2. Systemic vascular tone is low in children up to 8 years of age, as evidenced by the lack of a change in blood pressure when caudal or epidural blocks are administered.
3. Both heart rate and blood pressure increase with increasing age in childhood (Table 42-3).
TABLE 42-2 AIRWAY ANOMALIES THAT MAY PRESENT PROBLEMS DURING ANESTHESIA
Pierre Robin syndrome (micrognathia, glossoptosis, airway distress in first 24 hrs): Typically easy intubation by 2 yrs of age
Treacher Collins syndrome (progressively more difficult airway management with increasing age)
Crouzon disease, Apert disease, and Down syndrome (difficult mask ventilation but tracheal intubation is straightforward)
TABLE 42-3 NORMAL RANGE OF RESTING HEART RATES AND BLOOD PRESSURE IN CHILDREN
C. Central Nervous System
1. Oxygen consumption in the brains of children (5.5 mL/100 g/min) is 50% greater than that in adults (3.5 mL/100 g/min). In children 6 months to 3 years of age, the cerebral blood flow is 50% to 70% greater than in adults (50 mL/min/100 g vs. 70–110 mL/min/100 g).
2. Apoptosis. Most general anesthetics (with the exception of xenon, dexmedetomidine, and opioids) and sedatives, that also act on N-methyl-d-aspartic acid (NMDA) and GABAA receptors, cause apoptosis in newborn rodents and nonhuman primates after relatively prolonged exposure (>12 hours) and when administered in combination.
a. Brief anesthesia with ketamine (≤3 hours) or isoflurane (<2 hours) fails to induce neurocognitive dysfunction.
b. Whether the rodent and nonhuman primate evidence of anesthetic-induced neurotoxicity is applicable to humans remains hotly debated. (Because most anesthetics in young children last <3 hours, these data may have limited validity in humans.)
c. Also, the doses of intravenous (IV) anesthetics and sedatives in rodents and primates are up to 10-fold greater than in humans.
d. Studies in humans who received anesthesia at a young age suggested that cognitive disability in those who received anesthesia before the age of 3 years may be more prevalent than in those who did not. However, most of those studies were seriously flawed in terms of their design, and a large cohort of identical twins who were discordant for general anesthesia before 3 years of age in one study, when tested for intellectual aptitude 10 years later, were similar.
II. PHARMACOLOGY
A. Developmental Pharmacology
1. Age may have a substantial effect on the absorption of certain drugs, for example, because gastric juice is closer to neutral pH (pH ∼6) at birth and does not reach adult levels of acidity until about 3 years of age.
2. The albumin concentration is low in children, and α1-acid glycoprotein concentration is also reduced at birth and increases slowly with increasing age as well as during periods of stress. (Free fraction of medications such as lidocaine is greater in young infants than older children because the concentration of α1-acid glycoprotein in the former is reduced.)
3. Apart from a few enzyme systems that hold importance in fetal life (CYP450 3A7), the activities of the vast majority of CYP450 enzyme systems (CYP450 3A4, 2E1, and 2D6) increase with age from birth but at divergent rates (Fig. 42-1).
FIGURE 42-1. Developmental changes in common cytochromes of interest in pediatric anesthesia.
TABLE 42-4 FACTORS INCREASING THE WASHIN OF INHALATIONAL ANESTHETICS IN CHILDREN COMPARED WITH ADULTS
Alveolar ventilation:FRC ratio (5:1 in infants vs. 1.5:1 in adults)
Greater distribution of cardiac output to the vessel-rich group in infants (vessel-rich group comprises 18% of the body weight in infants compared with 8% in adults)
Reduced tissue solubility in infants
Reduced blood solubility in infants
FRC = functional residual capacity.
4. The glomerular filtration rate is markedly reduced in neonates but matures throughout childhood, reaching adult rates by 5 to 15 years of age.
B. Inhalational Anesthetics
1. The widespread appeal of the current inhalational anesthetics may be attributed to their physicochemical properties, which provide a rapid onset and offset of action, cardiorespiratory homeostasis, and limited metabolism and toxicity (Table 42-4).
2. Sevoflurane is the induction agent of choice in infants and children. Desflurane offers the most favorable pharmacokinetic and in vivo metabolic characteristics in terms of its minimal blood and tissue solubilities and resistance to metabolism, although its use as an induction agent in children is proscribed because it irritates the upper airway.
C. Pharmacokinetics
1. The greater ratio of alveolar ventilation to functional residual capacity (FRC) in infants and children compared with adults speeds the equilibration of inspired to alveolar anesthetic partial pressures. The net effect of a greater ventilation: FRC ratio is a reduction in the time constant, from 0.7 in adults to 0.2 in infants, which explains the speed of equilibration (sudden and rapid responses observed to inhalational anesthetic in infants and children compared with adults).
2. The greater cardiac output in infants speeds the equilibration of alveolar to inspired anesthetic partial pressures in the vessel-rich group, which takes up most of the anesthetic during the first couple of hours of anesthesia.
3. The solubility of all inhalational anesthetics is reduced in tissues in infants compared with adults, including in the brain, muscle, and heart.
FIGURE 42-2. Age and the minimum alveolar concentration (MAC) of isoflurane from premature infants to adults. (Reproduced with permission from LeDez KM, Lerman J. The minimum alveolar concentration (MAC) of isoflurane in preterm neonates.)
D. Pharmacodynamics
1. As a fetus matures and reaches birth, the minimum alveolar concentration (MAC) increases, peaking in infants 1 to 6 months of age isoflurane and then decreases steadily thereafter with increasing age (Fig. 42-2). (The reason for the age-dependent differences in MAC is unclear.)
2. The MAC of desflurane in adult redheads is 20% greater than in nonredheads; the same response would be expected in children of similar genetic predisposition.
III. RESPIRATION. All anesthetics, when administered in the absence of surgical stimulation, depress respiration and minute ventilation in a dose-dependent manner by decreasing tidal volume and increasing respiratory rate.
A. Airway resistance increases during desflurane anesthesia and decreases during sevoflurane anesthesia. (In children with asthma, the former is best avoided, and the latter is preferred.)
B. Upper airway responses to inhalational anesthesia (by mask) depend on both the concentration and the particular anesthetic administered. (Whereas sevoflurane does not trigger these reflex responses, isoflurane and desflurane are most irritating to the airway, particularly at >1 MAC concentrations.) The package insert for desflurane cautions against using it for inhalational inductions in children.
IV. CARDIOVASCULAR. Inhalational anesthetics depress the heart in a dose-dependent manner.
A. In the past, anticholinergics were commonly used to prevent bradycardia and arrhythmias in children given halothane; however, this practice is no longer necessary with the newer ether anesthetics that infrequently cause arrhythmias.
B. Sevoflurane maintains or increases heart rate during induction of anesthesia in most instances likely because of withdrawal of vagal tone. Desflurane and isoflurane tend to increase the heart rate.
C. Cardiac output in young children is dependent on heart rate.
D. Peripheral vascular resistance is very low in children as evidenced by the absence of a change in blood pressure when a caudal/epidural block is administered.
V. CENTRAL NERVOUS SYSTEM. All inhalational anesthetics decrease cerebral vascular resistance and the cerebral metabolic rate for oxygen.
A. Autoregulation of cerebral blood flow in children of all ages is similar to that in adults, although it occurs at greater blood flow rates. The lower limit of autoregulation in children of all ages appears to be similar (60 mm Hg mean arterial pressure).
B. Bispectral index readings are imprecise in children < 5 years of age.
VI. RENAL. Inhalational anesthetics do not exert substantive effects on the kidneys in children except through their metabolism, with the kidneys being a site of degradation of inhalational anesthetics.
VII. HEPATIC. There are little data regarding the effects of inhalational anesthetics on hepatic function in children. However, isolated cases of hepatic dysfunction in children have been reported after every inhalational anesthetic.
VIII. IN VIVO METABOLISM. Degradation of inhalational anesthetics in the presence of carbon dioxide absorbents has been the subject of intense research and concern in both adults and children.
IX. INTRAVENOUS. IV drugs are distributed first to the vessel-rich group, just as inhalational anesthetics are and then to the muscle, vessel-poor group, and fat groups. The primary anesthetic effect occurs when the anesthetic reaches an adequate brain concentration; it is then redistributed to other tissues and metabolized to terminate its action.
A. Propofol is the most commonly used IV induction agent in children. Propofol is an integral part of total intravenous regional anesthesia (TIVA) for maintenance of anesthesia in children undergoing medical or radiologic evaluations and surgery.
1. Propofol is the only anesthetic that has antinausea properties that may be exploited for use in children with histories of nausea and vomiting or who are undergoing emetogenic surgery.
2. Although propofol is used for both sedation and general anesthesia, long-term sedation in infants and children is not recommended with propofol after reports of unexpected death during propofol sedation with >4 mg/kg/hr for >48 hours.
B. Ketamine is a phencyclidine derivative that offers enormous flexibility in the clinical care of children. This anesthetic can be used as a premedication (orally, nasally, rectally, or intramuscularly [IM]), a general anesthetic induction agent (IV or IM) and maintenance agent as an infusion, as a sedative (IV or IM), or as a neuraxial analgesic (caudal/epidural).
C. Neuromuscular Blocking Drugs. With standard twitch devices readily available, every child who receives a muscle relaxant should be assessed for twitch response before attempting to antagonize the neuromuscular blockade. The role of neuromuscular agents in children has diminished with the demise in routine use of succinylcholine and the adoption of propofol as the adjunctive medication to facilitate tracheal intubation after induction of anesthesia with sevoflurane.
1. Succinylcholine is the only depolarizing muscle relaxant in clinical practice and remains the agent that provides the most rapid onset and offset of paralysis without additional medications to recover the normal twitch response.
a. The IV dose of succinylcholine is 3 to 4 mg/kg in neonates and infants, 2 mg/kg in children, and 1 mg/kg in adolescents. The larger dose requirement with decreasing age has been attributed to the larger volume of distribution in younger infants.
b. Side Effects. The salient side effects associated with succinylcholine are arrhythmias (most notably bradycardia), rhabdomyolysis (with hyperkalemia and myoglobinuria), raised intraocular pressure, fasciculations, and malignant hyperthermia.
2. Rocuronium. The potency of rocuronium is greatest in infants, least in children, and intermediate in adults (ED95 in infants is 0.25 mg/kg and in children, 0.4 mg/kg).
a. In healthy children during sevoflurane anesthesia, 0.3 to 0.4 mg/kg of rocuronium provides suitable intubating conditions in 2 to 3 minutes and permits antagonism within 20 minutes. Twice the ED95 or 0.6 mg/kg IV of rocuronium, provides relaxation in 1 to 1.5 minutes.
b. At three to four times ED95, 0.9 to 1.2 mg/kg of IV rocuronium yields similar intubating conditions to succinylcholine within 60 seconds and may be used for rapid sequence induction (RSI), although recovery may be markedly prolonged.
3. Neostigmine. Antagonizing neuromuscular blocking agents is recommended in infants and children when extubation is planned, provided the time interval from the last dose has not exceeded 2 hours. (The train-of-four should be ≥0.9 before the trachea should be extubated.)
a. The dose of neostigmine in infants and children is 30% to 40% less than that in adults, or 20 to 40 μg/kg, which should be administered when at least one twitch is present in the train of four. If recovery of neuromuscular blockade is incomplete, repeat doses of neostigmine may be administered up to 70 μg/kg.
b. Neostigmine should be preceded by an anticholinergic, 20 μg/kg of atropine, or 10 μg/kg of glycopyrrolate, to minimize the effect of neostigmine on the nicotinic receptors.
4. Sugammadex is a cylindrical oligosaccharide that uniquely binds rocuronium (and to a lesser extent vecuronium) to eliminate its activity. The rocuronium–sugammadex complex is excreted unchanged in the kidney.
D. Opioid use in infants and children has increased dramatically in the past few decades in response to recognition that children experience pain and that pain adversely affects the speed and quality of recovery.
1. Morphine. In children, perioperative analgesia is accomplished with intraoperative IV doses of 50 to 100 μg/kg and postoperative doses of 50 μg/kg morphine. Morphine may also be administered via the caudal/epidural route (25–50 μg/kg provides prolonged analgesia).
2. Fentanyl is the most widely used intraoperative analgesic in children (used via the IV, IM, oral, intranasal, and caudal/epidural routes).
3. Remifentanil is a unique μ-receptor opioid that undergoes spontaneous degradation in blood by tissue esterases, with an elimination half-life of approximately minutes that is independent of the duration of infusion (administered as a continuous infusion). The infusion rate for remifentanil ranges from 0.05 to 0.25 μg/kg/min with the dose adjusted according to the presence of concomitant medications (inhalational anesthetics).
4. Codeine has been the mainstay of postoperative analgesia in children for decades.
a. The analgesic effect of codeine depends on its conversion to morphine and possibly to hydrocodone. Because this conversion depends on CYP450 2D6, polymorphisms of this enzyme may produce enormous variability in the analgesia from this drug ranging from no analgesia to an opioid overdose.
b. Use of codeine is under increasing scrutiny because of these polymorphisms and the potential extreme responses.
E. Nonopioid Analgesics
1. Acetaminophen is a nonsteroidal analgesic/antipyretic that has been an effective supplement in children.
a. Acetaminophen has no anti-inflammatory properties and is free of platelet inhibiting properties.
b. Oral doses of 10 to 15 mg/kg or rectal doses 30 to 40 mg/kg yield adequate blood concentrations. Absorption after oral administration is rapid (∼10–15 minutes), but after rectal administration, it is slow and variable (1–2 hours).
2. Ketorolac (0.5 mg/kg) is the only parenteral nonsteroidal anti-inflammatory (NSAID) medication available for use in children. It possesses anti-inflammatory and analgesic properties for mild to moderate perioperative pain, and similar to other NSAIDs, it also inhibits platelet adhesion by reducing the synthesis of thromboxane.
3. Diclofenac is almost twice as effective for acute pain than acetaminophen for the treatment of acute pain during and after surgery. (The IV dose is 0.3 mg/kg, and the oral dose is 1 mg/kg.)
X. SEDATIVES
A. Midazolam is the most widely used anxiolytic in children (PO, sublingual, nasal, IV, IM, and rectal routes) that is water soluble, with a rapid onset of action when administered orally and a brief elimination half-life.
B. Dexmedetomidine is an α2-agonist sedative whose relative affinity for α2:α1 receptors is eightfold greater than clonidine. The oral dose, which requires 30 to 60 minutes to provide sedation, is 2.6 to 4 μg/kg.
XI. PREOPERATIVE ASSESSMENT
A. Fasting Guidelines (Table 42-5).
1. Gastric emptying times after breast milk and formula have only been evaluated in infants; there are no data for comparable emptying times in children (≥1 year of age).
2. The gastric fluid characteristics in the child who is chewing gum are not substantially different from those in the fasted child. The child must expectorate the gum before proceeding with general anesthesia.
B. Laboratory Testing
1. Preoperative laboratory testing (including urinalysis and hematocrit) is infrequently ordered in healthy children without pre-existing medical disease.
2. A preoperative pregnancy test is required before anesthesia and sedation in most girls of childbearing age.
C. Medical Conditions (Table 42-6)
TABLE 42-5 FASTING GUIDELINES FOR CHILDREN REQUIRING ELECTIVE ANESTHESIA
*Includes clear tea. If milk is added, recommend a 6-hour fast.

Full access? Get Clinical Tree
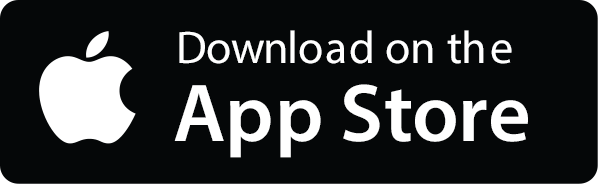
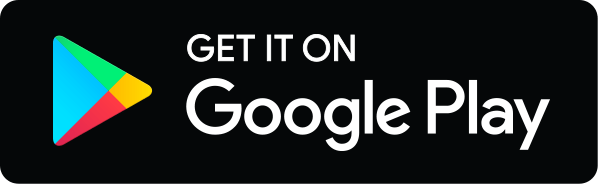