Osmotic Disorders
As many as 40% of ICU patients have a problem with the osmotic balance between intracellular and extracellular fluid (1). The signal for this problem is a change in the plasma sodium concentration, but the real problem is a change in cell volume, which is most apparent in the central nervous system. This chapter presents a simple approach to osmotic disorders based on a single variable: the extracellular volume.
I. Osmotic Activity
The concentration of solutes in a solution can be expressed in terms of osmotic activity, which is a reflection of the number of solute particles in a solution. The unit of measurement is the osmole (osm), which is 6 * 1023 particles (Avogadro’s Number) for a non-dissociable substance (2). The osmotic activity in a fluid compartment determines its water content.
A. Relative Osmotic Activity
When two fluid compartments are separated by a semipermeable membrane (not freely permeable to solutes), the solutes will not distribute evenly in the fluid compartments, and the osmotic activity will be higher in one fluid compartment. Water then moves from the fluid with the lower osmotic activity into the fluid with the higher osmotic activity.
The difference in osmotic activity between the fluid compartments is called the effective osmotic activity, and it is the force that drives the movement of water between fluid compartments. This force is also called the osmotic pressure.
The fluid with the higher osmotic activity is described as hypertonic, and the fluid with the lower osmotic activity is described as hypotonic.
If the two fluid compartments are the intracellular and extracellular volumes, then:
When the extracellular fluid is hypertonic, water will move out of cells.
When the extracellular fluid is hypotonic, water will move into cells.
B. Units of Osmotic Activity
Osmotic activity can be expressed in relation to the volume of the solution, or the volume of water in the solution (3,4).
Osmotic activity per volume of solution is called osmolarity, and is expressed as milliosmoles per liter (mosm/L).
Osmotic activity per volume of water is called osmolality, and is expressed as milliosmoles per kilogram of H2O (mosm/kg H2O, or mosm/kg).
Plasma is mostly (95%) water, so osmolality is typically used to described the osmotic activity of plasma. However, there is little difference between the osmolality and osmolarity of plasma, and the two terms are often used interchangeably (4).
C. Plasma Osmolality
The osmotic activity of plasma can be measured or calculated.
1. Measured Plasma Osmolality
Plasma osmolality is measured with the freezing point depression method. The freezing point of water (0° C) decreases 1.86° C for each osmole of solute that is added to one kilogram (liter) of water. Therefore, the freezing point of an aqueous solution (relative to water) can be used to determine the osmotic activity of the solution. This is the “gold-standard” method for measuring plasma osmolality.
2. Calculated Plasma Osmolality
Plasma osmolality can be calculated using the concentrations of the principal solutes in plasma (sodium, chloride, glucose, and urea) (3); i.e.,

Posm is the plasma osmolality in mosm/kg H2O (or mosm/kg).
Plasma Na is the sodium concentration in mEq/L, and is doubled to include the osmotic activity of chloride.
Glucose and BUN are the plasma concentrations of each in mg/dL.
18 and 2.8 are the molecular weights of glucose and urea divided by 10 (to express their concentrations in mosm/kg).
EXAMPLE: Using normal plasma concentrations of Na (140 mEq/L), glucose (90 mg/dL), and BUN (14 mg/dL), the plasma osmolality is: (2 * 140) + 90/18 + 14/2.8 = 290 mosm/kg.
3. Effective Plasma Osmolality
Urea readily crosses cell membranes, so an increase in
BUN will not increase the effective osmotic activity of plasma (i.e., azotemia is a hyperosmotic, but not a hypertonic, condition). Therefore, the calculation of effective plasma osmolality does not include the BUN; i.e.,
BUN will not increase the effective osmotic activity of plasma (i.e., azotemia is a hyperosmotic, but not a hypertonic, condition). Therefore, the calculation of effective plasma osmolality does not include the BUN; i.e.,

EXAMPLE: Using normal plasma concentrations of Na (140 mEq/L) and glucose (90 mg/dL), the effective plasma osmolality is: (2 * 140) + 90/18 = 285 mosm/kg.
Note that the plasma sodium accounts for 98% (280 of 285 mosm/kg) of the effective osmotic activity of the extracellular fluid. In other words, the plasma sodium concentration is the principal determinant of the distribution of total body water in the intracellular and extracellular fluid compartments.
D. Osmolal Gap
Because solutes other than sodium, chloride, glucose, and urea are present in the extracellular fluid, the measured plasma osmolality will be greater than the calculated osmolality. This osmolal gap is normally ≤10 mosm/kg (3,5).
The presence of a toxin or drug will increase the osmolal gap, and this makes the osmolal gap a useful measurement in cases of suspected toxin exposure or drug overdose (6).
II. Hypernatremia
The normal plasma sodium is 135–145 mEq/L, so hypernatremia is defined as a plasma sodium >145 mEq/L.
A. Extracellular Volume
Three conditions can produce hypernatremia (7):
Loss of sodium and water, with water loss >sodium loss (i.e., hypotonic fluid loss). This results in a decreased extracellular volume (ECV).
Loss of water only (free water loss), which results in an unchanged ECV.
Gain of sodium and water, with sodium gain >water gain (i.e., gain of hypertonic fluid), which results in an increased ECV.
Since each of these conditions is associated with a different ECV, assessment of the ECV can be used to identify the condition responsible for hypernatremia. This is shown in Figure 27.1. (Assessment of the ECV is not presented here. See Chapter 7 for the evaluation of hypovolemia.)
B. Encephalopathy
The clinical consequences of hypernatremia (i.e., hypertonicity) include insulin resistance, cardiac dysfunction, and encephalo-pathy (1), with the latter condition being the most significant.
Encephalopathy is more likely to occur with a rapid rise in plasma sodium (8). Possible mechanisms include shrinkage of neuronal cell bodies (8) and osmotic demyelination (9).
The clinical manifestations range from agitation and lethargy to coma and generalized or focal seizures (1).
Encephalopathy is a poor prognostic sign in hypernatremia, and carries a mortality rate as high as 50% (9).
III. Hypovolemic Hypernatremia
Hypernatremia associated with a low ECV is the result of loss of hypotonic fluids (i.e., fluids with a sodium concentration <135 mEq/L). Common sources of hypotonic fluid loss include: (a) diuretic-induced urine loss, (b) osmotic diuresis from glycosuria, (c) vomiting and diarrhea, (d) excessive sweat loss in heat-related illnesses, and (e) normal fluid losses without sodium or water intake (e.g., in elderly, debilitated patients).
A. Management
Management is aimed at correcting the two consequences of hypotonic fluid loss: (a) loss of sodium, which reduces the
ECV, and (b) loss of water in excess of sodium (the free water deficit), which creates a hypertonic plasma.
ECV, and (b) loss of water in excess of sodium (the free water deficit), which creates a hypertonic plasma.
1. Sodium Replacement
The most immediate concern with sodium loss is a decrease in plasma volume, which can decrease cardiac output and impair tissue perfusion. Therefore, the management of hypovolemic hyponatremia begins with sodium replacement using isotonic saline.
2. Free Water Replacement
When hypovolemia has been corrected, the next step is to replace the free water deficit. The free water deficit can be estimated, based on the assumption that the product of total body water (TBW) and plasma sodium concentration (PNa) is always constant (7).

Substituting 140 mEq/L for a normal PNa and rearranging terms yields the following relationship:

The normal TBW (in liters) is usually 60% of lean body weight (in kg) in men and 50% of lean body weight in women, but a 10% reduction in the normal TBW has been suggested for hypernatremic patients who are water depleted (10).
For patients who are hyperglycemic, the plasma sodium should be corrected for the dilutional effect of hyperglycemia. This effect averages 2 mEq/L for every 100 mg/dL increase in plasma glucose (see Section V-A-2).
Once the current TBW is calculated, the free water deficit is calculated as:
EXAMPLE: For an adult male with a lean body weight of 70 kg and a plasma Na of 160 mEq/L, the normal TBW is 0.6 * 70 = 42 L, the current TBW is 42 * (140/160) = 36.8 L, and the free water deficit is (42 − 36.8 = 5.2 liters.
Free water deficits are corrected with sodium-containing fluids such as 0.45% NaCL to replace ongoing sodium losses. The volume needed to correct the free water deficit can be estimated as follows (11):
where 140 is the desired plasma Na concentration, and 77 is the Na concentration in 0.45% NaCL.
3. Rate of Change in Plasma Sodium
Neuronal cells initially shrink in response to hypertonic extracellular fluid, but cell volume is restored within hours; an effect attributed to the generation of osmotically active substances within brain cells, called idiogenic osmoles (7). Once the cell volume is restored to normal, aggressive replacement of free water deficits can produce cell swelling and cerebral edema.
IV. Hypernatremia Without Hypo-Volemia
Hypernatremia with a normal ECV is the result of free water loss with no net sodium loss. This condition is common in ICU patients with hypernatremia (1), and usually occurs when sodium losses are replaced, leaving a net free water deficit. The condition described next is probably the best example of a pure water deficit.

Full access? Get Clinical Tree
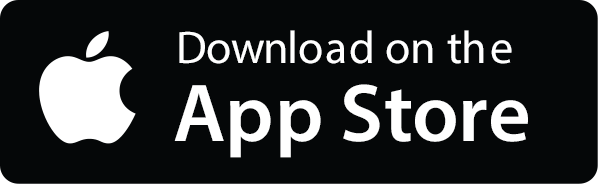
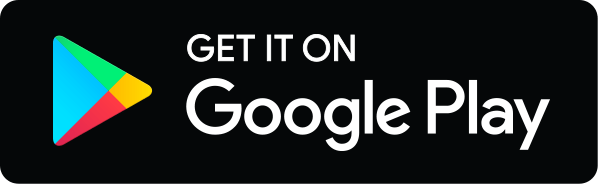