PULSE OXIMETRY
The amount of oxygen reversibly bound to hemoglobin in arterial blood is defined as hemoglobin saturation (SaO2), a critical element of systemic oxygen delivery. Unfortunately, clinical detection of hypoxemia is unreliable. Pulse oximeters enable in vivo, noninvasive, and continuous measurement of arterial oxygen saturation at the bedside. Reliable interpretation of the information provided by these devices requires appreciation of their technology and limitations.
Principles of Measurement
Pulse oximetry relies on the principle of spectral analysis, which is the method of analyzing physiochemical properties of matter based on their unique light-absorption characteristics. For blood, absorbance of transmitted light is dependent on the concentration of hemoglobin species. Oxygenated hemoglobin absorbs more infrared light and allows more red light to pass through than deoxygenated hemoglobin.
Oximeters are made up of a light source, photodetector, and microprocessor. Light-emitting diodes (LED) emit high-frequency signals at 660 nm (red) and 940 nm (infrared) wavelengths. When positioned to traverse or reflect from a cutaneous vascular bed, the opposed photodetector measures the light intensity of each transmitted signal. Signal processing exploits the pulsatile nature of arterial blood to isolate arterial saturation. The microprocessor averages these data over several pulse cycles and compares the measured absorption to a reference standard curve to determine hemoglobin saturation, which is displayed as percentage of oxyhemoglobin (SpO2). SpO2 and SaO2 correlation vary with manufacturer but exhibit high accuracy (±2%) within normal physiologic range and circumstances.
Anatomical locations with high vascular density are preferred for probe placement, and two oximetry techniques are used in clinical practice. Transmission oximetry deploys the LED and photodetector on opposite sides of a tissue bed (e.g., digit, nose, or ear lobe) such that the signal must traverse tissue. Reflectance oximeters position the LED and photodetector side by side on a single surface and can be placed in anatomical locations without an interposed vascular bed (e.g., forehead). This facilitates more proximal sensor placement with improved response time relative to core body SaO2.
Indications
Pulse oximetry provides important, real-time physiologic data and is the standard noninvasive measure of arterial saturation. It is now widely considered one of the standard measurements in patient vital signs. Continuous monitoring is indicated for any patient at risk or in the midst of acute cardiopulmonary decompensation. Reliable continuous oximetry is mandatory for patients requiring airway management and should be a component of each preintubation checklist. If placed on an extremity, the probe is preferably placed on the opposite side of the blood pressure cuff to avoid interruptions in SaO2 readings during cuff inflation.
Limitations and Precautions
Pulse oximeters have a number of important physiologic and technical limitations that influence bedside use and interpretation (Table 8-1).
Signal Reliability
Proper pulse oximetry requires pulse detection to distinguish light absorption from arterial blood relative to the background of other tissues. Abnormal peripheral circulation as a consequence of shock, vasoconstriction, or hypothermia may prevent pulsatile flow detection. Heart rate and plethysmographic waveform display verify arterial sensing, and SpO2 should be considered inaccurate unless corroborated by these markers. Varying pulse amplitude is easily recognized on the monitor and represents the measure of arterial pulsatility at the sampled vascular bed. Quantification in the form of perfusion index is being incorporated into some software to verify signal reliability and gauge microvascular flow.
Etiology and Examples of Unreliable Pulse Oximetry |
Etiology | Examples |
Sensor location | Critical illness (forehead probe is best) |
Extraneous light exposure | |
Motion artifact | Exercise |
Cardiopulmonary resuscitation (CPR) | |
Seizure | |
Shivering/tremor | |
Prehospital transport | |
Signal degradation | Hypothermia |
Hypotension/shock | |
Hypoperfusion | |
Vasoconstriction | |
Nail polish/synthetic nails | |
Physiologic range | Increasingly inaccurate when systolic BP < 80 mm Hg |
Increasingly inaccurate when SaO2 < 75% | |
Severe anemia | |
Sickle cell anemia | |
Dyshemoglobinemia | CO-Hgb (overestimates SpO2) |
Met-Hgb (variable response) | |
Intravenous dye | Methylene blue |
Indocyanine green |
Even with verified signal detection, measurement bias limits SpO2 reliability during physiologic extremes. Reliability deteriorates with progressive hypotension below systolic BP of 80 mm Hg, and in these patients, readings generally underestimate true SaO2. Severe hypoxemia with SaO2 < 75% is also associated with increased measurement error as comparisons to reference standards are limited below this value. However, patients with this severity of hypoxemia are typically receiving maximized intervention, and closer discrimination in this range rarely imparts new information that alters management.
A number of physical factors affect pulse oximetry accuracy. Signal reliability is influenced by sensor exposure to extraneous light, excessive movement, synthetic fingernails, nail polish, intravenous dyes, severe anemia, and abnormal hemoglobin species. Diligent probe placement and shielding the probe from extraneous light should be routine. Surface extremity warming may improve local perfusion to enable arterial pulse sensing, but SpO2 accuracy using this technique is not confirmed.
Dyshemoglobinemias such as carboxyhemoglobin (CO-Hgb) and methemoglobin (Met-Hgb) absorb light at different wavelengths and may affect the accuracy of oximetry readings. Co-oximeters (and some new generation pulse oximeters) use four wavelengths of light stimulus to selectively discriminate these species. However, CO-Hgb absorbance is close to oxyhemoglobin such that most conventional pulse oximeters sum up their measurement and give artifactually high SpO2 readings. Met-Hgb produces variable error, depending on the true oxy- and Met-Hgb levels. SpO2 classically approximates 85% in severe toxicity.
Response Time
Pulse oximetry readings lag the patient’s physiologic state; signal averaging of 4 to 20 seconds is typical of most monitors. Delay because of sensor anatomical location and abnormal cardiac performance compound the lag relative to central SaO2. Forehead and ear probes are closer to the heart and respond more quickly than distal extremity probes. Response difference compared to central SaO2 is also compounded by hypoxemia (i.e., starting on the steep portion of the oxyhemoglobin dissociation curve) and slower peripheral circulation such as low cardiac output states. As such, forehead reflectance probes are often preferred in critically ill patients. All of these response delays become more clinically important during rapid desaturation such as that which may occur during airway management and is the basis for our general recommendation to abort most intubation attempts when the SpO2 falls below 93%.
Physiologic Insight and Limitations
Hemoglobin saturation is just one part of the assessment of systemic oxygenation. Although monitoring is continuous, SpO2 provides momentary information on arterial saturation without true insight into systemic oxygenation and respiratory reserve. The physiologic context of oximetry is critical for appropriate interpretation and assists estimation of a patient’s cardiopulmonary reserve for planning and execution of an airway management plan.
Oximetry measures arterial hemoglobin saturation but not the arterial oxygen tension or oxygen content of blood. The oxyhemoglobin dissociation curve (see Chapters 5 and 20) describes the relationship of oxygen partial pressure (PaO2) and saturation (SaO2). Its sigmoidal shape hinges on varying hemoglobin affinity with successive oxygen binding. It is important to note that SpO2 provides poor correlation with PaO2 in the normal range. Normal SaO2 is associated with a wide range of PaO2 (80 to 400 mm Hg), which includes two extremes of oxygen reserve. Similarly, oximetry is insensitive at detecting progressive hypoxemia in patients with high-baseline PaO2. Correlation is established in the hypoxemic range at and below the upper inflection point of the oxyhemoglobin curve (PaO2 < 60 mm Hg approximating SaO2 90% at normal pH) where desaturation is rapid with declining PaO2.
Hemoglobin saturation must also be interpreted in the context of inspired oxygen fraction (FIO2) to provide insight into gas exchange and physiologic reserve. Simple observation at the bedside provides qualitative assessment. More formal calculation of the SpO2

Full access? Get Clinical Tree
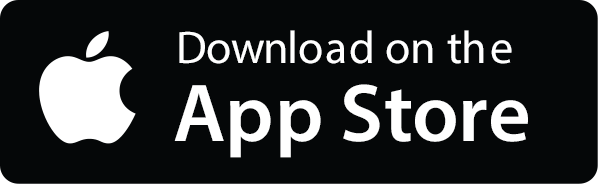
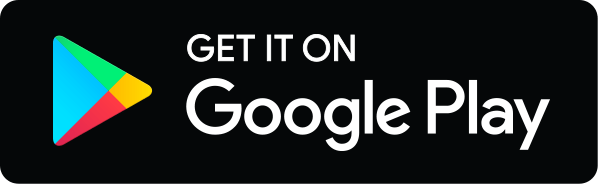