Chapter 48
Pediatric Anesthesia
Pediatric Anatomy and Physiology
A thorough understanding of the anatomic and physiologic differences between pediatric and adult patients is of paramount importance in providing safe anesthesia care. Because of the immaturity of children’s organ systems—which is most significant until 2 years of age—the psychological and physical stress of surgery, the effects of anesthetic medications, and related pathology all play an integral role in patient outcomes. A thorough discussion of anatomy and physiology for the pediatric patient is presented in Chapter 47.
Pediatric Pharmacologic Considerations
A complete discussion regarding the recent concern over possible cognitive changes associated with anesthetic drugs in developing children is included in Chapter 47.
Water freely diffuses across cell membranes and is essential for the transport of cellular nutrients and substrates that support metabolic reactions. Total body water (TBW), expressed in liters, is determined as a percentage of total body weight (1 L of water weighs 1 kg) and steadily decreases with increasing age and varies according to sex and body habitus. Total body water is distributed into the intracellular (ICF) and extracellular (ECF) fluid compartments. With maturation, there is an accompanying decrease in the relative fluid compartment volumes of TBW and ECF during the first year of life, followed by additional decreases in ECF later in childhood. Table 47-8 illustrates the changes in TBW, ICF, and ECF during maturation.
Volume of Distribution
Drug distribution is best described by the volume of distribution, which is determined by dividing the dose of the administered drug by the resulting plasma concentration. Accordingly, body water composition influences the volume of drug distribution. Infants have a larger extracellular fluid compartment and greater TBW content. Due to a dilutional effect, lower plasma drug concentrations occur immediately after administration of water-soluble drugs. Accordingly, a larger drug loading dose is required to achieve the desired plasma concentration. The fat, muscle, and fluid volume compartments are compared in Table 48-1. Higher plasma concentrations occur for lipid-soluble drugs when administered according to weight due to decreased fat and muscle concentrations as compared with an adult.1
Protein Binding
Alterations in protein binding theoretically affect the availability of the free-drug fraction of highly protein-bound drugs. Reductions in plasma proteins such as albumin and α-1-acid glycoprotein (AAG) increase the free-drug fraction, which may increase the availability of active drug. Total plasma protein is decreased in the infant, reaching equivalent adult concentrations by 5 to 6 months of age. Albumin, the predominant plasma protein, is responsible for the binding of primarily acidic pharmacologic compounds and AAG, and other proteins are responsible for the binding of basic drugs. Both albumin and AAG concentrations are diminished at birth but reach the adult equivalency by 5 to 6 months, and the fully functional binding capacity of albumin approaches adults at about 1 year old. Albumin concentrations may fluctuate, decreasing in chronic disease states with parallel increases in inducible AAG concentration. Protein binding of many anesthetic drugs is decreased relative to adults, but there is little clinical consequence. The major factor affecting drug action is the reduced clearance. A highly protein-bound drug with a high extraction ratio and a narrow therapeutic index, such as lidocaine, requires reduced doses.2 Drug metabolism takes place in the liver, gastrointestinal tract, gastric mucosa, and lungs. In most instances, metabolism reduces drug activity; however, the metabolite may be activated as with a prodrug. The ultimate goal of drug metabolism is the production of a water-soluble compound that can be more easily excreted.
Although the necessary enzyme systems are present at birth, enzyme activity is reduced, thereby increasing drug elimination half-lives. Drugs that produce a prolonged plasma half-life in the pediatric patient include bupivacaine (25 hours),3 mepivacaine (8.5 hours),4 diazepam (up to 100 hours),5 indomethacin (15 to 20 hours),6 meperidine (22 hours), and phenytoin (21 hours).7
Drug Administration
The majority of drugs used in anesthesia are given by the inhalation or intravenous routes. Routine medications are also given by oral and rectal drug administration. Drugs are usually formulated as liquids for oral administration in children. Midazolam may be administered orally for premedication, and the rectal route may be selected for the administration of acetaminophen, opioids, and benzodiazepines.8 Both routes rely on passive diffusion for drug absorption, although the rectal route can be erratic and variable. The resulting plasma drug concentration is dependent on the molecular weight, degree of drug ionization, and lipid solubility.
The degree of ionization of orally administered drugs is dependent on gastric and intestinal pH levels. Acidic drugs are nonionized and are favorably absorbed in an acidic environment such as the stomach. Basic drugs have more favorable absorption in the alkaline medium of the intestine. Most oral medications are absorbed in the intestine. The rate of absorption of most orally administered drugs is slower in neonates and young children because of a delayed gastric emptying time, which prolongs the time to reach the intestines. Gastric emptying time reaches adult values by 6 months of age.9–11
Acetaminophen is a popular antipyretic commonly administered to children during the perioperative period. Acetaminophen is capable of inducing dose-dependent hepatocyte injury in high doses. Acetaminophen is metabolized by the hepatic microsomal enzyme system, and approximately 80% of the parent drug is conjugated with glucuronic acid and sulfate (phase II metabolism). Animal data suggest that a small amount of the parent drug is metabolized by the cytochrome P-450 enzyme system (phase I metabolism), producing an intermediate metabolite that undergoes conjugation with glutathione and is excreted in the urine. High doses of acetaminophen may deplete glutathione, increasing the accumulation of this intermediate metabolite, which is thought to be responsible for acetaminophen-induced liver necrosis. Glutathione depletion may develop with continued administration of high doses of acetaminophen.12 Doses used in anesthesia rarely approach toxic levels.
Intravenous acetaminophen (Ofirmev) is marketed for mild to moderate pain and to reduce fever in adults and children. Given in conjunction with an opioid for moderate to severe pain, it has been shown to have an opioid-sparing effect. The recommended dose of Ofirmev for patients 13 years old or older weighing 50 kg or more is 1000 mg every 6 hours or 650 mg every 4 hours IV; the maximum daily dose of acetaminophen by any route is 4000 mg. For patients 2 years old or older weighing less than 50 kg, the recommended dose is 15 mg/kg every 6 hours or 12.5 mg/kg every 4 hours IV.13
Types of Agents
The rapid increase in alveolar concentration of inspired anesthetic is quantified by the ratio of the alveolar to inspired concentration (FA/FI). Factors that affect the FA/FI ratio include the delivered inspired anesthetic concentration, the inhalation agent blood-gas partition coefficient, alveolar ventilation (VA), cardiac output (Q), and the distribution of Q to the vessel-rich organs (i.e., heart, brain, kidneys, and liver). Although tidal volume is similar between children and adults (5 to 7 mL/kg), children have greater minute ventilation and a higher ratio of tidal volume to functional residual capacity (5:1) compared with the adult (1.5:1). The greater minute ventilation and higher Q in infants and children are responsible for rapid inhalation anesthetic uptake and rapidly increasing alveolar anesthetic concentration. In addition, their decreased distribution of adipose tissue and decreased muscle mass affect the rate of equilibration among the alveoli, blood, and brain. The percentage of blood flow to the vessel-rich organs is greater than in the adult, and the blood-gas partition coefficients are lower in infants and children.14,15
The minimum alveolar concentration (MAC) of inhalation anesthetics is an indicator of dose and anesthetic requirements that change with age. Neonates have a somewhat lower MAC, which peaks at around 30 days of age (Figure 48-1). MAC is higher in infants from age 1 to 6 months of age; thereafter, MAC values are known to decrease with increasing age.16,17 These increased requirements are a reflection of increased basal metabolic rate.
Myocardial depression may be exaggerated when inhalation anesthetics are administered to pediatric patients.18,19 A more rapid rise in FA/FI ratio, the greater percentage of blood flow to the vessel-rich organs, and higher administered anesthetic concentrations are central to the cause of myocardial depression. To summarize, inhalation induction is more rapid in pediatric patients and is accompanied by a higher incidence of myocardial depression than in adults.
Sevoflurane
The MAC of sevoflurane in oxygen is 3% for the infant up to 6 months of age, decreasing to 2.5% to 2.8% up to 1 year of age.20,21 Sevoflurane produces a rapid induction and emergence due to its low blood-gas partition coefficient.22 Sevoflurane is easily breathed and is readily accepted for mask induction. Sevoflurane is the inhalation agent of choice for pediatric anesthesia. Sevoflurane depresses minute ventilation and, at deeper levels, respiratory rate. At high inspired concentrations, apnea will occur. 23,24
Sevoflurane metabolism may produce concentration-dependent elevations in serum fluoride levels that decline when sevoflurane is discontinued.25 Concerns regarding fluoride-induced renal damage have not been a clinical issue. Sevoflurane does not sensitize the myocardium to the effects of endogenous and exogenous catecholamines. As with any inhalation agent, the degree of myocardial depression is dependent on the concentration—higher concentrations cause a greater degree of inhibition of myocardial contractility. The MAC of sevoflurane in oxygen is 2% to 3%.
Isoflurane
The MAC of isoflurane in oxygen is 1.6% in infants and children. Inhalation induction with isoflurane produces more adverse respiratory events (i.e., breath-holding, coughing, and laryngospasm with copious secretions) than sevoflurane. Administration of isoflurane to adults produces dose-dependent decreases in peripheral vascular resistance, whereas increases in heart rate maintain blood pressure. This touted advantage (i.e., increase in heart rate to maintain blood pressure) does not occur in infants. Anesthetic induction in infants with isoflurane produces significant dose-dependent decreases in heart rate, blood pressure, and mean arterial pressure.26
Desflurane
The MAC of desflurane in oxygen is 9% for infants and 6% to 10% for children. Desflurane has the lowest blood-gas partition coefficient of all the inhalation anesthetics (0.42), which facilitates a rapid induction, rapid alterations in anesthetic depth, and emergence. Like isoflurane, desflurane is pungent and is associated with more adverse respiratory events during inhalation induction including breath-holding, laryngospasm, coughing, and increased secretions with accompanying hypoxia. After inhalation induction with sevoflurane, desflurane is appropriate for the maintenance of general anesthesia with facemask, endotracheal tube, or laryngeal mask airway. As in the adult population, dramatic increases in desflurane concentrations may induce sympathetic stimulation evidenced by tachycardia and hypertension.27,28
Emergence Delirium
A variety of terms are used interchangeably when referring to postoperative agitation. These include emergence delirium, emergence agitation, and postanesthetic agitation. These terms describe altered behavior in the immediate postoperative period manifested as nonpurposeful restlessness, crying, moaning, incoherence, and disorientation.29,30 These episodes can be very upsetting to the parents and caregivers. Emergence delirium is fortunately self-limiting but may manifest for as long as 45 minutes. The incidence ranges from 10% to 80%. It occurs during the early stage of emergence during initial wakening. No single factor has been identified as the cause of emergence agitation, which is a composite of biologic, pharmacologic, psychological, and social components.31 Recent hypotheses have implicated the rapid emergence associated with the newer low-solubility anesthetic agents such as sevoflurane and desflurane. This may create a dissociative state in which children awaken with altered cognitive perception, although others dispute the speed of emergence as a factor. There is conflicting research as to the incidence with the slower emergence of isoflurane.32,33 Proposed causes also include rapid awakening in unfamiliar settings, pain, stress during induction, hypoxia, airway obstruction, noisy environment, anesthesia duration, child’s personality, premedication, and type of anesthesia. A recent meta-analysis found that propofol, ketamine, fentanyl, dexmedetomidine, and preoperative analgesia had a prophylactic effect in preventing agitation and treating acute episodes. Midazolam and 5HT3 inhibitors do not have a protective effect.34
Intravenous Anesthetics
As discussed previously, infants and children have a higher proportion of cardiac output delivered to vascular-rich tissues (i.e., heart, brain, kidneys, and liver). Intravenously administered drugs are readily taken up by these tissues and are subsequently redistributed to muscle and fat, tissues that are less well perfused. Intravenously administered drugs may have a prolonged duration of action in infants and children because of decreased percentages of muscle and fat. The central nervous system (CNS) effects of opioids also may be prolonged because of the immaturity of the blood-brain barrier.35,36 Although this evidence suggests that intravenously administered anesthetic doses should be reduced, one must also recall the effect of increased body water. Increased doses of propofol and ketamine are required, presumably because of a greater volume of distribution.37
Propofol
Propofol has a rapid onset and a short duration of action and is the most popular agent for induction and maintenance of general anesthesia. It also may be combined with an opioid and nitrous oxide to provide total intravenous anesthesia. Propofol may be delivered as a continuous infusion for short diagnostic and radiologic procedures and is used as a primary sedative in chronically ventilated intensive care patients. Its antiemetic properties may reduce the incidence of postoperative nausea and vomiting in children undergoing strabismus correction.38 Infants require larger induction doses (2.5 to 3 mg/kg) than children (2 to 2.5 mg/kg).39,40 These induction doses produce decreased systolic blood pressure.41,42 The pain that accompanies intravenous administration may be reduced with the addition of small amounts of lidocaine. Additional strategies used to decrease the pain during injection include a slower injection of propofol into a rapid-running intravenous line or injection into larger intravenous catheters placed in the antecubital space.43
In 1992, Parke et al. reported the deaths of five children who received long-term, high-dose propofol infusions.44 The large doses were administered by continuous infusion in critical care units with long-term sedation over several days and were not associated with short-term anesthesia use. Additional case reports followed, further characterizing this serious reaction, subsequently referred to as propofol infusion syndrome. Propofol infusion syndrome is characterized by severe lactic acidosis, followed by rhabdomyolysis and lipidemia. It frequently results in cardiovascular collapse and death. The likely mechanism involves propofol inhibiting mitochondrial function and uncoupling oxidative phosphorylation (see Chapter 9). Children with mitochondrial defects may have an increased risk with propofol administration.45,46 Algorithms have been proposed to prevent this adverse drug effect.47
Neuromuscular Blocking Agents
Neuromuscular blocking drugs are highly ionized and have a low lipophilicity, restricting their distribution to the ECF compartment. The ECF compartment is larger in the neonate and infant than in the child and adult, causing a dilutional effect and thereby increasing the volume of distribution. Increases in ECF volume and the ongoing maturation of neonatal skeletal muscle and acetylcholine receptors affect the pharmacokinetics and pharmacodynamics of neuromuscular relaxants. Table 47-9 references the effective doses of neuromuscular blocking drugs in various age groups.
The neuromuscular junction is incompletely developed at birth, maturing after 2 months of age.48 Skeletal muscle, acetylcholine receptors, and the accompanying biochemical processes essential in neuromuscular transmission mature during infancy into childhood.
The presynaptic release of acetylcholine is slowed compared with the adult, which explains the decreased margin of safety for neuromuscular transmission in the neonate. The acetylcholine receptors of the newborn are anatomically different from the adult receptors, which may explain the sensitivity of the neonate to the nondepolarizing class of neuromuscular relaxants.49 This neuromuscular immaturity may be demonstrated with the appearance of fade after tetanic stimulation in the absence of neuromuscular blocking drugs.48,50
Succinylcholine
In the early 1990s a series of unexpected cardiac arrests were reported after the routine administration of succinylcholine in apparently healthy children, with less than 40% of patients successfully resuscitated. After much research it was found that the patients had undiagnosed Duchenne muscular dystrophy. Succinylcholine administration produces massive hyperkalemia and subsequent cardiac arrest in these children.51 The U.S. Food and Drug Administration (FDA) relabeled succinylcholine, restricting its use to emergency airway management in children.52,53 It is now used only as a rescue drug in children younger than 8 years old. Most clinicians avoid using it for patients through the early teen years and in patients with muscle disorders. It is contraindicated in patients with malignant hyperthermia.54,55
Nondepolarizing Neuromuscular Blocking Agents
Infants and children are more sensitive than adults to the effects of nondepolarizing neuromuscular blocking drugs (see Table 47-9). A lower plasma concentration of the selected neuromuscular relaxant is required to achieve the desired clinical level of neuromuscular blockade. This does not imply that the selected dosage should be decreased because infants have a greater volume of distribution. The larger volume of distribution and slower drug clearance result in longer elimination half-life, decreasing the need for repeated drug dosing (longer dosing intervals). Neuromuscular function monitoring must be used to guide repeated administration of these drugs in all pediatric patients. The degree of neuromuscular blockade should be assessed using physical signs (e.g., strength of movement, spontaneous respirations with adequate tidal volume) and neuromuscular monitoring (i.e., train-of-four and sustained tetanus). Checking neuromuscular function should be accomplished by assessing the adductor pollicis muscle on the forearm because function in this region is more reflective of diaphragmatic activity as compared with the orbicularis oculi (temporal region). The selection of a nondepolarizing neuromuscular relaxant should take into consideration the desired degree and duration of skeletal muscle paralysis, the immaturity of organ systems, and the associated side effects of the selected relaxant.56,57
Antagonism of Neuromuscular Blockade: Residual neuromuscular blockade places the infant and child at risk of hypoventilation and the inability to independently and continuously maintain a patent airway. Because of increased basal oxygen consumption, impaired respiratory function will lead to arterial oxygen desaturation and CO2 retention. The detection of residual neuromuscular blockade requires the integration of clinical criteria and the assessment of neuromuscular blockade via a peripheral nerve stimulator. Conventional doses of the anticholinesterase inhibitors (50 to 60 mcg/kg of neostigmine; 500 to 1000 mcg/kg of edrophonium) combined with appropriate doses of atropine or glycopyrrolate are acceptable for antagonism of nondepolarizing neuromuscular blockade.
Voluntary clinical tests are obviously not applicable to the infant and young child. Useful clinical signs of successful antagonism of neuromuscular blockade include the ability to flex the arms, lift the legs, and flex the thighs upon the abdomen, providing evidence of the return of abdominal muscle tone, in addition to the return of a normal responses to nerve stimulation.58 Neonates are capable of generating a negative inspiratory force (NIF) of −70 cm H2O with the first few breaths after birth.59 A negative inspiratory force of at least −32 cm H2O has been found to correspond with leg lift, which is indicative of the adequacy of ventilatory reserve required before tracheal extubation.60,61
Preoperative Preparation
Review of Systems
Reviewing the medical record can answer many questions before approaching the patient/parents for information. Understanding congenital history can help preparation and development of a plan of care. Previous anesthetic records can be useful tools. Table 48-2 lists the anesthetic implications of the review of systems and history.
TABLE 48-2
Medical History and Review of Symptoms: Anesthetic Implications
System | History | Possible Anesthetic Implications |
Central nervous and neuromuscular | Seizures | Medications: Drug interactions, possible inadequate serum levels, valproate-induced hepatitis |
Head trauma | Elevated intracranial pressure | |
Hydrocephalus | Possible elevated intracranial pressure | |
CNS tumor | Possible elevated intracranial pressure, chemotherapeutic drugs and interactions | |
Possible risk of malignant hypothermia | ||
Cardiovascular | Heart murmur | Septal defect, avoid air bubbles in IV line |
Cyanosis | Right-to-left cardiac shunt | |
History of squatting | Possible tetralogy of Fallot | |
Diaphoresis with feedings | Congestive heart failure | |
Hypertension | Possible coarctation of the aorta; renal disease; pheochromocytoma | |
Transplant recipient | Fixed heart rate; insensitivity to anticholinergic drugs | |
Respiratory | Prematurity | Increased risk of postoperative apnea; possible lower respiratory tract illness |
Bronchopulmonary dysplasia | Lower airway obstruction; reactive airways; possible subglottic stenosis; possible postoperative hypoxia and apnea; pulmonary hypertension | |
Lower respiratory infection, cough | Reactive airways; bronchospasm; medication history; drug interactions | |
Croup | Possible subglottic stenosis or anomaly | |
Snoring, sleep apnea | Perioperative airway obstruction; hypoxia | |
Asthma | β-Agonist or theophylline drugs; pulmonary hypertension or cor pulmonale; steroid use; adrenal insufficiency; postoperative hypoxia | |
Cystic fibrosis | Drug interactions; pulmonary toilet; pulmonary dysfunction; reactive airways | |
Recent cold | Possible lower respiratory tract infection; reactive airways | |
Gastrointestinal and hepatic | Vomiting, diarrhea | Electrolyte abnormality; dehydration; full stomach |
Growth failure | Possible anemia | |
Gastroesophageal reflux | Risk of aspiration; reactive airways; hypoxia | |
Jaundice | Altered drug metabolism; risk of hypoglycemia | |
Liver transplant recipient | Altered drug metabolism; immunosuppression | |
Frequency, nocturia | Unrecognized diabetes, urinary tract infection | |
Renal failure or dialysis | Electrolyte abnormality; hypervolemia or hypovolemia; anemia; medication history | |
Renal transplant recipient | Immunosuppression | |
Endocrine and metabolic | Hypoglycemia | Hypoglycemia |
Diabetes | Insulin requirement; intraoperative hypoglycemia | |
Steroid therapy | Adrenal insufficiency | |
Pregnancy | Teratogenic effects of N2O and other drugs; risk of spontaneous abortion | |
Hematologic | Anemia | Transfusion requirement |
Bruising, excessive bleeding | Coagulopathy | |
Sickle cell disease | Anemia; transfusion; hydration; oxygenation; orthopedic tourniquet use | |
AIDS | Susceptibility to infection; infectious risk to medical personnel | |
Allergies | Medication history | Drug reactions; drug interactions |
Dental | Loose teeth | Dental trauma; aspiration of tooth |
Modified from Coté CJ, et al, eds. A Practice of Anesthesia for Infants and Children. 5th ed. Philadelphia: Saunders; 2013.
• Birth and development. Was the child born prematurely or term? Did the child experience any neonatal complications or spend any time in the neonatal intensive care unit? Does the child have any history of apnea or bradycardia? Is there any history of sudden infant death syndrome in the child’s family? Are there any developmental or learning setbacks?
• Heart disease. Does the patient play at school without severe shortness of breath or syncope? Does the child have a heart murmur?
• Pulmonary problems. Does the child have asthma, bronchitis, or pneumonia? Does the child currently receive, or has received in the past, supplemental oxygen therapy? Has the child had a recent cold, cough, or respiratory infection? Many times the child will have a persistent runny nose due to an inner ear infection. It is important to ask the parent about the color, consistency, and duration of drainage.
• Bleeding disorders. Does the child have sickle cell anemia? Does the child bleed or bruise easily?
• Liver disease. Has the child had jaundice or liver problems?
• Urology problems. Has the child had problems with the kidneys, ureters, or bladder? Any urinary tract infections?
• Gastrointestinal problems. Has the child had gastric acid reflux or a hiatal hernia? Has the child experienced problems with diarrhea or vomiting?
• Neurologic problems. Has the child experienced any neurologic symptoms such as seizures?
• Obstetric history. Could the patient possibly be pregnant? (controversial topic)
• Airway. Does the patient have a history of a difficult intubation? Does the child have trouble opening the mouth? Are there any chipped, missing, or loose teeth?
• Other concerns. Does the child have diabetes or hypoglycemia problems? Does the child have any problems with the thyroid or adrenal glands?
• Medications. Is the child currently on any medications or has he or she taken any within the last 3 months? Has the child taken steroids within the last year? If so, for what condition?
• Allergies. Is the patient allergic to any medication or food? Any problems with latex or other environmental items?
Fasting Status
The risk of pulmonary aspiration in the pediatric patient is extremely low (1 in 10,000).62 Accordingly, traditional fasting guidelines have become broader. Although the goal of preoperative fasting is to ensure an empty stomach at the time of anesthetic induction, prolonged fasting may produce irritability as a result of thirst and hunger. Prolonged fasting also may alter fluid balance, producing preinduction hypovolemia and hypoglycemia. Hypoglycemia is especially problematic in premature infants. Preoperative access to clear fluids (e.g., apple juice, water) 2 hours before anesthetic induction has been shown to have a minimal impact on gastric volume. Current recommendations by the American Society of Anesthesiologists (ASA) are 2 hours of fasting for clear liquids, 4 hours for breast milk, 6 hours for infant formula and non-human milk and a light meal, and 8 hours for a regular meal including fatty foods (see Table 43-2). The guidelines are commonly referred to as the 2-4-6-8 rule.63 These parameters are suggested for healthy patients undergoing elective surgery. No gum is to be chewed after midnight; also, prescribed medications can be taken with a sip of water or prescribed liquid mixture. If a patient should present with a history of hiatal hernia while preparing for elective surgery and has not been nil per os (NPO; nothing by mouth) for the recommended period of time, providers must collaborate to make the best decision in regard to keeping the patient safe. For a patient such as this, the best decision will likely be to postpone surgery until the proper fasting time has been achieved.
Upper Respiratory Infection
Upper respiratory infections (URIs) are common in the pediatric age group, are seasonal in occurrence, and may be accompanied by cough, pharyngitis, tonsillitis, and croup. The child with an active or resolving URI has increased airway reactivity, a propensity for the development of atelectasis and mucous plugging of the airways, and the potential to experience postoperative arterial hypoxemia.64 In addition, bronchial reactivity may persist for 6 to 8 weeks after a viral lower respiratory tract infection. The presence of chronic respiratory disease (asthma or bronchopulmonary dysplasia) requires a thorough assessment and medication review to ensure that the disease is well controlled and the child is not currently experiencing an exacerbation. A history of steroid use necessitates consideration of steroid supplementation throughout the perioperative period.
Lower respiratory tract dysfunction typically accompanies viral or bacterial URI. This combination may be associated with a greater frequency of laryngospasm (fivefold greater incidence) and bronchospasm (tenfold greater incidence) during anesthetic management, particularly when endotracheal intubation is performed.65 Although mild URI may be inconsequential during the intraoperative period, significant problems may develop in the immediate postoperative period. Studies have noted an increase in the incidence of postintubation croup, hypoxemia, and bronchospasm in patients with URIs compared with asymptomatic children.65–67
Multiple factors must be considered when one is deciding whether to cancel an elective procedure. Children with signs and symptoms of acute airway dysfunction should have further medical evaluation by a pediatrician. A white blood cell count of 12,000 to 15,000/mm3 suggests the presence of infection, and the surgery should be canceled. Clearly, elective surgery should be postponed for children who have a cough and pharyngitis accompanied by fever and wheezing. Acute verses chronic symptoms need to be deciphered. Children with a fever 38.4° C and higher, malaise, wheezing, dyspnea, rhonchi, nasal congestion, and a productive cough are showing signs of an acute infection necessitating the postponement of surgery.68,69 Evidence-based recommendations are given in Box 48-1.
Laboratory Testing
Laboratory testing should be ordered preoperatively based upon the patient’s medical history and physical examination. Indications for preoperative laboratory testing are listed in Box 48-2. The American Academy of Pediatrics (AAP) states routine laboratory tests do not need to be performed in healthy patients undergoing outpatient surgery.70 However, in patients presenting with an abnormality, preoperative testing is imperative to guide the surgical and anesthetic plans.
Preoperative laboratory tests should be ordered based on abnormal findings from the medical history and physical examination. Preoperative hemoglobin determination has characteristically been obtained to provide an assessment of anesthetic fitness. An ‘‘adequate’’ hemoglobin concentration is essential for oxygen delivery and has been arbitrarily defined as a hemoglobin of 10 g/dL or a hematocrit of 30%. No scientific studies support or refute this ‘‘acceptable’’ quantity. The determination of an acceptable value requires an understanding of the child’s current medical history, the proposed surgical procedure, and an understanding of global oxygen transport and use. The value of a routine hemoglobin determination has been questioned for some time and has been found rarely to affect the anesthetic management of children.71 The frequency of asymptomatic anemia in a prospective study of 2649 pediatric outpatients was found to be less than 1%, with 7 of 14 anemic patients younger than 1 year of age. However, the frequency of anemia may be as high as 12% in immigrant and indigent children.72 Children who benefit from preoperative hemoglobin determinations include premature infants less than 60 weeks’ postconceptional age, children with concurrent cardiopulmonary disease, children with known hematologic dysfunction (e.g., sickle cell disease), and children in whom major blood loss is anticipated during the surgical procedure.
Premedication
The selection and administration of premedication for the pediatric patient requires an understanding of the desired goals, the planned surgical procedure (inpatient or outpatient procedure), the familiarity and previous experiences with the particular drug, and the availability of nursing staff to monitor the child after the drug’s administration (Box 48-3). The ideal premedicant should be dependable, with a rapid and reliable onset and offset, and should be devoid of undesirable effects. The use of ‘‘cookbook’’ doses of premedicants is hazardous and may produce general anesthesia in some children while producing ineffective sedation in others.73 Table 48-3 lists commonly prescribed pediatric premedicants. Premedication must be individualized to account for differences in maturation and development and the child’s previous surgical experiences. The reliance on pharmacologic premedication in preparing the child for the surgical experience should not be routine; rather, it should be reserved for children who are extremely apprehensive. Anxiolytics and sedatives may prolong the time to discharge, thereby increasing patient care costs.74 Older children may benefit from anxiolytic premedication to decrease preoperative anxiety and modify behavioral changes after discharge. Analgesics are important for children experiencing pain and to provide some postoperative relief.75

Full access? Get Clinical Tree
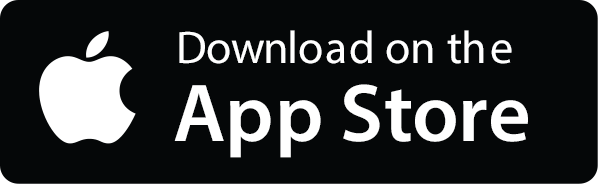
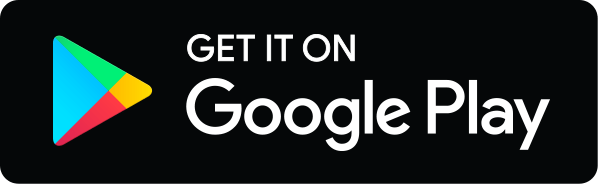