fluid balance, renal perfusion, cardiac output, and adequate blood pressure. Nephrotoxin exposure should be limited. Judicious use of diuretics can greatly augment the patient’s ability to maintain fluid homeostasis. After initial early and goal-directed fluid resuscitation in patients with hemodynamic shock, fluids may need to be restricted to prevent worsening degrees of fluid overload.11,12 Patients require careful dietary management to provide sufficient nutrition in a smaller daily fluid volume and to avoid excess delivery of substances normally cleared through the kidney (e.g., potassium and phosphorus).
Conservative management avoids the potential risks associated with renal replacement modalities; the cases of many pediatric patients with milder renal dysfunction can be managed successfully without renal replacement therapy. As noted previously, however, support exists for the use of renal replacement early in the ICU course. Data show improved outcomes for pediatric patients receiving CRRT who demonstrated less fluid overload at initiation of therapy,7–1013 suggesting a survival advantage for patients who begin CRRT earlier rather than later. Although the best time to initiate renal replacement for the patient in the ICU remains unclear, there is a growing consensus to avoid unnecessary delay.14
Basic Physiology of Dialysis and Ultrafiltration
Diffusion describes the movement of dissolved particles across a semipermeable membrane from an area of high concentration to an area of low concentration (Figure 72-1). This physical principle operates in all renal replacement modalities in which dialysis fluid is used. Diffusion favors the movement of smaller particles and is most rapid when the concentration gradient across the semipermeable membrane is greatest; diffusion stops when the concentrations achieve equilibrium.
Convection occurs when dissolved particles pass across a semipermeable membrane because of the effects of a pressure gradient (Figure 72-2). Particles that are smaller than the pores of the membrane can pass freely, whereas larger particles are restricted. Because particles and water are moving together, the removed solution is isotonic to the original.
Peritoneal Dialysis
Peritoneal dialysis (PD) has been a standard form for renal replacement therapy for many years. Despite improving techniques that permit the application of other modalities to even the smallest patients, PD remains an effective method of renal replacement therapy and may represent the best choice in selected cases.15 Even the most experienced centers may have difficulty achieving vascular access in some patients. Children with vascular abnormalities, certain types of cardiac disease, or hemodynamic issues may be suboptimal candidates for extracorporeal perfusion through a hemodialysis or hemofiltration circuit. In such circumstances, PD can be the best choice for renal replacement therapy.
Physiology
It has been known for many years that the peritoneum can be used as a dialyzing membrane.16 Instillation of a dialysis fluid into the peritoneal space permits diffusion of particles out of the blood and into the dialysis fluid across the peritoneum, which acts as a semipermeable membrane. Through the use of a hypertonic solution, water also passes across the membrane, generating an ultrafiltrate. Water movement will also tend to drag particles across the peritoneum by convection. After the dwell is complete, the spent dialysate is drained from the abdomen and fresh dialysis fluid is introduced.
Indications
PD can remove excess fluid and provide volume control in patients with oligoanuria. Compared with fluid removal with intermittent hemodialysis, fluid removal with PD is much slower. Manipulation of dialysis fluid osmolality and dwell time can adjust the quantity of volume removed. The slow, steady ultrafiltration achieved with PD may be preferable to the rapid fluid removal that occurs in intermittent hemodialysis, particularly in unstable, critically ill patients.
Technique
Flexible catheters are most often used for chronic PD in pediatric patients. For acute PD, either this form of a surgically placed catheter or percutaneously inserted temporary PD catheters may be used; some data suggest that fewer complications occur with surgically placed catheters.17 Catheters come in a variety of sizes, depending on the size of the patient. Local practice often determines who will insert the catheters when they are needed; the procedure requires expertise to ensure proper functioning of the catheter.
PD fluid comes in standardized, sterile bags with premixed formulations, and thus pharmacy preparation is unnecessary. Because most patients with renal failure have metabolic acidosis, the dialysis fluid contains base, usually in the form of lactate. Thus the PD system will remove unwanted particles and also can act as a source of electrolytes. Lactate absorption can lead to confusion regarding acid/base interpretation, especially in critically ill infants. In such settings bicarbonate-based dialysis fluid may be preferable,18 although this fluid may require extemporaneous preparation by the local pharmacy.
Dialysis fluid should be warmed to body temperature before instillation. This step is particularly important in small patients, in whom hypotension associated with cold dialysis fluid infusion can develop.19
Initial exchanges with a new PD catheter should use relatively lower volumes of dialysis fluid (10 to 20 mL/kg; <500 mL/m2) to limit the chance of leakage from the catheter entrance operative site. Volumes may increase gradually during the next few days or weeks to 1100 mL/m2.19
Disadvantages and Complications
Peritonitis must always be considered in a patient undergoing PD who has a fever or cloudy effluent. Dialysate should be analyzed for cell count, Gram stain, and bacterial culture if infection is suspected. Empirical or specific antibiotic therapy can be placed in the dialysate to treat peritonitis via the intraperitoneal route.20
Dialysate can fail to fill or drain through the PD catheter because of a number of potential problems, including kinking of the catheter, fibrin plugs, omental obstruction, or catheter malposition. Percutaneously inserted temporary dialysis catheters are more prone to malfunction than are surgically placed catheters.21 Abdominal x-ray images can confirm appropriate positioning and permit checks for kinks in the catheter. Some success has been reported with thrombolytic agents to treat fibrin plugging.22–24 Revision or replacement of the catheter may need to be considered if simple maneuvers do not correct the malfunction.
Perforation of abdominal or pelvic structures can occur, either at the time of initial catheter placement or later.21 Although this event is relatively uncommon, significant morbidity can result.
Fluid leakage is seen most often with dwell volumes that are too large, especially in the period immediately after catheter placement.25 Lower fill volumes should be used. Fluid leakage into the thorax can compromise respiration. External fluid leakage around the catheter increases the risk of infection.
Intensive Care Unit Issues
Patients undergoing PD can lose protein into the dialysate. Nutritional support must provide sufficient protein to compensate for this loss. High dextrose concentrations in the dialysis fluid can cause hyperglycemia; administration of insulin may be necessary. Indwelling dialysis fluid causes increased intraabdominal pressure that can complicate care of the critically ill patient. Diaphragmatic excursion may be limited, and venous return can be reduced. Stomach compression can lead to gastroesophageal reflux. Although patients undergoing long-term PD who receive fewer daily exchanges usually require maximal fill volumes to achieve adequate dialysis, patients undergoing short-term PD may do better using submaximal fill volumes with more frequent exchanges provided around the clock.
Intermittent Hemodialysis
Intermittent hemodialysis (IHD) has been the traditional form of renal replacement therapy in the ICU. The technique is well established for pediatric patients.26 IHD offers the advantages of high efficiency for rapid metabolic correction and fluid removal. Its very advantages, however, may limit IHD’s usefulness in the critically ill patient. IHD for infants and small children can be technically difficult and demanding. IHD may be the preferred modality for some critically ill pediatric patients; successful treatment in this setting requires experienced personnel.
Physiology
Analogous to the process of diffusion, ultrafiltration during IHD removes fluid only from the vascular compartment. Extravascular or “third space” fluid must move into the vascular compartment for removal by ultrafiltration. When the rate of movement from the extravascular space is slower than the rate of ultrafiltration, intravascular depletion can occur even though total body water remains elevated (Figure 72-3). This “two-compartment” model represents a potential limitation of rapid ultrafiltration during IHD, especially in the critically ill patient.
< div class='tao-gold-member'>

Full access? Get Clinical Tree
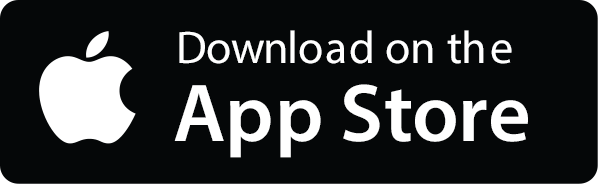
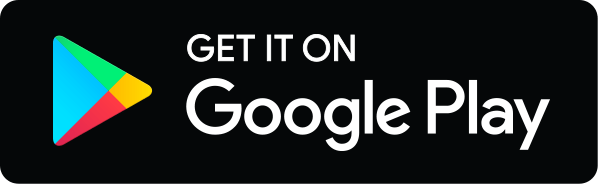