Fig. 19.1
Open fracture of the right lower limb following a motorcycle accident showing extensive loss of soft tissue injury, periosteal stripping an major wound contamination (Type IIIb following the Gustilo classification [13])
Degree | Characteristics |
---|---|
Type I | Wound less than one centimeter long Clean |
Type II | Laceration more than one centimeter long Without extensive soft-tissue damage, flaps, or avulsions |
Type III | Open segmental fracture or Open fracture with extensive soft-tissue damage or Traumatic amputation Special categories: Gunshot injuries Any open fracture caused by a farm injury Any open fracture with accompanying vascular injury requiring repair |
Type IIIa | Open fractures with adequate soft tissue coverage of a fractured bone despite Extensive soft tissue laceration or flaps, or high-energy trauma regardless of the size of the wound |
Type IIIb | Extensive soft tissue injury with periosteal stripping and bone exposure Usually associated with massive contamination |
Type IIIc | Arterial injury requiring repair |
In 2010, a new system was introduced by the Orthopedic Trauma Association based on an extensive literature search as well as weighting by an expert panel. The Orthopedic Trauma Association Open Fracture Classification (OTA-OFC) considers skin injury, muscle injury, arterial injury, contamination and bone loss, and each category is subdivided into three grades of severity (Table 19.2) [16]. This new grading system showed moderate to excellent results regarding the interobserver reliability in a first evaluation [17] and therefore may serve as a new classification system in future investigations. Moreover, the OTA-OFC showed a predictive value regarding treatment strategies [18].
Parameter | Skin | Muscle | Arterial | Contamination | Bone loss |
---|---|---|---|---|---|
1 | Laceration with edges that approximate | None or minor muscle tissue necrosis; some muscle tissue injury with intact muscle unit function | No major vessel disruption | No grossly visible contamination | None or insignificant bone loss |
2 | Laceration with edges that does not approximate | Loss of significant muscle tissue but the involved muscle unit remains in longitudinal continuity | Vessel injury without distal ischemia | Surface contamination, visible | Bone missing or devascularized bone fragments, but some contact between proximal and distal fragments |
3 | Laceration associated with extensive degloving | Extensive muscle necrosis with loss of muscle tendon unit continuity, (partial or complete compartment excision, muscle defect that does not reapproximate) | Vessel injury with distal ischemia | Contaminant embedded in bone or deep soft tissues or high risk environmental conditions (barnyard, fecal, dirty water, etc.) | Segmental bone loss |
Per definition, an open fracture requires communication of the fracture site with a skin discontinuity. If exclusion is not possible or there remains any doubt, the fracture should be treated as an open fracture.
Regardless of the soft tissue damage X-rays in two views (ap. and lateral) with adjacent joints should be performed. Dependent on the patient’s status articular fractures should be visualized with CT imaging before definitive treatment is performed.
19.3 Surgical Treatment
19.3.1 Hair Removal
Removal of gross contamination should be performed before disinfection of the surgical site. Historically, preparation for surgery included routine hair removal of the surgical site. Although no studies are specifically focusing on open fracture treatment, a recent Cochrane review was not able to recommend a strategy for or against hair removal before surgery. When hair removal is necessary, a clipper should be used since shaving was associated with an increased incidence of surgical site infections [19].
19.3.2 Debridement and Irrigation
Debridement and irrigation remain the key steps in surgical treatment of open fractures. Small puncture wounds or lacerations need to be extended until complete soft tissue damage can be evaluated. Debridement should include grossly contaminated and/or devitalized soft tissue. Since perfusion is impaired in this tissue the host defense system is not able to eliminate foreign organisms. Moreover, if dead or contaminated tissue remains it serves as a medium for bacterial growth. Thus, debridement of subcutaneous tissue, muscle, and fascia is necessary until viability of marginal tissue is preserved and a clean and stable wound is achieved.
Fragments of devitalized bone or bone unattached from soft tissue should be discarded (Fig. 19.2). A most recent study comparing more or less aggressive bone debridement in type II and III open supracondylar femur fractures suggests bone debridement restricted to grossly contaminated bone “with retention of other bone fragment” and without use of antibiotic cement spacers. The less aggressive protocol leads to more frequent healing after the index procedure without increasing the incidence of infections [20] suggesting a cautious debridement.
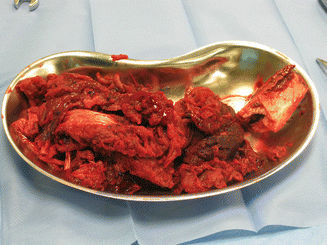
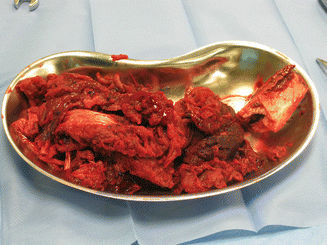
Fig. 19.2
Discarded contaminated and devitalized soft tissue and isolated bone fragments after thorough debridement of an open fracture of the lower leg
In fractures with extensive soft tissue injury, initial evaluation of tissue viability is difficult. In those patients, a repeat debridement after 48–72 h “to eliminate devitalized tissue that subsequently develops” should be performed [21].
Multiple irrigation protocols in open fracture treatment including distilled water, boiled water, soap, antibiotic solutions as well as antiseptic solutions are described [22–24]. Regarding antiseptic solutions, povidone iodine wound irrigations [25, 26] and chlorhexidine gluconate [27] were evaluated. These antiseptic solutions show a broad spectrum activity versus antimicrobials including bacteria, viruses, and fungi [28]. However, to date, all clinical trials failed to show beneficial results regarding the incidence of infections. These observations are supported from experimental studies in which various antiseptic irrigation solutions were tested [29–31]. Moreover, toxicity towards intrinsic cells may cause problems in wound healing.
In a recent prospective randomized trial comparing soap solution with normal saline, no differences regarding infection, wound healing problems, and nonunion were observed [32].
In experimental studies, use of antibiotic solutions with an antimicrobial effect was able to show beneficial effects in infection prevention as compared to normal saline which could not be confirmed in human studies [33]. In an international survey, the majority of responding surgeons favored normal saline solution for irrigation in open fractures [34].
To date, there is no convincing data showing superior effects of solutions other than normal saline for irrigation of wounds associated with open fractures.
Pulsatile lavages for wound irrigation using different pressures are commercially available (Fig. 19.3). Under experimental settings, high pressure pulsatile lavage caused penetration of particulates up to 15.6 mm; cellular damage was detected 1.3 mm under the surface while low pressure pulsatile lavage showed penetration of particulates and cell death in 0.7 mm below tissue surface [35]. In parallel, bacterial penetration was twofold increased by high pressure pulsatile lavage and number of retained bacteria was two- to fourfold lower after low pressure pulsatile lavage [36]. In another study, propagation of bacteria into the intramedullary canal due to high pressure irrigation was detected [37]. High pressure pulsatile lavage showed no beneficial effects regarding removal of adherent bacteria after 3 h delay as compared to low pressure pulsatile lavage. In contrast after 6 h delay, only high pressure pulsatile lavage removed adherent bacteria from bone [38].
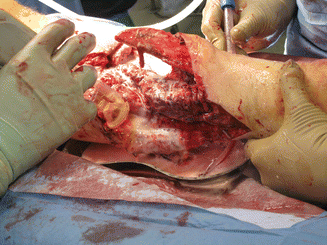
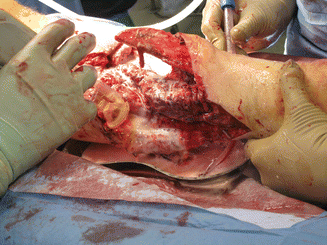
Fig. 19.3
Low pressure pulsatile lavage during the initial surgical debridement
Experimental studies investigating the effect of high pressure pulsatile lavage consistently showed detrimental effects on fracture healing. In a rabbit model, high pressure pulsatile lavage resulted in diminished bone density during the early phase (2 weeks) of fracture healing [39]. Mechanical testing of fractured femora in a rat model revealed a decreased mechanical stability 3 weeks after high-pressure irrigation [40].
In a clinical study, the use of low pressure pulsatile lavage showed superior results regarding the infection-associated reoperation rate when compared to high pressure devices [32].
Low pressure pulsatile lavage seems sufficient for bacterial clearance and is associated with less detrimental effects regarding propagation of bacteria and bone healing.
19.3.3 Time to Debridement
Three decades ago, initial treatment of all open fractures was stated as an emergency procedure [41]. In accordance, clinical guidelines recommended initial debridement within 6 h following injury, commonly known as the “six-hour rule” [42]. Circumstances of infrastructural or human resources as well as the patient’s condition may delay the period between injury and surgical treatment raising the question of the consequences. A recent systematic review including six prospective and ten retrospective cohort studies with 3539 open fractures provides an overview about this issue. For type I and II injuries, the infection rate was 12 % following early (within 6 h) and 5 % following late debridement without reaching statistical significance [42]. In type III fractures, early debridement resulted in 15 % and late debridement in an 11 % infection rate without reaching statistical significance [42]. A beneficial effect of early surgical debridement could not be shown in dependence of anatomic location or when only deep infections were considered. In children, delay in surgical debridement (>6 h) did not influence the rates of acute infection as well [43]. Additionally, early debridement did not lead to improved bone healing [44].
Surgical debridement should be performed within 12 h after injury. There seems no evidence for the historical six-hour rule in the current literature.
19.4 Antibiotic Treatment
Superficial and deep infections including bony structures and joints remain a major problem in patients suffering from open fractures. In polytraumatized patients, reduced perfusion of the fracture may additionally impair the body’s response to contamination. Besides surgical debridement, administration of antibiotics is recommended in various guidelines [45, 46]. The administration of antibiotics before or at the time of primary treatment reduced the number of early infections independently of agents used or duration [8]. There is consensus that administration should be initiated within 1 hour after detection of an open fracture [47]. Prehospital treatment of open fractures with antibiotics was associated with a shortened time interval between injury and antibiotic administration. However, it did not show an additional benefit regarding incidence of infections or impaired fracture healing [48].
Multiple studies investigated the effectiveness of different antibiotics for infection prevention [8, 46]. Although antibiotic treatment is routinely used for therapeutic purposes in open fractures, most studies suffer from “methodologic problems, including commingling of prospective and retrospective data sets, absence of or inappropriate statistical analysis, lack of blinding, or failure of randomization” [46]. However, the most current Cochrane review states that “further placebo controlled randomized trials are unlikely to be justified in middle and high income countries” [8].
For Type I and type II fractures, it is recommended to cover gram-positive germs, especially against Staphylococcus aureus [46]. Antibiotic treatment of type III fractures should be effective against gram-negative bacteria and therefore additional treatment with an aminoglycoside is recommended [8].
Duration of treatment with antibiotics is mostly dependent on wound closure. In most studies, antibiotic coverage for 24 h after primary closure was sufficient. Thus, antibiotic coverage for 2–3 days should be sufficient for type I and II fractures. More severe open fractures may benefit from prolonged treatment.
Systemic antibiotic treatment should be initiated as early as possible after injury. Cephalosporines covering a gram positive spectrum can be used for type I and II injuries, for type III fractures an aminoglycoside should be added to cover gram-negative bacterias.
Local antibiotic administration using the bead pouch technique produce high local levels of antibiotics in the wound. This should inhibit local bacterial growth without side effects induced by systemic administration. Especially in complication wounds that cannot be initially closed local antibiotic treatment offers a tool in wound management. In an animal study, deleterious effects of gentamycine on bone healing were excluded [49]. The advantage of high concentrations of locally administered antibiotics seems questionable after introducing negative-pressure wound therapy for temporary closure [50].
Only few retrospective clinical trials investigated the benefit of local antibiotic administration. In a prophylactic approach, local antibiotic treatment with tobramycin-impregnated bead chains decreased incidences of wound infections and osteomyelitis when compared to a systemic antibiotic triple therapy with cefazolin, tobramycin, and penicillin [51]. The beneficial effect of tobramycine beads seems pronounced in higher degree open fractures [52, 53]. The same authors confirmed the results in a study including more than 1000 patients in which local aminoglycoside treatment resulted in a decrease of the infection rate from 12 to 3.7 % [54]. The only prospective study comparing local and systemic antibiotic treatment included 67 patients with 75 open fractures. No difference regarding incidence of infection was detected. In addition, local treatment in conjunction with intravenous antibiotics did not show an additional benefit [55].
According to the actual literature, a beneficial effect of local antibiotic administration in open fractures is limited based on studies with low evidence.
19.5 Fracture Stabilization
Mechanical stabilization of open fractures is a key step in the treatment strategy. Surgical stabilization of the fracture prevents secondary damage to adjacent soft tissue, and decreases the incidence of infection. Various factors including patient’s parameter (e.g., age, comorbidities, etc.), additional injuries, and localization of the fracture influence the type of fracture stabilization. Common techniques include external fixation, intramedullary stabilization, conventional and locking plate osteosynthesis as well as screw and wire fixation. During the last two decades the concept of damage control orthopedics changed treatment strategies in polytraumatized patients [56, 57]. Considering the patient’s status with regard to hemodynamics as well as head and thoracic injuries temporary stabilization of long bone fractures with external fixation devices (Fig. 19.4a, b) will reduce further surgical harm and therefore prevent an exaggerated posttraumatic immune response [58, 59].
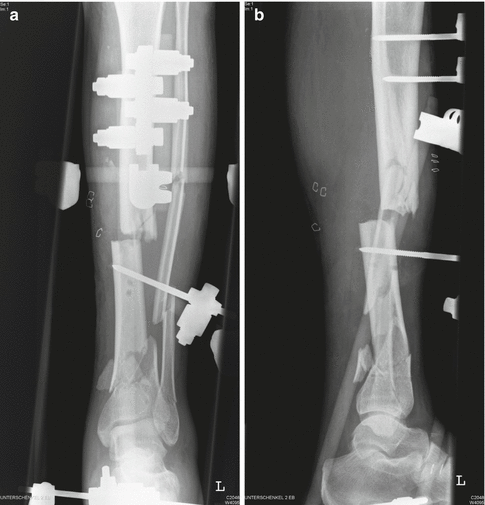
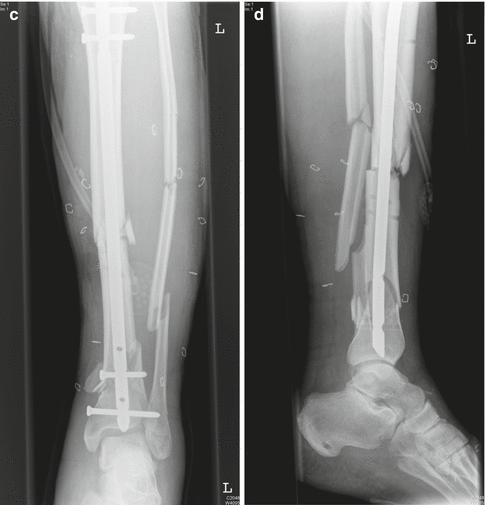
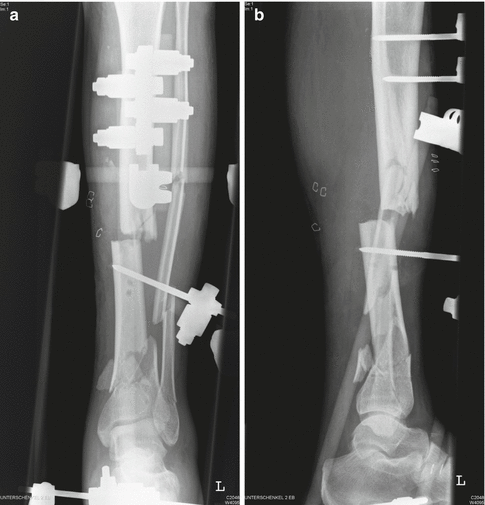
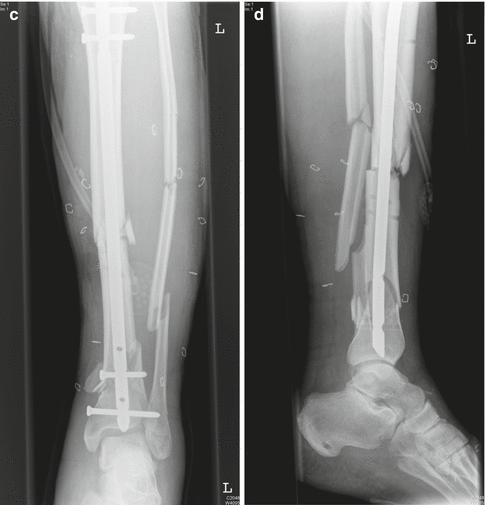
Fig. 19.4
X-ray of temporary external fixation in an open fracture of the lower limb (Type IIIb following the Gustilo classification) in ap. (a) and lateral (b) view. X-ray of definitive stabilization with a locking nail in ap. (c) and lateral (d) view
Insofar, complex reconstruction of articular surfaces is not part of the initial management. In these cases, mechanical stabilization is achieved with a temporary external fixation. Placement of pins should consider future surgical approaches, implant placement or local flaps.
Fixation with external devices is fast and easy to handle but a high incidence of pin tract infections, difficulties in soft tissue management and malunions are described when definitive stabilization was attempted [60]. When external fixation is used as a bridging device until the patient is stabilized and definitive internal fixation is performed during the clinical course no increased risk of infectious complications was evident [61]. Moreover, results regarding osseous healing showed a 97 % union rate at 6 months in open and closed femoral fractures following temporary stabilization [62]. Consistently, the interval between temporary external stabilization and definitive intramedullary nailing influences incidence of complications. The delay before conversion was identified as a risk factor for infections [61, 63, 64].
External fixation as definitive treatment has shown an increased risk for reoperation, nonunion, and infection as compared with unreamed nailing [65].
Definitive treatment of diaphyseal fractures with intramedullary nailing (Fig. 19.4c, d) should be considered in patients in type I and II fractures in whom adequate soft tissue coverage is possible and no severe contamination is present. Although only minimal incisions are required preventing further damage to the soft tissue in the fracture region, nail locking must be possible.
The debate of reamed versus unreamed nailing remains in the current literature. In a prospective multi-center study (SPRINT) including over 1300 patients with open and closed tibial fractures, no differences regarding required revision surgery between reamed and unreamed nailing were revealed. However, patients with open fractures treated with reamed nailing had an increased risk for revision surgery as compared to unreamed nailing [66]. In contrast, reamed nailing showed superior results in closed fractures [66]. An earlier meta-analysis including 2 randomized trials with 132 patients did not show any effects regarding revision surgery, infections or mal- and nonunions [65]. Nonetheless, an insignificant advantage for reamed nailing was detected which is in contrast to the SPRINT study [65].
Primary plate osteosynthesis for fixation in open fracture has been shown to be associated with high infection rates [67, 68]. Although introduction of locking plates allows minimal invasive surgical techniques, infection rates remain high [69]. In contrast, staged protocols including temporary external fixation were shown to decrease infection rates [70].
An algorithm for fracture stabilization is provided in Fig. 19.5.
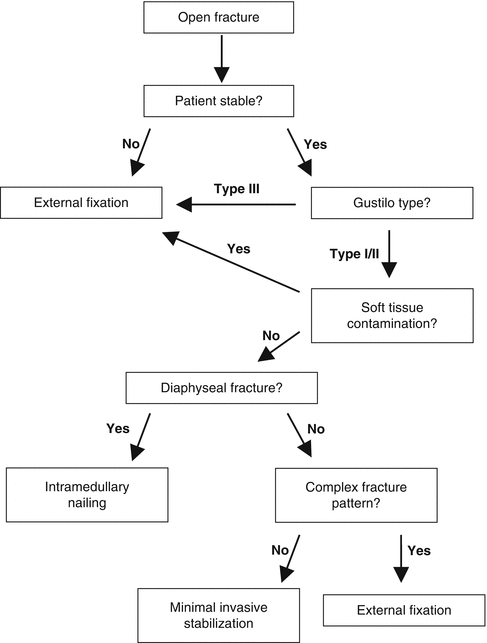
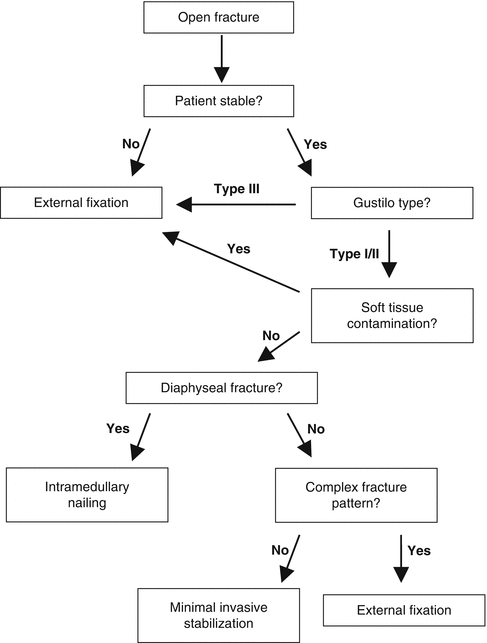
Fig. 19.5
Algorithm for fracture stabilization considering patient’s status and local injury characteristics
19.6 Timing of Wound Closure
The question of wound closure timing remains a controversial debate since more than 25 years ago a landmark paper about emergency free flaps was published in 1988 [71]. Approximately 10 years before, open wound management was still propagated to prevent fatal infections such as gas gangrene [72]. The advantage of emergency free tissue transfer was seen in reduced infection rates occurring in longstanding open wounds [73]. Moreover delayed wound closure prolongs in-hospital time as well as treatment costs. A reduced infection rate and time to union due to microsurgical reconstruction within 72 h following injury was published 2 years earlier by Godina with inclusion of 532 patients [74]. The concept of early wound closure was supported by microbiologic analyses showing that initial microbial contamination before surgical debridement was not predicting occurrence of infections. In a prospective study investigating 117 open fractures, in only two of seven infections initial wound cultures were positive [75]. In another study, 39.3 % of predebridement swabs were positive while none of them developed an infection [76]. To date, the concept of fix and flap is still used and excellent results regarding infection rate, limb salvage as well as function were reported in a retrospective study [77]. However, the evidence for immediate closure remains low with mostly retrospective studies conducted before vacuum-assisted wound closure (VAC) was introduced. Additionally, most of the studies investigating early versus delayed wound closure were criticized since a selection bias with less severe injuries in the early closure group was evident [78, 79].
Nowadays, the concept of immediate or early wound closure requiring microsurgical techniques comes into conflict with the damage control approach. Free tissue transfer may take several hours and require repositioning of the patient during the surgical procedure [80] which may not be tolerated from patients suffering from severe head, pulmonary, and abdominal trauma.
Delayed wound closure was already suggested in 1959 when problems with more severe open fractures occurred [11]. The well-known study by Gustilo and Anderson revealed an increased infection rate up to 44 % in open segmental tibial fractures with extensive tissue injury [12]. Hence, they concluded that type I and type II injuries could be primarily closed while type III open fractures should benefit from a delayed closure. Following this treatment protocol a reduced infection rate from 14 to 4.5 % was observed [81].
In general, studies favoring delayed closure or not showing significant differences show a higher level of evidence with mostly prospective studies. Another problem remains with the different definitions of time periods referring to early and late wound closure [82] impeding the comparability of published studies.
The introduction of VAC-systems (Fig. 19.6) provided a bridging tool until definitive wound coverage can be performed. Negative-pressure wound therapy prevents further contamination and may increase perfusion close to the wound edge [83]. The VAC therapy stimulates granulation tissue, supports skin grafts and therefore prevents requirement of more complex reconstruction procedures [84, 85]. Treatment with VAC resulted in decreased edema improving local tissue perfusion which may have positive effects on wound healing [85]. Although a diminished number of bacteria due to VAC therapy were advocated, recent studies question this hypothesis [86, 87]. For type III open diaphyseal tibial fractures a reduction of required free tissue transfer due to application of negative-pressure wound therapy was shown (Fig. 19.7) [88].