Questions
- 1.
Describe the anesthetic evaluation before lung resection.
- 2.
- 3.
What are the indications for one-lung ventilation?
- 4.
Describe the use of single-lumen endotracheal tubes and bronchial blockers for one-lung ventilation.
- 5.
If a double-lumen tube is to be used, which would be preferable, a left-side or right-side tube?
- 6.
Describe the proper technique for placing a double-lumen endobronchial tube.
- 7.
How is correct positioning of the double-lumen endobronchial tube assessed?
- 8.
What clinical problems are associated with placement and use of double-lumen endobronchial tubes?
- 9.
What complications are related to placing the patient in the lateral decubitus position?
- 10.
How are pulmonary perfusion and ventilation altered during one-lung ventilation?
- 11.
How is hypoxemia that occurs during one-lung ventilation treated?
- 12.
Describe the role of a thoracostomy tube after pulmonary resection.
- 13.
Describe a commonly used pleural drainage system.
- 14.
A 70-year-old man presents for resection of a left upper lobe tumor. He has a long history of cigarette smoking.
1
Describe the anesthetic evaluation before lung resection.
Preoperative evaluation begins with the same basic information required before all anesthetics. Next, it is determined whether the patient is in optimal condition for the planned procedure or whether further preoperative evaluation or preparation is indicated. Finally, an assessment is made to predict lung function after resection. Specific pulmonary evaluation includes history of cough, sputum production, chest pain (possibly pleuritic), dyspnea, wheezing, arm pain (resulting from a Pancoast tumor invading the brachial plexus), weakness (resulting from myasthenic syndrome), other endocrine syndromes (caused by tumors secreting hormones), and weight loss (hypoproteinemia). Physical examination includes auscultation for wheezing, rales, and rhonchi. Wheezing may require treatment with bronchodilators, and infected sputum is treated with antibiotics. Chest radiograph, tomography, computed tomography, and magnetic resonance imaging provide further information about the tumor site, structures involved, and possible airway compromise.
Lung function tests are indicated to predict the risk of respiratory failure, right heart failure (cor pulmonale), and atelectasis and to guide bronchodilator therapy. Spirometry is a noninvasive test that provides data on lung volumes and gas flow rates. Flows are tested before and after bronchodilator therapy, such as albuterol by inhaler, to determine reversible obstructive airway disease. Improved flows after bronchodilator therapy indicate preoperative adjustments in the bronchodilator regimen.
Pulmonary function tests that may correlate with outcome include forced expiratory volume in 1 second (FEV 1 ), forced vital capacity (FVC), maximum voluntary ventilation, and ratio of residual volume to total lung capacity (RV/TLC). Based on how much lung tissue is to be resected, predicted postoperative (PPO) function can be calculated. A PPO FEV 1 >40% has been associated with improved outcome. FVC <50% of predicted or RV/TLC >50% of predicted may indicate higher risk.
Spirometry reflects respiratory mechanics, whereas gas exchange is assessed by measuring the diffusing capacity for carbon monoxide (DLCO). A PPO DLCO <40% indicates reduced lung parenchymal function and is a predictor of increased risk.
Cardiopulmonary reserve can be evaluated by measuring the maximal oxygen consumption ( O 2 max). A
O 2 max <10 mL/kg/min indicates very high risk, and a
O 2 max >20 mL/kg/min indicates reduced risk. Other tests of cardiopulmonary reserve include exercise oximetry, in which a decrease in oxygen saturation by pulse oximetry (SpO 2 ) of 4% during exercise may indicate increased risk. A simple test is stair climbing; inability to climb more than one flight of stairs is associated with increased risk, and ability to climb three or more flights of stairs reflects decreased risk.
If lung function results are poor, split lung function testing (ventilation/perfusion radiospirometric studies) can be done to allow calculation of PPO lung function. Ultimately, the operative decision to perform pneumonectomy versus lobectomy versus wedge resection is a clinical one in which the patient’s overall condition is considered.
2
How are ventilation and oxygenation monitored noninvasively during surgery, and how do these monitors work?
The purpose of ventilation is to remove carbon dioxide (CO 2 ) from the lungs. The average 70-kg man produces approximately 220 mL per minute of CO 2 and normally maintains an arterial carbon dioxide tension (PaCO 2 ) of 40 mm Hg. If removal of CO 2 is impaired or if production increases and ventilation does not increase, PaCO 2 increases. Arterial blood gas analysis provides the ultimate monitor of ventilation: PaCO 2 . In the lungs, CO 2 diffuses into alveoli, and in areas where ventilation and perfusion are well matched, alveolar CO 2 tension (P A CO 2 ) approximates PaCO 2 . P A CO 2 may be sampled as end-tidal carbon dioxide (P ET CO 2 ) and analyzed by various technologies to provide a noninvasive estimation of PaCO 2 . Overall, P ET CO 2 is normally 4–6 mm Hg less than PaCO 2 . Increases in physiologic dead space or a decrease in alveolar ventilation results in increased PaCO 2 .
Capnography is the science of measuring and displaying CO 2 as a concentration plotted against time (capnogram). The CO 2 concentration in gas mixtures may be measured using many technologies, the most common of which is infrared light spectroscopy. Although mass spectrometry, infrared (IR) acoustic spectroscopy, and Raman scattering have been used in the past, the monitors that used these technologies are no longer in production. IR light spectroscopy is based on 4.3 μm (4300 nm) wavelength IR radiation absorbance by intermolecular bonds in the CO 2 molecule. The greater the number of CO 2 molecules present, the greater the absorbance of radiation. Gas is drawn through a sample cell (cuvette), and IR light of 4.3 μm is passed through the cell. Transmission of light through the gas sample to a photodetector is inversely proportional to the tension of CO 2 in the gas sample. Such systems are in widespread use, and sampling may be of the sidestream or mainstream (in the airway) design.
By continuously following the tension of CO 2 against time, the highest value is designated end-tidal and defines exhalation, and the lowest value is designated inspiratory and defines inspiration. Continuous capnography is considered the standard of care because it provides a continuous monitor of ventilation and, with certain limitations, can provide a noninvasive estimate of PaCO 2 .
Oxygenation is monitored best by sampling arterial blood and analyzing it for tension (PaO 2 ), hemoglobin (Hb) saturation with oxygen (fractional saturation, i.e., O 2 Hb/total Hb), and total Hb. The oxygen content of arterial blood (CaO 2 ) is given by the following equation:
CaO 2 = [(Hb × 1.34 × SaO 2 ) + (PaO 2 × 0.003)]
Normal arterial oxygen content can be calculated by substituting variables as shown, assuming that the patient is breathing room air, the SpO 2 is 97%, and the PaO 2 is 97 mm Hg:
CaO 2 = [(15 × 1.34 × 0.97) + (97 × 0.003)] = 19.5 + 0.29 = approximately 20 mL oxygen/100 mL blood
However, this calculation requires invasion of an artery for blood sampling.
Noninvasive monitoring of oxygenation is most commonly achieved using pulse oximetry, which is a standard of care. Pulse oximetry uses a combination of two technologies: (1) spectrophotometry of oxygenated and deoxygenated Hb and (2) optical plethysmography. The latter detects pulsatile components of changes in light transmitted through tissue. Each pulse oximeter probe has two light-emitting diodes that emit light at 660 nm and 940 nm as well as one photodetector. The ratio of the pulse-added absorbances at 660 nm to that at 940 nm is related through an empirically derived algorithm, to a saturation estimate that is designated SpO 2 . Pulse oximeter readings have a standard deviation of approximately +2% in the saturation range of 70%–100%. The accuracy decreases at lower SpO 2 levels. Nevertheless, pulse oximeters are valuable as monitors of saturation trends. Pulse oximeters are inaccurate or may fail in the presence of poor perfusion (e.g., vasoconstriction from low cardiac output or cold extremities), venous pulsations, severe peripheral vascular disease, intravascular dyes, dyshemoglobins, certain nail polishes, and certain pigmentations. If doubt exists regarding the validity of pulse oximeter readings, an arterial blood gas sample should be drawn for blood gas tension analysis and saturation analysis in a laboratory cooximeter.
3
What are the indications for one-lung ventilation?
One-lung ventilation is indicated for isolation and protection of one lung from contamination by the other lung or for selective ventilation of one lung only. Indications for separating the lungs include prevention of blood or pus spillage and bronchopulmonary lavage. Situations in which hemorrhage or infectious material might affect the contralateral lung include bronchiectasis, lung abscess, and hemoptysis. The ability to control the distribution of ventilation between lungs improves exposure for thoracic surgery. Positive pressure ventilation through a single-lumen tube risks losing large amounts of tidal volume through a bronchopleural fistula or pneumothorax in the case of a giant unilateral lung cyst. Surgical exposure is improved during pneumonectomy, descending thoracoabdominal aortic aneurysm repair, and resection of midesophageal and upper esophageal lesions. One-lung ventilation is needed for video-assisted thoracoscopy because the surgeon cannot operate endoscopically on an inflated lung.
4
Describe the use of single-lumen endotracheal tubes and bronchial blockers for one-lung ventilation.
Bronchial blockers can be placed through single-lumen tracheal tubes. This practice eliminates the need for changing a single-lumen tube to a double-lumen tube (DLT) at the beginning of a case in which single-lumen tubes had been placed for an initial bronchoscopy. It also eliminates the need for replacing DLTs with single-lumen tubes at the conclusion of surgery. That might be required if bronchoscopy is performed or preferred if the patient’s airway is to be kept intubated. When using bronchial blockers, single-lumen tubes of sufficient diameter are employed to accommodate both the blocker and a fiberscope, which is needed for correct placement. Endotracheal tubes cannot be too close to the carina, especially in the case of left-sided thoracic surgery, because there will not be enough space to manipulate a blocker into the left main stem bronchus. It may be necessary to rotate the endotracheal tube such that the concave side is on the left or to turn the patient’s head to the right for the bronchial blocker to enter the left main stem bronchus.
The most commonly used bronchial blockers are the Uniblocker (Fuji Systems, Tokyo, Japan), Cohen blocker (Cook, Bloomington, IN), and Arndt blocker (Cook). The first commercially available blocker was the Univent tube (Fuji Systems). The Univent tube is a combined single-lumen endotracheal tube and an integral bronchial blocker. The single-lumen tube is equipped with a narrow internal lumen through which a balloon-tipped bronchial blocker is advanced into the right or left main stem bronchus. With the bronchial blocker retracted and deflated, the tube is positioned by standard techniques into the trachea. The tube is turned 90 degrees toward the side to be blocked, and the blocker is advanced under flexible fiberoptic bronchoscopic guidance into the appropriate bronchus. The balloon is inflated, and lung separation is confirmed by auscultation of breath sounds. More rapid collapse of the lung follows deflation of the blocker balloon and disconnection from the anesthesia breathing circuit. After successful lung collapse, reinflation of the blocker’s balloon and connection to the anesthesia breathing circuit allow for ventilation of one lung and collapse of the other. The blocker of the Univent tube is now available as an independent blocker, the Uniblocker, which is bent near the tip. Because the lumen of a bronchial blocker is small, deflation of the blocked lung is slower than when a DLT with larger lumens is used. When placed, a blocker tends to dislocate more than a DLT, especially when placed on the right side because of its location near the carina. There is more space in the left main stem bronchus to seat the balloon of the bronchial blocker.

Full access? Get Clinical Tree
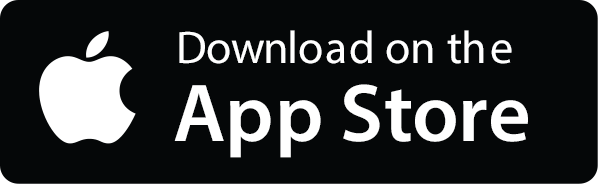
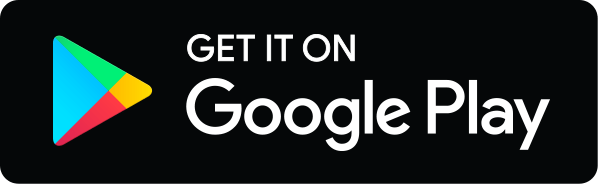