Treatment
The only effective long-term means of reversing malignancy-associated hypercalcemia is reduction in tumor burden (Table 147.2). Antihypercalcemic therapy is a temporizing measure that does not affect survival (5). The aggressiveness of the therapeutic approach depends on the potential for palliation and cure. When all antitumor strategies have failed, or in patients who do not wish to pursue further treatment of their cancer, an ethical, humane, and appropriate approach may involve withholding antihypercalcemic treatment (5,9).
Stewart (5) has classified hypercalcemia based on serum calcium levels into mild hypercalcemia (10.5 to 11.9 mg/dL), moderate hypercalcemia (12.0 to 13.9 mg/dL), and severe hypercalcemia (14.0 mg/dL or greater). In addition to the magnitude of hypercalcemia, the severity of symptoms and the underlying cause are important factors in formulating an appropriate treatment strategy. In general, severe hypercalcemia requires emergent, aggressive treatment in the presence or absence of symptoms, whereas interventions in mild-to-moderate hypercalcemia are contingent on the severity of the symptoms. Prior to initiating therapy, the clinician should assess the patient for correctable factors that may contribute to hypercalcemia such as calcium-containing intravenous fluids, parenteral nutrition, and oral calcium supplements. In addition, thiazide diuretics, vitamins A and D, calcitriol, lithium, and estrogens or antiestrogens should be held (11). Immobilization is a well-established cause of hypercalcemia, and weight-bearing ambulation is recommended whenever possible (24). Finally, hypercalcemia is more difficult to treat in the setting of hypophosphatemia; oral or nasogastric phosphate supplementation should be administered to keep the calcium-phosphate product between 30 and 40. Intravenous phosphorous replacement may precipitate hypocalcemia, seizures, and acute renal failure, and is reserved for patients in whom oral or nasogastric administration cannot be performed (5).
Fluids and Diuretics
The initial intervention in the treatment of hypercalcemia is the administration of isotonic saline at a rate of 200 to 500 mL/hr based on the degree of hypovolemia and renal and cardiovascular dysfunction to maintain urine output of 150 to 200 mL/hr (5). Once the fluid deficit is replaced, the infusion rate should be decreased to 100 to 200 mL/hr in patients without cardiac or renal impairment (20). The patient must be carefully monitored to prevent fluid overload. Saline hydration reduces serum calcium level by increasing the glomerular filtration rate and increasing calcium delivery to the proximal tubule where urinary calcium excretion is augmented by the calciuric effects of saline (5).
Loop diuretics inhibit calcium reabsorption at the loop of Henle, increasing calciuresis. These agents should be used judiciously and only after euvolemia is achieved in hypovolemic patients or in patients who present with volume overload (5,9,18). Because of ensuing complications such as hypokalemia, hypomagnesemia, and volume depletion, and because of the availability of bisphosphonates, loop diuretics are used less favorably in clinical practice (24).
TABLE 147.2 Treatment of Hypercalcemia of Malignancy | |
![]() |
Bisphosphonate Therapy
Bisphosphonates inhibit osteoclastic bone resorption and are the principal agents used in the management of hypercalcemia of malignancy (25,26). When compared to saline and diuretics alone, and other antiresorptive agents including calcitonin, bisphosphonates are superior in treating hypercalcemia of malignancy (5). Because only 1% to 2% of oral bisphosphonates are absorbed, these drugs are administered intravenously (7). Pamidronate and zoledronate are the most commonly used bisphosphonates for the treatment of hypercalcemia of malignancy. Patients respond to bisphosphonate therapy within 2 to 4 days, with a nadir in serum calcium occurring within 4 to 7 days; normocalcemia may persist for 2 to 4 weeks (5,16). Zoledronate is 850 times more potent than pamidronate and is considered first-line therapy (9,27). In a pooled analysis of two randomized controlled trials comparing a single 4-mg dose of zoledronic acid to a 90-mg dose of pamidronate, serum calcium concentrations normalized within 10 days in 88% versus 70% of patients, respectively, and the duration of response was 32 days versus 18 days, respectively, within the two groups (27). Both zoledronate and pamidronate have been associated with acute and chronic renal failure, with more adverse events reported with zoledronate. Dose reduction of zoledronate is recommended in patients with a creatinine clearance between 30 and 60 mL/min. Other complications of bisphosphonates include acute systemic inflammatory reactions such as fever and arthralgias, ocular inflammation, electrolyte imbalances, and osteonecrosis of the jaw (28).
Other Agents
Calcitonin is a well-tolerated synthetic polypeptide analog of salmon calcitonin, which reduces serum calcium levels by inhibiting bone resorption. When administered subcutaneously or intramuscularly, it produces a rapid but transient decrease in serum calcium levels within 12 to 24 hours (5,9). This agent is useful in patients with congestive heart failure (CHF) or renal failure where saline, diuresis, and bisphosphonates may be contraindicated. Tachyphylaxis may occur with continued use (7). Glucocorticoids are effective in decreasing serum calcium in hypercalcemia of malignancy associated with some lymphomas, particularly Hodgkin lymphoma (HL). These agents have limited utility in the acute setting because a reduction in serum calcium may not be observed for 1 to 2 weeks (11).
Denosumab is a human monoclonal antibody that inhibits osteoclast formation by preventing human receptor activator of nuclear factor kappa-β ligand (RANKL) from binding with its receptor. It is metabolized and excreted by the liver and considered safe to give to patients with renal insufficiency although optimal dosing is uncertain. In patients with multiple myeloma, hypercalcemia, and severe renal impairment (serum creatinine 2.5 to 5.7 mg/dL), denosumab improved serum calcium and was associated with improved in renal function (29). In patients with breast cancer metastatic to bone, denosumab proved superior to zoledronic acid in preventing skeletal-related events such as pathologic fractures (30). It has also proved efficacious in patients with persistent hypercalcemia despite bisphosphonate therapy (31,32).
Dialysis may be necessary for patients with severe malignancy-associated hypercalcemia and concomitant renal insufficiency or heart failure, who will not tolerate the therapies outlined above (5,16).
Controversies
As noted above, the use of loop diuretics has fallen out of favor in the routine management of hypercalcemia. These agents may be necessary, however, to prevent fluid overload in selected individuals with renal insufficiency or heart failure.
ACUTE TUMOR LYSIS SYNDROME
Acute tumor lysis syndrome (ATLS) occurs as a consequence of the rapid and massive destruction of tumor cells resulting in the release of intracellular metabolites into the circulation in quantities sufficient to exceed renal excretory capacity (33,34). The four biochemical disturbances generated by this process that characterize the syndrome are life threatening (35):
- Hyperkalemia
- Hyperphosphatemia
- Hypocalcemia
- Hyperuricemia
These metabolic abnormalities have widespread adverse effects on the cardiac, musculoskeletal, nervous, and renal systems.
ATLS is most frequently observed after the administration of cytotoxic chemotherapy in patients with high-grade hematologic malignancies—classically, Burkitt lymphoma and acute lymphocytic leukemia (ALL) (7,36,37). The incidence of clinically significant ATLS in non-Hodgkin lymphoma (NHL) and ALL has been reported as 6% (38) and 5.2% (7), respectively. Metabolic derangements in these patients may develop within a few hours to a few days after initiating chemotherapy (7,39–41). Other malignancies in which ATLS has been described include chronic leukemia, low-grade lymphoma, and, rarely, solid tumors such as metastatic breast carcinoma, lung carcinoma, seminoma, thymoma, medulloblastoma, ovarian carcinoma, rhabdomyosarcoma, melanoma, vulvar carcinoma, and Merkel cell carcinoma (34). The syndrome can also occur after radiation therapy, immunotherapy with rituximab, and endocrine therapy (7,34,36). Spontaneous tumor lysis syndrome (STLS) is a rare entity that develops primarily in Burkitt lymphoma and leukemia in the absence of any treatment. Prompt recognition of ATLS is essential because it is associated with poor outcomes and high mortality rates (41). Predisposing factors for developing ATLS include large tumor burdens (36), bulky lymphadenopathy (7), extensive bone marrow involvement (37), rapid tumor cell proliferation, leukocytosis (more than 50 × 103 cells/μL) (34), lactate dehydrogenase (LDH) more than 1,500 IU/L (37), and high tumor chemosensitivity (7,35). Pretreatment hyperuricemia, renal dysfunction, and hypovolemia, as well as treatment with nephrotoxic agents, also confer an increased risk of ATLS (34).
Pathophysiology
Rapid dissolution of cells with aggressive cytotoxic therapy results in an increase in plasma uric acid, potassium, and phosphorus levels. Hyperphosphatemia then leads to secondary hypocalcemia; hyperkalemia occurs 6 to 72 hours after the administration of chemotherapy (39). Associated manifestations include lethargy, nausea, vomiting, diarrhea, muscle weakness, paresthesias, and electrocardiographic abnormalities such as peaked T waves, PR-interval prolongation, and QRS-complex widening. Ventricular arrhythmias may lead to sudden death (7,34). Hyperphosphatemia is seen 24 to 48 hours following chemotherapy (39). Malignant cells may contain up to four times more phosphorous than nonneoplastic cells and, as plasma phosphorous increases with cell lysis, the normal renal mechanism that excretes excess phosphate and prevents distal tubular reabsorption becomes overwhelmed, leading to hyperphosphatemia (42). Acute hyperphosphatemia manifests as secondary hypocalcemia with a range of signs and symptoms including anorexia, vomiting, confusion, neuromuscular irritability, tetany, carpopedal spasm, seizures, dysrhythmias, and cardiac arrest (7,34). Secondary hypocalcemia occurs in association with hyperphosphatemia when the calcium phosphate product exceeds 60. Calcium phosphate then precipitates into tissues, including the renal interstitium and tubules, resulting in nephrocalcinosis (35). However, hypocalcemia may persist even after correction of hyperphosphatemia when an inappropriately low plasma calcitriol level is present (43). Hypocalcemia itself causes a rise in serum parathyroid hormone, which, in turn, increases phosphate resorption in the proximal tubule, leading to nephrocalcinosis and acute renal failure (ARF) (7).
Hyperuricemia occurs 48 to 72 hours after chemotherapy (39). Patients may exhibit nonspecific symptoms such as nausea, vomiting, anorexia, and lethargy. ARF with associated oliguria, edema, hypertension, and altered sensorium will be seen in untreated patients (36). Uric acid is generated from purine metabolism in the liver. Adenosine and guanosine nucleotides are degraded to hypoxanthine and xanthine, respectively, and xanthine oxidase converts these products to uric acid (7). Rapidly proliferating neoplastic cells have high turnover rates with accelerated purine catabolism from DNA and RNA degradation (44), and these cells contain large amounts of purine nucleotides; consequently, with cytotoxic therapy, there is a rapid rise in plasma uric acid (36). Uric acid is excreted by the kidneys through the processes of glomerular filtration, partial proximal tubular reabsorption, and distal tubular secretion (35). The clearance of uric acid is independently proportional to intravascular volume status (34) and the urinary flow rate (35), and may be significantly reduced in the presence of dehydration or tubular obstruction from acute nephrocalcinosis or uric acid nephropathy. Uric acid nephropathy develops when uric acid crystals deposit in the renal tubules and collecting ducts under acidic conditions. The urinary pKa of uric acid is 5.4, and the luminal pH of the distal tubules and collecting ducts is 5.0, resulting in the poor solubility of uric acid in acidic urine (7). This poor solubility, coupled with the marked hyperuricosuria present in ATLS, leads to uric acid precipitation, intraluminal obstruction, oliguria, and ARF (7,45). ARF in ATLS may also be caused by renal calculi from phosphate and uric acid precipitation (34), as well as ischemic acute tubular necrosis caused by renal hypoperfusion (36). Drug toxicity, sepsis, and tumor-associated obstructive uropathy or renal parenchymal infiltration may exacerbate ATLS-induced ARF (42).
Diagnosis and Classification
Hande and Garrow (38) first classified ATLS into laboratory TLS and clinical TLS. Cairo and Bishop (42) have modified and further developed this classification system into the Cairo–Bishop definition, which uses laboratory and clinical data in conjunction with a grading scale to assess the severity of ATLS. Laboratory TLS (LTLS) is defined as two or more of the following metabolic abnormalities occurring 3 days before or 7 days after chemotherapy: uric acid 8 mg/dL or greater, potassium 6 mEq/dL or greater, phosphorous 6.5 mg/dL or greater, or a 25% increase in baseline levels of these metabolites, and calcium up to 7 mg/dL, or a 25% decrease from baseline level. Clinical tumor lysis syndrome is defined as LTLS in addition to one or more of the following findings: serum creatinine at least 1.5 times the upper limit of normal, cardiac dysrhythmia/sudden death, or seizure. The grading of ATLS from 0 through 5 is determined by the presence or absence of LTLS, the degree of serum creatinine elevation, and the presence and severity of the cardiac arrhythmia and seizure (42).
Prevention and Treatment
Early recognition of patients at high risk for ATLS is an essential component of the management strategy so that appropriate prophylactic interventions can be instituted.
Fluids and Alkalinization
Except in patients at risk for CHF, aggressive intravenous hydration with isotonic or hypotonic saline (46) is the single most important intervention for both prevention and treatment of ATLS. Cytotoxic therapy should be delayed whenever possible to administer appropriate hydration (7,46). Intravenous hydration should commence 2 days before and for 2 to 3 days after chemotherapy (34,36) at a rate of 2,000 to 3,000 mL/m2/day (7,9,38,46,47), or two to four times the daily fluid maintenance requirement to achieve a urine output of greater than or equal to 80 to 100 mL/m2/hr (30,38,46). Aggressive administration of intravenous fluid increases the intravascular volume, renal blood flow, glomerular filtration rate, and urinary flow rate, resulting in correction of electrolyte derangements by dilution of the extracellular fluid and prevention of phosphate and uric acid precipitation by increasing urinary excretion of these metabolites (48). Volume expansion alone may be insufficient to maintain adequate urine output, necessitating the administration of diuretics. Once euvolemia is achieved, and no signs of acute obstructive uropathy are present, a dose of furosemide (0.5 to 1 mg/kg or 2 to 4 mg/kg for severe oliguria or anuria, respectively) may induce or improve urine output (34). The effectiveness of furosemide is diminished in the setting of uric acid precipitation in the renal tubules; in this circumstance, mannitol, at a dose of 0.5 mg/kg, may be administered.
Alkalinization of the urine to a pH of 7.0 has fallen out of favor (7,9,46). This practice is based on the biochemical properties of uric acid, that is, uric acid is 13 times more soluble at pH 7.0 than at pH 5.0 (35), maximal solubility of uric acid is attained at pH 7.5, and urine alkalinization (pH ≥6.5) enhances renal excretion of uric acid (42). What limits this approach is that calcium phosphate precipitation increases with systemic alkalinization, exacerbating nephrocalcinosis (7,34). Additionally, hypoxanthine and xanthine solubility are substantially reduced, leading to xanthine nephropathy with concurrent allopurinol therapy (9,34,40). At this time, use of sodium bicarbonate can only be recommended in patients with metabolic acidosis and high levels of uric acid. It should be discontinued when serum phosphate levels begin to rise (47).
Management of Hyperuricemia
Allopurinol reduces the risk of ATLS when administered 2 to 3 days prior to chemotherapy by inhibiting the production of uric acid (9). Allopurinol is both a synthetic structural analog of the purine base, hypoxanthine, and a competitive inhibitor of xanthine oxidase (36), and, therefore, in the presence of allopurinol, xanthine oxidase cannot catalyze the conversion of hypoxanthine to xanthine and xanthine to uric acid (34). Allopurinol is administered orally at 300 to 800 mg daily (10 mg/kg/day or up to 400 mg/m2/day) in one to three divided doses, and should be titrated to uric acid level. Intravenous allopurinol can be administered in doses of 200 to 400 mg/m2/day, to a maximum of 600 mg/day, in patients unable to tolerate oral medications (7,49). Dose adjustment of allopurinol is required for reduced creatinine clearance (7,49). There are several limitations with allopurinol therapy:
- A reduction in serum uric acid level is not seen before 48 to 72 hours after initiating allopurinol because the drug inhibits the synthesis of uric acid but does not affect the pretreatment uric acid concentration (7).
- Inhibition of xanthine oxidase by allopurinol leads to increased plasma levels of xanthine and hypoxanthine, which may precipitate in the renal tubules (36).
- Three percent of patients develop hypersensitivity reactions, including Stevens–Johnson syndrome.
- Allopurinol interacts with many drugs, including chemotherapeutic agents such as cyclosporine and azathioprine (45).
Another agent that lowers uric acid concentration is urate oxidase (48). Urate oxidase converts uric acid to allantoin, which is five to ten times more soluble in urine than uric acid. Rasburicase, a recombinant urate oxidase, normalizes uric acid levels within 4 hours of administration in children and adults at doses of 0.15 to 0.20 mg/kg (7,45). This dose may be repeated daily for a total of 5 days, and chemotherapy should be initiated 4 to 24 hours after the first dose. In addition to being more effective than allopurinol in reducing pretreatment and posttreatment uric acid levels, rasburicase does not generate increased xanthine and hypoxanthine levels, thereby minimizing the risk of uric acid nephropathy that may be seen with allopurinol use (7,34,45). Of note, rasburicase is contraindicated in patients with glucose-6-phosphate dehydrogenase (G6PD) deficiency; additionally, bronchospasm and anaphylaxis may rarely occur with rasburicase therapy (50). There is insufficient evidence that rasburicase reduces the incidence of dialysis in ATLS, and because a 5-day course of therapy is considerably more expensive than a 5-day course of oral allopurinol (7,42), cost-effectiveness must be considered in formulating a treatment plan.
Correction of Electrolyte Abnormalities
Because of the potential for life-threatening dysrhythmias, prompt recognition of electrolyte derangements is imperative. Laboratory monitoring should be performed every 4 to 6 hours in the first 24 hours of chemotherapy in patients at high risk for ATLS, and then every 6 to 8 hours thereafter. A baseline electrocardiogram (ECG) should be obtained to assess for cardiac effects related to electrolyte abnormalities. Hyperkalemia is treated with calcium gluconate to stabilize the cardiac membrane and with intravenous insulin/dextrose and inhaled beta2 agonists to facilitate intracellular shift of potassium. Although sodium bicarbonate may also shift potassium intracellularly by improving the metabolic acidosis, its use may result in inappropriate volume expansion. Potassium-binding resins such as sodium polystyrene sulfate increase potassium elimination in the GI tract and have a delayed hypokalemic effect. Diuretics can be administered to reduce serum potassium in patients without renal failure. When ARF occurs, dialysis may be required to emergently reduce serum potassium. Asymptomatic hypocalcemia should be left untreated to preclude calcium phosphate precipitation; however, symptomatic hypocalcemia is managed with intravenous calcium gluconate. Treatment of hyperphosphatemia with oral phosphate binders such as aluminum hydroxide or aluminum carbonate will usually concurrently correct the hypocalcemia (7,34).
Dialysis
Dialysis is indicated in patients with marked elevations in serum uric acid, phosphate, and potassium that do not respond to aggressive treatment, and in patients with ARF with volume overload, severe uremia, or acidosis (33,36,42,46). Hemodialysis is used in ATLS because it is superior to peritoneal dialysis in the clearance of both uric acid and phosphorous (34,42).
Controversies
- Administration of urine alkalinizing agent such as sodium bicarbonate or acetazolamide can only be recommended in patients with metabolic acidosis and high levels of uric acid. It should be discontinued when serum phosphate levels begin to rise (47).
- The preferred regimen for lowering serum uric oxide remains unclear. Although rasburicase is a more effective hypouricemic agent than allopurinol, it is unknown if its use results in improved clinical outcomes such as reduced AKI and mortality.
SUPERIOR VENA CAVA SYNDROME
Superior vena cava syndrome (SVCS) describes the set of signs and symptoms associated with obstruction of the superior vena cava (SVC), which may be caused by extrinsic compression, vascular invasion, or intraluminal thrombosis of the vein (51–53). The SVC is a thin-walled, compliant, low-pressure middle mediastinal vessel, rendering it easily vulnerable to disease processes in the adjacent right lung, the paratracheal and perihilar lymph nodes, the mainstem bronchi, the esophagus, and the thoracic spinal cord (53,54).
First described by Hunter (55) in 1757 in a patient with an aortic aneurysm secondary to syphilis, SVCS was—prior to the widespread use of antibiotics—primarily a complication of infectious diseases, as seen in syphilitic aortitis, histoplasmosis-induced fibrosing mediastinitis, and tuberculous mediastinitis (56,57). Currently, malignancy is the most common cause of SVCS accounting for approximately 60% to 85% of cases (57,58,59). Benign etiologies of SVCS account for the remaining 15% to 40% and appear to be increasing due to the increasing use of intravascular devices, such as central venous catheters and pacemaker wires (71%) (57). Other nonmalignant causes of SVCS include fibrosing mediastinitis from prior irradiation or histoplasmosis, aortic dissection, and complications of surgery, such as aortic dissection repair (57,58,60,61). Bronchogenic carcinoma accounts for 85% to 90% of the malignancies in which SVCS presents (61–64). Overall, SVCS develops in 2% to 10% of lung malignancies (46,51,55,58,60), and the risk of SVCS is higher in small cell lung cancer, with an incidence of 6.6% to 12% (65) because it involves the central mediastinal structures. In addition, because of the anatomic location of the SVC, right-sided lung cancers cause SVCS four times as often as left-sided lung cancers (63). Other neoplasms causing SVCS include malignant lymphomas; although HL more often involves the mediastinum, it rarely causes SVCS (61,64). Primary germ cell cancers, thymoma, mesothelioma (66), and metastatic disease (primarily breast carcinoma) constitute a small proportion of SVCS cases (57,61,66).
Pathophysiology
The severity of signs and symptoms depends on the extent, location, and rapidity of onset of the SVC occlusion (62). In general, obstruction within or below the azygos vein results in more pronounced symptoms. Normally, azygos venous capacity increases from 11% to 35% to augment drainage of the head and neck (52), but impedance of flow from obstruction precludes this auxiliary function (52,61,66). With slowly developing SVCS, collateral vessels in the chest wall and upper extremities are recruited as a diversion for the existing SVC engorgement; hence, SVCS in this population is of insidious onset, as in fibrosing mediastinitis (62). The most commonly reported symptom in SVCS is dyspnea followed by head and facial swelling (53,57). Other cardiopulmonary symptoms include cough, orthopnea, and chest pain. Associated signs are neck and arm vein distention, plethora or cyanosis of the head and neck (53), venous collateralization in the arms and upper chest wall (61), and chronic pleural effusions (61,62). More extensive airway or vascular obstruction is predicted when positional maneuvers such as lying supine or leaning forward exacerbate respiratory or cardiac symptoms; for example, respiratory insufficiency in the supine position worsens as the weight of the mediastinal structures impinges on the tracheobronchial tree. In the substantially compromised patient with SVCS, cardiopulmonary arrest may ensue simply with the administration of sedatives and general anesthesia (61). Other head and neck signs and symptoms range from conjunctival and periorbital edema, nasal congestion, dysphagia, and hoarseness due to laryngeal nerve compression (67) to proptosis, glossal edema, stridor secondary to laryngeal edema, and tracheal obstruction (61,62). Patients with central nervous system (CNS) manifestations may exhibit mild headaches, dizziness, and lethargy with progression to syncope in rapidly developing or complete SVC obstruction, seizures, or coma from cerebral edema and increased intracranial pressure (52,61). Bleeding complications such as epistaxis, hemoptysis, and GI hemorrhage from esophageal varices in long-standing SVC may occur (61).
Diagnosis
Once the clinical diagnosis of SVC syndrome is suspected, confirmation can be obtained using radiologic techniques. Chest radiography reveals widening of the superior mediastinum in approximately 60% of patients (60,61,63) and pleural effusions, most frequently right-sided, in up to 25% of patients (53,61). A normal chest radiograph, however, does not exclude the diagnosis. Contrast-enhanced helical computed tomography (CT) accurately delineates the site, extent, and cause of the occlusion (63,66), as well as any associated thrombus and collateral vessel development (66). The radiologic diagnosis of SVCS is made by demonstrating both decreased or absent venous opacification below the level of obstruction and prominent collateral vessel opacification (63). MRI is an alternative imaging method in patients with iodinated contrast allergy or without adequate venous access for contrast administration, but offers no distinct advantage over CT (52,61,68). Venography is most useful when planning bypass or stenting procedures (52,66). Although venography is superior to CT in identifying the site and extent of obstruction and in mapping the collateral circulation, it does not elucidate the underlying cause of the SVCS (68), unless SVC thrombosis alone is the causative factor (58,63,66).
Treatment
The primary goals of treatment are symptom relief and eradication or palliation of the underlying malignancy. Initial symptomatic management involves bed rest, head elevation to reduce venous pressure, and supplemental oxygen administration. Diuretics and sodium restriction may decrease edema, but reports are anecdotal. Use of glucocorticoids to minimize inflammatory responses to tumor or radiotherapy (XRT) is controversial (64), but steroids are a mainstay of treatment in NHL (61,63). Urgency of treatment is guided by the severity of symptoms (58,69–72). The American College of Chest Physicians (ACCP) and the National Comprehensive Cancer Network (NCCN) recommend expedited stent placement or radiotherapy for patients with symptomatic SVCS (73,74). Patients with evidence of cerebral or laryngeal edema or those with hemodynamic compromise require an emergent intervention.
Endovascular Stenting
If conservative measures are ineffectual in controlling symptoms, a percutaneously placed endovascular stent can be inserted with or without balloon angioplasty (63,75–77). Prior series note relief of symptoms immediately after stent placement in over 90% of patients with few complications (78,79). Recurrence of SVCS occurs in up to 40% of patients after primary stenting (average 13%) and is usually successfully treated with a second interventional procedure. Complications of stent placement occur in up to 7% of patients and include infection, bleeding, pulmonary embolus, stent migration, and SVC perforation (79–81).
Thrombolysis
With the increased use of intravascular devices, thrombus now accounts for a larger proportion of the benign causes of SVCS (57). When SVC syndrome is attributable to thrombosis of a central venous catheter, and catheter preservation is desired, thrombolytic therapy given within 5 days of symptom onset is associated with an 88% success rate versus 25% after 5 days (63,82). Patients with extensive thrombosis in the context of SVCS may benefit from catheter-directed thrombolysis (83).
Radiotherapy and Chemotherapy
The treatment modality selected should be individualized to the type of malignancy, stage, and performance status of each patient (66). Primary management of radiosensitive tumors such as NSCLC involves XRT. NSCLC associated with SVCS carries a poor prognosis, with 1-year survival, in one series, of 17% (84). The treatment of choice in NSCLC is XRT and, when possible, stent insertion (73). Within 72 hours of XRT, patients have relief of symptoms, and within 2 weeks, 70% to 90% of patients are symptom free (63). In a large systematic review, 60% of the NSCLC patients had relief of SVCS after chemotherapy and/or radiotherapy, and SVCS recurred in 19% (66).
Chemotherapy prolongs survival and improves quality of life in patients with small cell lung cancer (SCLC), and addition of thoracic irradiation may reduce the recurrence risk of SVCS. In the aforementioned systematic review, SVCS was relieved in 77% of patients receiving chemotherapy and/or radiation, with relapse in 17% of patients (66). Lymphoma and germ cell tumors are usually treated with chemotherapy based on the histologic type, grade, and stage of the disease. In HL, chemotherapy followed by XRT to areas of bulky disease may be indicated (63). In NHL, XRT alone may be used in early-stage disease, and chemotherapy is the treatment for higher-stage tumors. Whether to irradiate areas of bulky disease in NHL after chemotherapeutic remission is less clear; however, with residual tumor or progression of disease after chemotherapy, radiotherapy is administered (63).
Surgery
Surgical bypass of the obstruction with vein grafts or prosthetic grafts may be appropriate in patients with benign causes of SVCS. In patients with malignancy, surgical intervention, when no further treatment options are possible, at best, is a palliative measure with poor long-term survival (62,63,84).
Controversies
The need for long-term anticoagulation following SVC stent placement remains unclear. Given the poor prognosis of many of these patients, there is little data to guide choice of anticoagulant and duration of therapy. Most practitioners favor a short course (4 to 12 weeks) of dual antiplatelet therapy. Anticoagulation with low–molecular-weight heparin is indicated when SVC occurs in the presence of an upper extremity DVT.
ACUTE AIRWAY OBSTRUCTION
Oropharyngeal and Tracheal Obstruction
Sudden upper airway obstruction (UAO) of the larynx, pharynx, or extrathoracic trachea is uncommon with cancers of the head and neck. Tumors of the larynx, pharynx, base of tongue, and thyroid are primarily slow growing and, as they progressively enlarge, obvious signs and symptoms of airway compromise are usually evident prior to the development of acute obstruction (85); tracheal masses, which take years to be discovered, first become symptomatic when the airway lumen is narrowed by 75% (86). Mechanisms of UAO include direct tracheal invasion as well as extrinsic tracheal compression (87).
In the head and neck, direct tracheal invasion is seen with locally advanced oropharyngeal tumors, laryngeal neoplasms associated with bulky or supraglottic lesions, and rarely, thyroid cancer and primary tracheal tumors (85). In thyroid cancer, tracheal invasion develops in 1% to 6.5% of patients, and UAO is the most common cause of death in this group (88). Direct tumor extension into the trachea from adjacent structures by malignancies of the lung, esophagus, and mediastinum occurs more frequently than metastatic disease spread (89).
Tracheal impingement in lung cancer occurs when there is tracheal ingrowth of the primary tumor originating in a mainstem bronchus or from enlarging paratracheal or subcarinal lymph nodes. Bilateral vocal cord paralysis with recurrent laryngeal nerve paralysis may also be associated with lung malignancies (85,90). Extrathoracic malignancies may metastasize to mediastinal and endobronchial lymph nodes, causing airway obstruction. Renal cell carcinoma, sarcomas, breast cancer, and colon cancer are most commonly involved (91). Melanoma may arise as a primary tracheal tumor but more often is a metastatic lesion (89).
Tracheal compression, which is usually attributable to benign disease, is a secondary mechanism of UAO in neoplastic disease and is often the initial presentation of mediastinal tumors and extensive lymphoma (92).
Pathophysiology
Patients may present with dysphagia, hoarseness, intractable cough, hemoptysis, dyspnea, or stridor (85). Important goals during physical examination are to determine whether impending airway obstruction is present and to localize the site of the lesion. Once stridor is apparent, the airway caliber has narrowed to approximately 6 mm and, without intervention, complete UAO is imminent. Inspiratory stridor implies an extrathoracic lesion at the level of the glottis or above, whereas expiratory stridor suggests an intrathoracic lesion. Biphasic stridor may be indicative of a subglottic or tracheal mass. Voice alteration, such as muffling and hoarseness, accompanies subglottic lesions and unilateral vocal cord paralysis, respectively (52).
Diagnosis
A chest radiograph may identify an obstructive neck mass and consequent tracheal deviation. Flexible oropharyngeal or nasopharyngeal endoscopy can be performed to assess the airway. Once the airway is stabilized, high-resolution CT of the head and neck provides comprehensive evaluation of the sites of narrowing and the size and extent of the tumor in relation to adjacent structures. Spirometry demonstrates a plateau in the inspiratory limb of the flow–volume loop if there is a fixed obstructive lesion in the extrathoracic trachea (93).
Treatment
Initial management includes head elevation and administration of cool humidified oxygen. Case reports have demonstrated that inhalation of a helium–oxygen mixture, consequent to its lower density compared to oxygen supplementation alone, reduces the work of breathing (61,94,95). Airway obstruction in patients with bulky oropharyngeal, laryngeal, or thyroid carcinomas will require emergent or elective tracheostomy. Endotracheal intubation is not recommended for patients with bulky, friable, laryngeal, and/or pharyngeal disease, as it may exacerbate existing airway edema and hemorrhage (86). For intraluminal tracheal lesions, bronchoscopy with interventions such as laser therapy (96,97), brachytherapy, photodynamic therapy, or stenting may be performed to rapidly alleviate symptoms (97). Stents are also useful in palliating symptomatic extrinsic compression (98). Endotracheal intubation or stenting may be used to maintain the airway when there is extrinsic compression from lymphoma (98) or other highly radiosensitive or chemosensitive tumors with anticipation of rapid reduction of tumor mass. Surgical resection is indicated for primary airway tumors (99) and for lung cancers without mediastinal lymph node involvement. In lung and thyroid cancers that directly invade the trachea, surgery may be curative (89); metastatic disease to the trachea requires palliative treatment.
Intrathoracic Obstruction
Pathophysiology
Intrathoracic airway obstruction may be present with intrinsic primary endobronchial tumors such as bronchogenic carcinoma and carcinoid, with metastatic tumors or their associated lymphadenopathy—lung, renal, breast, thyroid, and colon cancers, and sarcoma or melanoma—or with bulky disease causing airway compression. Symptoms often progress slowly over time, and patients may complain of dyspnea, wheezing, or chest discomfort, leading to the misdiagnosis of asthma or bronchitis prior to the development of fulminant airway obstruction (100); postobstructive pneumonia may be a finding on initial presentation. With impending obstruction, patients may exhibit hypertension, tachycardia, tachypnea, and significant pulsus paradoxus. Poor air movement, use of accessory muscles, and mental status changes are indicators of severe obstruction. Progressive symptoms may result in negative pressure pulmonary edema and anoxic brain injury (93). Chest examination may reveal a prolonged expiratory time and wheezing.
Diagnosis
Respiratory symptoms are unilateral with lesions below the carina (100), and the chest radiograph reveals asymmetric lung fields, particularly on end-expiration. Stable patients should have a flow–volume loop performed. An intrathoracic, mobile tracheal lesion above the carina will demonstrate airway compression during the expiratory phase, producing flattening of the expiratory limb of the flow–volume loop, whereas a plateau in both inspiratory and expiratory limbs will be observed with fixed obstructive lesions (93). Chest CT defines tumor extent and location, but rigid bronchoscopy is usually necessary to evaluate the airway in impending obstruction and those in whom significant bleeding is likely. When airway obstruction is severe, flexible bronchoscopy is hazardous because this technique does not permit ventilatory support, and, additionally, the bronchoscope may obstruct the already narrowed airway lumen (100).
Treatment
Treatment proceeds with the general measures of oxygen or helium/oxygen supplementation and, possibly, steroids. If endotracheal intubation is required, the clinician must recognize the potential for hemodynamic compromise associated with asymmetric obstruction and significant increases in airway pressure distal to the obstruction (93). Bronchoscopy with various interventions, including debridement, dilation, endotracheal stent placement, laser ablation, photodynamic therapy, and placement of brachytherapy catheters may relieve symptoms (100); external beam radiotherapy may also play a role. In lung cancer, tracheal and carinal resection is indicated in patients without mediastinal lymph node involvement for a potential cure (61).
SPINAL CORD COMPRESSION
Malignant spinal cord compression (MSCC) is a profoundly debilitating, but usually nonfatal, manifestation of metastatic cancer, occurring in 5% to 10% of cancer patients (102–105). The term, MSCC, refers to epidural, intramedullary, and leptomeningeal disease; however, the focus of this section is on epidural spinal cord compression (ESCC) because the literature primarily discusses this population (103). Although any malignancy capable of metastatic spread may give rise to MSCC, prostate, breast, and lung cancers are most commonly involved, with each accounting for 15% to 20% of cases (101,104) or, in combination, 60% of cases (103,105). The cumulative incidence of MSCC is specific to tumor type, with the highest rates occurring in multiple myeloma (8%), prostate cancer (7%), and nasopharyngeal cancer (6.5%) (105). Other tumors include NHL and renal cell carcinoma, with each representing 5% to 10% of cases (106), and GI cancers, sarcoma, melanoma, thyroid cancer (92,93), and unknown primary carcinoma (105,106). Enlarging meningiomas, nerve sheath tumors, and leptomeningeal metastases may also compress the spinal cord. Nonmalignant causes of MSCC in the cancer patient are epidural abscesses in the presence of immune compromise and hematoma with bleeding diatheses (107).
MSCC has a proclivity for the thoracic spine (102,106,108–110) and is estimated to occur in this location in approximately 60% to 66% of cases (107,109). Twenty percent of cases involve the lumbar spine (102,107); MSCC in the cervical spine is uncommon, seen in 7% to 10% of cases (109,110). Prostate and colorectal carcinomas favor the lumbosacral spine (107); MSCC is the initial manifestation of malignancy in 20% of patients.
Pathophysiology
The mechanisms by which MSCC occurs include vertebral body invasion by tumor with possible vertebral collapse causing encroachment on the anterior spinal cord (85%); direct extension into the intervertebral space by paraspinal lymphoma, sarcoma, or lung cancer, seen in 10% to 15% of cases; and epidural or intramedullary space invasion, seen in less than 5% of cases (102). The mechanism of injury to the spinal cord is mediated by white matter vasogenic edema and axonal swelling that result from cord compression. Venous hypertension, decreased spinal cord blood flow, and cord infarction ensue, resulting in ischemic hypoxic neuronal injury.
Pain, which may be characterized as localized, radicular, or referred, is the primary presenting symptom in MSCC, occurring in 83% to 95% (106,108,111) of patients for a median of 8 weeks prior to diagnosis (106,108). Focal bony pain is typically localized, dull or aching, and constant. Direct tenderness of the involved vertebral body may be evident with periosteal destruction (112). With time, radicular pain occurs in the dermatome of the affected nerve root and is severe, deep, and lancinating. Radicular symptoms occur most often in the lumbosacral spine and may be unilateral or bilateral, the latter more frequent with thoracic spine involvement (107,113). Referred pain does not radiate, but appears in a region distal to the area of pathology; for example, sacroiliac pain may result from L1 compression (113). The pain of MSCC is typified by worsening with recumbency secondary to distention of the epidural venous plexus (106,107); coughing, sneezing, or Valsalva maneuvers will also exacerbate the pain (102). Straight-leg raising identifies a lumbosacral radiculopathy, and neck flexion reproduces symptoms of thoracic radiculopathy (107,111,113).
Motor weakness is present in 60% to 85% of patients on diagnosis of MSCC. Although only one-third of patients complain of lower extremity weakness on initial presentation (107), two-thirds are not ambulatory at the time of diagnosis (106,107). Motor deficits at the level of the conus medullaris or above generally have a symmetric distribution. Paresis is usually seen in the extensors of the upper extremities or the flexors of the lower extremities, depending on the location of the lesion in the spine. Upper motor neuron signs such as spasticity, hyperreflexia, and Babinski responses, may be present. Cervical lesions may lead to quadriplegia and respiratory collapse (112).
Sensory deficits, reported as varying degrees of paresthesias, are less common than motor deficits but can still be found in 40% to 90% of patients. The level of hyperesthesia on examination occurs one to five levels below the actual anatomic level of cord compression (106). The sensation of an electric shock radiating through the spine and extremities with neck flexion, termed the Lhermitte sign, is seen infrequently with cervical or thoracic neoplasms. Perineal paresthesias may occur with cauda equina lesions. Gait ataxia may follow sensory loss impairment, but in the absence of sensory findings, impairment of the spinocerebellar tract should be considered.
Bowel and bladder dysfunction reflects autonomic dysfunction and is a late manifestation of MSCC (113). Patients report urinary hesitancy and frequency, and both incontinence of urine, from poor sphincter tone or overflow of urine, and urinary retention may ensue. At the time of diagnosis, 50% of patients are incontinent or catheter-dependent (114); patients may also exhibit erectile dysfunction and impotence. Constipation and incontinence of stool with diminished sphincter tone may be present (113).
Diagnosis
The imaging study of choice in evaluating MSCC is magnetic resonance imaging (MRI); this noninvasive test provides high resolution of the soft tissues, including bony metastases and intramedullary pathology. One study found that MRI had a sensitivity, specificity, and overall accuracy of 93%, 97%, and 95%, respectively, in detecting MSCC in patients with known malignancies, excluding primary CNS tumors (114). When the entire spine is imaged beyond the area of clinically determined cord compression, multiple epidural metastases (MEMs) are found in 30% of patients. Because the presence of MEMs may alter treatment strategy, several studies have purported using whole-spine MRI in all patients undergoing imaging (104,109,114,115). Myelography, with or without CT myelogram, is a more invasive tool than MRI and is used in imaging MSCC when MRI is contraindicated. CT alone does not adequately define the soft tissues and spinal cord, and plain radiographs and radionuclide testing have low sensitivity and specificity for demonstrating MSCC. Plain films detect vertebral metastases at the site of known cord compression only 80% of the time, and many metastases are missed because the ability to visualize these lesions requires that 30% to 40% of the bone be eroded (116).
Delay in diagnosis may be attributed to the patient’s failure to identify symptoms and diagnostic delays by the generalist and hospital practitioner, leading to potentially avoidable deterioration in motor or bladder function (117).
Treatment
The goals of therapy are pain control and preservation of neurologic function. Treatment options include narcotics, corticosteroids, radiation, and surgery. Pretreatment neurologic function is the most important predictor of posttreatment outcome (118,119).
Corticosteroids
In a randomized trial that established the efficacy of corticosteroids in cord compression, patients were assigned to XRT with or without dexamethasone. At the conclusion of the study, 81% of those receiving corticosteroids and XRT versus 63% of those receiving XRT alone remained ambulatory. At 6 months, the percentages were 59% and 33% in the two groups, respectively (120). There are less well-established data regarding the use of high-dose dexamethasone regimens because, although higher doses (100-mg vs. 10-mg boluses) may have greater clinical efficacy in improving posttreatment ambulation, they are associated with a higher proportion of adverse effects. Typical regimens include a 10-mg bolus, followed by 16 mg divided four times daily, tapered over 2 weeks. High-dose regimens (100-mg bolus, then 96 mg divided four times daily, tapered over 2 weeks) (103) may be reserved for patients with paresis or paraplegia. In ambulatory patients who are asymptomatic and undergoing XRT, corticosteroids may be withheld (103,104,121).
Surgery and Radiation
Definitive management of MSCC includes decompressive surgery and/or radiotherapy. Individuals with mechanical instability of the spine and a favorable overall prognosis should proceed directly to surgery (122). Vertebroplasty or kyphoplasty is an option for patients who are not suitable candidates for spine surgery. The same is true of individuals with stable spine lesions that are not radiosensitive. Patients with mechanically stable MSCC should proceed with radiotherapy. This may be administered via external beam radiation therapy (EBRT) or stereotactic radiosurgery (SRS). Occasionally, embolization of vascular tumors may be performed preoperatively.
Controversies
The role of high-dose steroids remains unclear. As noted above, only one of three studies demonstrated improved motor function at higher doses of dexamethasone (with an increased incidence of side effects). Current practice favors the use of high-dose steroids only for patients with severe myelopathy upon presentation.
CARDIAC TAMPONADE
Primary neoplasms of the myocardium and pericardium are uncommon, but metastatic disease to the pericardial space is frequently seen (123). Primary pericardial tumors, of which mesothelioma represents the largest proportion, are 40 times less common than metastatic disease. Secondary malignancies include, most frequently, lung, breast, ovarian carcinomas, and melanoma, lymphoma, and leukemia (124). Malignancy is a primary cause of pericardial effusion in the United States (125), and pericardial tamponade resulting from malignant pericardial effusion (MPCE) represents at least 50% of reported cases of pericardial fluid collection requiring intervention (126,127). Autopsy series have reported, with varying estimates, that MPCE is seen in 2% to 22% of cancer patients (125,126,128), and that these effusions are clinically quiescent, remaining unrecognized (52). In some patients, MPCE may be the initial presentation of cancer, but in any patient, it signifies a dismal prognosis, with most patients dying within 1 year (129). Pericardial effusions in some cancer patients may be attributable to comorbid conditions rather than to malignant disease, and other causes must be considered, such as radiation-induced pericarditis, infection, uremia, myocardial infarction, CHF, and pneumonia (124).
Pathophysiology
The pericardium is a fibroserous sac, composed of two layers that surround the heart. The outer layer is the fibrous pericardium, which attaches to the diaphragm and securely anchors the heart within the thoracic cavity. The serous pericardium is a single layer of mesothelial cells and its underlying connective tissue, which lines the fibrous pericardium. During embryonic development, the heart invaginates the walls of the serous pericardium, creating a potential space between an inner serous layer that is adherent to the heart (visceral pericardium) and an outer serous layer that lines the fibrous pericardium (parietal pericardium). The pericardial space is formed between the two serous layers, and it normally contains 15 to 50 mL of fluid for lubrication. The fluid is drained from the right pleural space into the right lymphatic duct, and from the parietal pericardium into the thoracic duct (130,131); any interruption in this flow will result in accumulation of fluid and pericardial effusion. The mechanisms by which malignant disease generates MPCEs include direct invasion of the pericardium or myocardium, and disruption of lymphatic flow from lymph node metastases or from prior radiotherapy to the chest or mediastinum (126,128). The tumors that invade the pericardium directly or hematogenously are most often lung cancer, followed by lymphoma and breast cancer (52,126).
With either of the aforementioned mechanisms, pericardial fluid accumulates and inhibits passive diastolic filling of the normally low-pressure right heart structures, producing jugular and abdominal venous hypertension (126). As the pericardial effusion expands, the heart is further compressed, leading to reduced diastolic compliance, decreased diastolic filling, and, ultimately, decreased stroke volume, cardiac output, and blood pressure. Right atrial and right ventricular collapse ensues, resulting in frank tamponade, which, untreated, will lead to shock (133). Pericardial reserve volume, defined as the volume that will just distend the pericardium, is approximately 10 to 20 mL. As the pericardial effusion enlarges, capacity for stretch is exceeded. Therefore, when fluid accumulates rapidly, the pericardium cannot stretch rapidly enough to accommodate the added volume, and the heart becomes compressed (131). Under these circumstances, acute tamponade may occur with as little as 50 mL of fluid (132). When effusions develop chronically, the pericardium is able to compensate by stretching slowly over time—the phenomenon of stretch relaxation (131–133). In cancer patients, the MPCE develops slowly, and as much as 2 L of pericardial fluid may be present before critical symptoms occur (132).
Patients may be asymptomatic with small pericardial effusions (9,124) and, in general, symptoms correlate with the compressive effect of the effusion on surrounding structures, including the lung, trachea, and esophagus. Symptoms include dyspnea, cough, chest pain, hoarseness, hiccups, and dysphagia (131). The most commonly reported physical sign is distension of the jugular veins. The classic finding of the Beck triad of hypotension, increased jugular venous pressure, and quiet heart sounds may be present, in addition to the Kussmaul sign, which is paradoxical jugular venous distention and increased jugular venous pressure on inspiration. Sinus tachycardia, hepatomegaly, and peripheral edema may all be apparent. On cardiac examination, dullness beyond the apical impulse and râles can be detected, and in patients with inflammatory effusions, a pericardial rub is often heard. A narrow pulse pressure is frequently noted, and pulsus paradoxus, a decrease in systolic blood pressure greater than 10 mmHg, is observed in 77% of patients with acute tamponade; patients may report a feeling of uneasiness (132). When low-output shock results from failure of compensatory mechanisms to maintain cardiac output, the patient exhibits cold, clammy skin, cyanosis, oliguria, and altered mental status (133).
Diagnosis
Chest radiograph reveals a water bottle–shaped heart with widening of the cardiac silhouette and, occasionally, pericardial calcifications (132); pleural effusions will be present in one-third of cases (126). The ECG may demonstrate a low-voltage QRS or nonspecific ST-T wave changes (9). Electrical alternans in the P wave and QRS complex is a rare finding, noted in 0% to 10% of patients (131), in which every other QRS complex has a lower-voltage and/or reversed polarity (132). The echocardiogram precisely localizes the pericardial fluid, discerns the quality of the effusion (homogeneous vs. heterogeneous), determines whether loculations or bulky tumor are present, assesses right and left ventricular function, and ascertains whether right atrial and right ventricular diastolic collapse are present. On echocardiography, the heart may be seen to swing in a pendular fashion within the pericardial fluid. Right heart catheterization is the definitive standard for further defining the pericardial effusion. Classically, there will be equalization of diastolic pressures across all cardiac chambers (126).
Treatment
There are no randomized control trials to guide the management of malignant pericardial effusions. Treatment strategy should be individualized to each patient based on age, comorbid conditions, malignancy type, and overall prognosis (125). Although temporizing measures such as volume repletion and inotropic support may provide some benefit, definitive treatment requires removal of fluid from the pericardial space. The initial emergent intervention in malignant cardiac tamponade is to drain the effusion, usually with echocardiographic guidance (124). Fluid should be sent for chemical analysis, microbiology, and cytology; the effusion is removed successfully in 97% of patients (134). In the absence of tamponade, systemic chemotherapy is administered as baseline treatment (124), thereby precluding recurrences in 67% of cases (134). Systemic chemotherapy is effective in controlling malignant effusions when the tumors are chemosensitive, as in lymphoma, leukemia, and breast cancer. Notably, XRT is highly effective (93%) in controlling malignant pericardial effusions in patients with lymphoma and leukemia, although radiation myocarditis or pericarditis is, in itself, a complication of radiotherapy (134). Pericardiocentesis should be performed in MPCE, especially when these are large, for symptomatic relief and to establish a cause. Because fluid reaccumulates within 48 hours of the initial pericardiocentesis (135), intrapericardial sclerosing or cytostatic agents, selected according to tumor type, should be administered to prevent recurrence. The mechanism of action of sclerosing agents is to effect symphysis of the visceral and parietal pericardia (124). A surgical approach to MPCE management is subxiphoid pericardiotomy to create a pericardial window. An advantage of this technique is that it is performed using local anesthesia and has a low recurrence rate. Additionally, tissue can be obtained for pathologic review. However, there is a small risk of myocardial laceration, pneumothorax, and mortality with this procedure. One study showed a 12% recurrence at 1 year and a 4% reoperation rate for subxiphoid pericardiotomy (125). Pleuropericardiotomy and pericardiectomy, which require general anesthesia, have higher morbidity and mortality rates, and are rarely used in MPCE management (124). Percutaneous balloon pericardiotomy is a treatment option for patients with large recurrent neoplastic pericardial effusions. Requiring only local anesthesia, it facilitates passage of pericardial fluid into the left pleural or peritoneal spaces, which have greater resorptive capacity. The major side effect is asymptomatic pleural effusion in most patients (52,136). Percutaneous balloon pericardiotomy appears to be a safe and effective technique in patients with large MPCEs and recurrent tamponade (90 to 97%) (136,137). Reaccumulation rates with this method are 0% to 6%. Reaccumulation rates for other therapies that are administered after initial pericardiocentesis is performed are radiotherapy, 33%; systemic chemotherapy, 30%; sclerotherapy with tetracycline, 15% to 30%; and mechanical therapies, including indwelling pericardial catheter placement, balloon pericardiotomy, and thoracotomy with pericardiostomy, 0% to 15% (52).
Even if there is no reaccumulation of fluid, cardiac function may remain impaired in the presence of epicardial infiltration by tumor. Diastolic dysfunction occurs because of the constrictive effect of a diseased epicardium surrounding the heart. Effusive-constrictive pericarditis results in a combination of tamponade and cardiac restriction. This entity must be considered in the differential diagnosis when a patient develops hemodynamic collapse a few days after pericardiocentesis. Pericardiectomy may be useful in alleviating the constrictive component; irrespective of this procedure, mortality is extremely high (52,138–140).
NEUTROPENIC ENTEROCOLITIS
Neutropenic enterocolitis (NE) is also known as necrotizing enteropathy, ileocecal syndrome, or typhlitis (141), from the Greek derivation of the word “typhlon,” or cecum (142). The disorder is a life-threatening inflammatory syndrome in the immunocompromised patient that involves the terminal ileum, ascending colon, and cecum (143). Because the disease affects both the small and large bowel, the term, neutropenic enterocolitis, is most commonly used (144). The cardinal features that define the syndrome are fever, abdominal pain, and bowel wall thickening in a patient with neutropenia (143,145), where neutropenia is defined as a neutrophil count of either less than 500 neutrophils/μL, or less than 1,000 neutrophils/μL with an expected precipitous decline to below 500 neutrophils/μL (146,147). In its natural history, the disease may progress to bowel ulceration, necrosis, and perforation, and ultimately, sepsis and death (148).
NE occurs primarily in patients following aggressive cytotoxic therapy for acute leukemia (143,149) and other hematologic malignancies such as lymphoma (143,145,150), chronic leukemia (143), multiple myeloma (143–145), and rarely in solid tumors, such as colon, breast, testicular, lung (141,151), and pancreatic cancer (151). In leukemia, administration of drugs toxic to the bowel mucosa, such as cytosine arabinoside (152), which cause changes ranging from cellular atypia to frank ulceration, increases the risk of NE (151). Other agents include cytarabine, cisplatin, paclitaxel, fluorouracil, vincristine, doxorubicin, 5-fluorouracil, thioguanine, and mercaptopurine (152,153). Patients receiving immunosuppressive therapy for bone marrow (154) or renal transplantation (155) are also at risk.
The incidence of NE in adults varies widely in the literature, ranging from 0.8% to 26%. The increased use of cytotoxic chemotherapies that lead to GI tract mucosal inflammation is believed to result in an increased incidence of NEC (142,156,157). Early case reports described mortality rates ranging from 50% to 100% (158). Although no large recent case series have been published, early recognition and timely interventions described below have significantly reduced mortality from NE (159).
Pathophysiology
NE has a predilection for the terminal ileum, cecum, and appendix (160). One factor that may explain this predisposition is the overall decreased blood supply to the colon (145). Also, inherent to the cecum is decreased vascularity and increased distensibility compared to other colonic segments (151,161), and progressive distention in the cecum may cause increasing intraluminal pressure and exacerbation of submucosal edema (151). The pathogenesis of NE is multifactorial and remains unclear (141,145). Drug-induced cytotoxic mucosal injury (141,142,151) initiates the process by limiting cellular proliferation and generating glandular epithelial atypia and necrosis (cytosine arabinoside), and by producing myenteric plexus degeneration (vincristine) (151). Subsequently, mucosal barrier integrity is breached because cells cannot rapidly regenerate to repair the damaged surface (151). Once mucosal damage develops, bacterial translocation occurs, resulting in microbial infection and sepsis (141,151). Marked neutropenia impairs host defense and promotes further microbial invasion; bowel flora becomes altered (145). Blood cultures are often positive for Clostridium septicum, C. difficile, Escherichia coli, Pseudomonas, Klebsiella, Enterobacter, and Staphylococcus (144,151). Candidiasis, primarily Candida albicans, which colonizes mucosal surfaces, is the most common fungal infection in neutropenic patients and is associated with a high morbidity and mortality (162). These microbial infections lead to inflammation and edema. With sustained profound neutropenia, bacterial invasion is unconstrained, resulting in transmural necrosis, hemorrhage, ulceration, and perforation (144,145,151,163). In addition to drug-induced mucosal injury, infiltration of mucosa with leukemic and lymphoproliferative cells and mucosal ischemia from sepsis-related hypotension may also participate in initiating and perpetuating mucosal injury (151,160).
Diagnosis
The onset of NE is 7 to 10 days after treatment when neutropenia is evident. The clinical presentation includes fever, occurring in 90% of all hospitalized neutropenic patients at any time (163), nausea and vomiting, abdominal pain, and watery or bloody diarrhea. Physical examination may reveal stomatitis with diffuse mucositis, abdominal tenderness, abdominal distention, and peritoneal signs suggestive of bowel perforation (151,152,164). In 60% to 80% of patients, right lower quadrant (RLQ) tenderness is elicited. Palpation of a mass in the RLQ usually indicates a thickened, dilated, fluid-filled cecum (151).
On laboratory analysis, in addition to neutropenia, thrombocytopenia may be seen. Blood cultures are positive in 50% to 82% of cases for bowel organisms as described above (144). Stool studies may be notable for absence of C. difficile toxin A because C. difficile is not the primary pathogen in NE (143,161).
Plain radiographs of the abdomen are usually normal or nonspecific. Findings may include a decrease in RLQ gas with dilated small bowel loops and air–fluid levels consistent with a distal bowel obstruction. Free intraperitoneal air after perforation, pneumatosis coli, or localized or diffuse “thumb-printing” characteristic of mucosal edema may be exhibited (141,151). Sonography assists in confirming the diagnosis of NE and in excluding other differential diagnoses by detecting bowel wall thickening. Additionally, ultrasound is useful in following the clinical course of the disease (145,165). Sonographic manifestations of NE include a rounded mass with dense central echoes and a wider hyperechoic periphery (141), pseudopolypoid changes of the cecal mucosa, and pericolic fluid collections (151). CT is a more accurate modality for assessing cecal wall thickening and evaluating the extent of the colitis (141,145). It also has utility in differentiating NE from appendicitis, appendiceal abscess, or pseudomembranous colitis (167). CT findings include diffuse submucosal thickening and edema of the terminal ileum and ascending colon, mural hemorrhage, pericolic fluid collections, abscess formation, pneumatosis coli, and intraperitoneal free air (145). The false-negative rates in identifying NE for CT, ultrasound, and plain radiographs are 15%, 23%, and 48%, respectively (149).
Barium enema is unsafe because it may result in bowel perforation in the presence of severely damaged, necrotic bowel (142,167). Endoscopic evaluation is generally avoided because it involves a high risk of perforation in addition to hemorrhagic and infectious complications, and it may precipitate fulminant mural necrosis (141). Colonoscopy has been performed in a small number of patients and shows irregular nodular mucosa, ulcerations, hemorrhagic friability, and a masslike lesion resembling carcinoma (142).
Management
Prospective trials or case-control studies evaluating therapeutic interventions in NE are lacking (143). Conservative management of NE is indicated for patients without overt perforation, peritonitis, or bleeding; this includes bowel rest, intravenous fluid and blood product resuscitation, broad-spectrum antibiotics, granulocyte colony-stimulating factor (G-CSF), and nasogastric decompression. Medications that inhibit bowel motility, such as antidiarrheal and narcotic agents should be avoided because they perpetuate ileus and promote bacterial overgrowth (152). Patients with chemotherapy-induced NE may suffer from repeated episodes with future treatment; therefore, further chemotherapy should be withheld until NE has completely resolved. Bowel decontamination may be helpful before subsequent chemotherapy (142). Empiric antibiotic therapy with a carbapenem, piperacillin–tazobactam, or cefepime plus metronidazole should be initiated immediately (143,151,168). Empiric antifungal therapy with voriconazole or amphotericin B therapy should be considered in profoundly neutropenic patients whose fevers persist beyond 3 days of appropriately dosed broad-spectrum antibiotics (146).
G-CSF increases cell division in myeloid precursor cells, decreases bone marrow transit time, and modulates activity and function of developing and mature neutrophils (163). As clinical improvement in NE patients is usually seen after normalization of the neutrophil count, G-CSF is often given as part of initial management of this condition; it should be noted that there are no data to support its use. Furthermore, there is a theoretical risk that bowel inflammation during immune recovery may lead to further inflammation and perforation (142).
There are no standard recommendations, but rather, general guidelines in the literature regarding surgical intervention in NE. Most patients are unlikely to be surgical candidates. Early reports recommended aggressive and early surgical resection of involved bowel, anticipating that in the natural history of NE, bowel perforation is inevitable (143). Subsequent series demonstrate successful nonsurgical management (143,169,170). More recent publications support surgery with laparotomy alone for patients with perforation and ileus (143). Some advocate that patients who fail to improve or develop bowel perforation and peritonitis after 2 or 3 days of conservative therapy warrant consideration for surgery (161,171). Several authors recommend surgery for severe complications such as abscess, necrotic bowel, and obstruction (145). In general, definitive indications for surgery include intraperitoneal free air/perforation, generalized peritonitis, and persistent bleeding in spite of correction of coagulopathy (141,151). Important considerations that must influence the decision to surgically intervene are the patient’s prognosis and comorbidities (151). If surgery is warranted, the procedure of choice is colectomy with ileostomy and mucous fistula; a primary anastomosis is used in very few patients (172). Of note, the extent of mucosal necrosis may be underestimated by the appearance of the serosa. A surgeon must ensure complete resection of edematous bowel, even in the absence of necrosis and inflammation, to preclude a fatal outcome (151).
Controversies
The management of NE is not based on prospective studies of medical versus surgical intervention. Treatment is usually individualized and based on past experience. Although it appears reasonable to treat patients with early NE conservatively, there are no data to support superior outcomes with this approach.
TOXICITY OF CHEMOTHERAPY
Most antineoplastic agents exert their therapeutic actions by targeting rapidly proliferating malignant cells. Because these agents interrupt fundamental cellular processes such as DNA, RNA, and protein synthesis, they are not completely specific to malignant cells and will also act on normal tissues, causing multiple toxicities. Rapidly regenerating cells, such as the hematopoietic lineage, GI mucosa, spermatogonia, and hair follicles may suffer transient toxicity compared to cells that have limited regenerative capacity, including those of the myocardium, and nerves (173–175). This section focuses on the major life-threatening toxicities that occur with commonly used chemotherapeutic agents. Molecularly targeted therapies and immunotherapy often have organ-specific adverse effects, which will be discussed below.
Pulmonary Toxicities
Pulmonary toxicity, both acute and chronic, is seen increasingly with numerous antineoplastic agents (176). Chemotherapy-induced lung disease describes lung injury with multiple etiologic agents and varying pathophysiologic mechanisms. These major mechanisms include direct lung toxicity, immunologic response, and increased capillary permeability. The corresponding clinical presentations are interstitial pneumonitis/fibrosis, hypersensitivity syndrome, and capillary leak syndrome, respectively, and each may eventuate in fulminant respiratory failure. Symptoms can appear immediately or months after termination of therapy (177).
Antitumor Antibiotics
Bleomycin
Bleomycin is an antitumor antibiotic used in the treatment of lymphoma, germ cell tumors, cervical carcinoma, and head and neck squamous cell carcinoma. The absence of bleomycin hydrolase in the skin and lungs prevents deactivation of the drug, accounting for its selective toxicity. Bleomycin interstitial pneumonitis is the most ominous toxicity, associated with a 3% mortality rate (178) and occurring in up to 10% of patients receiving bleomycin-containing regimens, either during treatment or up to 6 months after discontinuation (179). Toxicity is mediated by the mechanism of direct lung injury via generation of cytokines and free radicals, the sequelae of which are endothelial damage, inflammatory cell infiltration, fibroblast activation, and fibrosis (177,179). There is conflicting evidence in the literature as to whether perioperative oxygen supplementation exceeding a concentration of 24% fractional inspired oxygen causes synergistic toxicity with bleomycin through the production of free radicals (180,181); older patients and those with renal insufficiency are more susceptible. Drug withdrawal, steroids, and avoidance of supplemental oxygen may be helpful (182).
Mitomycin C
This is an antibiotic used in treating solid tumors, primarily breast and lung carcinomas. The mechanism of injury is alkylation of endothelial cell DNA, precluding cell division. This agent is associated with the development of an interstitial pneumonitis/fibrosis (184,185), usually 3 to 12 months after therapy (182,184), with a 3% to 14% incidence. Mortality is as high as 14% to 50% (183–185). Risk factors include oxygen exposure, prior irradiation, and other cytotoxic drug administration, such as bleomycin, cisplatin, the vinca alkaloids, cyclophosphamide, and doxorubicin. Drug withdrawal, steroids, and avoidance of supplemental oxygen may be helpful (182).
Alkylating Agents
Carmustine
Carmustine (BCNU) is a nitrosourea used in the management of CNS tumors and in induction therapy for bone marrow transplantation (BMT). Its cytotoxicity is mediated by alkylation of guanine in DNA (180). Carmustine causes dose-dependent pulmonary fibrosis and carries the highest incidence of fibrosis among the nitrosoureas. The mortality rate ranges from 24% to as high as 90% in some reports (182,185). Sixty percent of patients will respond dramatically to steroids (182).
Microtubule-Targeting Agents
Taxanes
Paclitaxel inhibits microtubule disassembly (186), and has activity against solid tumors such as non–small cell lung carcinoma, breast carcinoma, and ovarian carcinoma (177); it is prepared in Cremophor, a castor oil-based solution (177,186). A type I hypersensitivity reaction, characterized by urticaria, bronchospasm, angioedema, and hypotension, occurs within 2 to 10 minutes of infusion of paclitaxel (186,187) with a 3% to 10% incidence (182), and is attributable to the Cremophor vehicle rather than paclitaxel itself (177). Premedication with steroids and H1 and H2 blockers can curtail this reaction (186).
Antimetabolites
Cytosine Arabinoside
Ara-C is a substituted nucleoside antimetabolite that disrupts DNA replication and is used in the therapy of leukemia and NHL. One of its toxicities is the abrupt onset of endothelial inflammation and capillary leak syndrome (177), causing noncardiogenic pulmonary edema, acute dyspnea, and a diffuse interstitial and alveolar pattern. Management is supportive and includes oxygen, diuretics, and mechanical ventilation when needed (182).
Gemcitabine
This pyrimidine analog, structurally similar to ara-C (187), has activity against a variety of solid tumors and lymphoma. The reported incidence of lung toxicity is less than 1.4% (188). The proposed mechanism of injury involves pulmonary endothelial cell damage resulting in capillary leak syndrome (177,187). The symptoms of gemcitabine pulmonary toxicity range from mild dyspnea to fatal ARDS. Increasing age, pulmonary neoplasm, and prior radiotherapy may be contributing risk factors (177,189). Patients respond rapidly to corticosteroids (177), but fatalities do occur (177,185,187,189,190).
Differentiation Agents
All-trans-Retinoic Acid
ATRA is a differentiation agent used for the treatment of acute promyelocytic leukemia (APL). It is associated with retinoic acid syndrome, developing in 20% to 50% of APL patients receiving ATRA (191) a median of 7 days (range 0 to 35 days) after induction therapy (192). The clinical presentation includes fluid retention, weight gain, fever, and musculoskeletal pain, with progression to respiratory distress, pulmonary infiltrates, pleural (191) and pericardial effusions (182), renal insufficiency, skin infiltrates, hypotension, and death (191). Corticosteroids are highly effective when the syndrome commences but have limited utility once pulmonary symptoms are apparent. The putative mechanism of the pulmonary toxicity of ATRA is a capillary leak syndrome (182). Treatment is supportive.
Cardiac Toxicities
Antitumor Antibiotics
Anthracyclines. These are red-pigmented antibiotics (rhodomycins), which include doxorubicin, daunorubicin, idarubicin, and epirubicin (175). They are active against a broad spectrum of tumors, such as breast and esophageal carcinomas, HL and NHL, osteosarcomas, Kaposi sarcoma, and soft-tissue sarcomas. Acute cardiotoxicities include nonspecific ST-T wave changes (193), supraventricular tachycardia (SVT), ventricular dysrhythmias, myopericarditis, cardiomyopathy, and sudden death. The cardiomyopathy is dose dependent, and is classified as subacute and late. Subacute cardiomyopathy presents within 8 months of therapy, with a peak onset of 3 months, whereas late cardiomyopathy is observed after 5 or more years. A continual decline in left ventricular function results in CHF (175). Reduced cardiotoxicity has been observed with liposomal doxorubicin (194). Dexrazoxane, an iron chelator with cardioprotective properties, has been demonstrated to substantially reduce toxicity (195,196). Toxic effects may be compounded by other therapies, including trastuzumab, cyclophosphamide, dactinomycin, mithramycin, mitomycin, etoposide, melphalan vincristine, bleomycin, dacarbazine (175), and taxanes (197,198). In a recent study of patients with anthracycline-induced decrease in left ventricular ejection fraction (LVEF), treatment with enalapril and carvedilol resulted in normalization of LVEF in 42% of patients (199). Patients with CHF resulting from anthracycline-induced cardiomyopathy respond to standard treatment including ACE inhibitors, beta blockers, and loop diuretics (200).
Mitoxantrone. This agent has structural similarity to the anthracyclines, and is used in managing metastatic breast cancer, acute myeloid leukemia, and NHL (198). The mechanism of cardiac injury, like that of the anthracyclines, may involve iron chelation complexes (201); dysrhythmias and dose-dependent heart failure are toxicities. The incidence of a moderate-to-severe decrease in LVEF and of CHF is 13% and 2.6%, respectively, with a cumulative dose of less than or equal to 140 mg/m2. Doses below 110 mg/m2 decrease the incidence of heart failure, whereas incidence increases with doses greater than 160 mg/m2 (175). Again, standard therapy with ACE inhibitors, beta blockers, and diuretics are indicated for CHF.
Mitomycin C. In addition to its lung toxicity, mitomycin is cardiotoxic, resulting in an increased incidence of cardiac failure with cumulative doses exceeding 30 mg/m2 (175,202). Additive cardiotoxicity occurs when mitomycin is used in conjunction with anthracyclines (202); superoxide free radicals may mediate this toxicity (193).
Alkylating Agents
Cyclophosphamide. This agent is a nitrogen mustard alkylating agent effective in treating a variety of solid and liquid tumors. Acute cardiotoxicity may develop with doses of 120 to 170 mg/kg given over 1 to 7 days. ECG may reveal decreased QRS amplitude, nonspecific T-wave abnormalities, poor R-wave progression, supraventricular and ventricular tachydysrhythmias, and second-degree atrioventricular block (201). Acute fulminant CHF may occur in up to 28% of patients treated with high-dose cyclophosphamide (175), but CHF is usually short lived and reversible (201). The drug is metabolized to its active form in the liver by the cytochrome P-450, and more rapid metabolism amplifies the risk of CHF (203). Another cyclophosphamide-related cardiotoxicity is hemorrhagic myocarditis, putatively mediated by endothelial capillary injury, which results in pericardial effusion, tamponade, and death. Most effusions are treatable with corticosteroids and analgesics.
Ifosfamide. This is an alkylating agent, with similar properties to cyclophosphamide, used to treat lymphoma, leukemia, and testicular and bladder tumors. Dysrhythmias and transient, reversible, dose-dependent CHF—as with cyclophosphamide—may be seen (201,204).
Cisplatin. This agent cross-links interstrand DNA. It is used in treating cancers of the testes, bladder, ovaries, and other tumors. Bradycardia, SVT (175), acute ischemia (205), myocardial infarction, and ischemic cardiomyopathy may be observed (175). Acute chest pain and palpitations may be associated with cisplatin infusion. Hypomagnesemia and hypokalemia generated by cisplatin-induced tubular defects (175) may exacerbate arrhythmias (193). Venous thrombosis and pulmonary embolism have also been recognized (206).
Antimetabolites
5-Fluorouracil and Capecitabine. 5-Fluorouracil (5-FU) is a synthetic pyrimidine antimetabolite used in regimens for managing multiple solid tumors including GI, breast, ovarian, and head and neck malignancies. Myocardial ischemia, possibly triggered by coronary vasospasm, is a well-known cardiac toxicity that occurs with increased frequency in combination with cisplatin. In one study, silent ischemic ECG changes were identified during 24 hours of observation in up to 68% of patients receiving a continuous 5-FU infusion (207). Other cardiac manifestations include chest pain, angina, atrial and ventricular dysrhythmias, myocardial infarction, persistent ventricular dysfunction, sudden death, and cardiogenic shock (175) requiring inotropic support (175). Pre-existing cardiac morbidity significantly increases the risk of cardiotoxicity compared to no prior cardiac disease (15.1% vs. 1.5%). The oral equivalent of infused 5-FU is capecitabine, which exhibits a similar cardiotoxicity profile to 5-FU (208). Given the potential for severe cardiotoxicity, infusions should be terminated when chest pain occurs. If chest pain persists despite discontinuation of drug, administration of nitrates or calcium channel blockers results in resolution of symptoms in 70% to 90% of patients (209).
Differentiation Agents
All-trans-Retinoic Acid. Pericardial effusions, cardiac tamponade, myocardial ischemia (175), fatal infarction, and thrombosis (193), in addition to pulmonary toxicity, may occur with the retinoic acid syndrome as described above (175). (See also Pulmonary Toxicities.)
Arsenic Trioxide. Arsenic trioxide is a differentiation agent effective in treating relapsed acute promyelocytic leukemia. Like all-trans-retinoic acid, it may also cause the retinoic acid syndrome. Prolongation of the QT interval is another complication seen in up to 63% of patients, leading to torsades de pointes (175) and sudden death. The degree of QT prolongation is higher in the presence of hypokalemia; therefore, careful monitoring of electrolytes and maintaining levels in the high normal range is prudent (210).
Monoclonal Antibodies
Trastuzumab. There is an increased risk of cardiotoxicity associated with trastuzumab, which is highest in patients receiving concurrent anthracycline plus cyclophosphamide (27%) (211). The mechanism of cardiac toxicity of trastuzumab is not well understood, but cardiac erbB2 is essential for myocyte function, and trastuzumab targets both HER2 and erbB2 receptors (211). Early following initial treatment, there may be an asymptomatic decline in LVEF with late progression to dilated cardiomyopathy. Risk factors for cardiovascular toxicity include older age, cumulative doxorubicin dosage 400 mg/m2 or greater (213), and concurrent anthracycline and trastuzumab administration, rather than temporally separated dosing (212). Pertuzumab and ado-trastuzumab emtansine, other monoclonal antibodies that target HER2, are also associated with a decline in LVEF, although not as severe as trastuzumab. Trastuzumab-related cardiomyopathy is reversible with discontinuation of drug (212).
Rituximab. The CD20 antigen, present on normal and malignant B cells, is the target of the chimeric murine/human monoclonal antibody rituximab, which is used to treat leukemias and lymphomas, as well as benign diseases. Cardiac toxicity involves dysrhythmias and angina in less than 1% of infusions (175). Most adverse effects with rituximab are infusion related, usually occurring within 2 hours of the first infusion (213). Acute infusion-related deaths have been reported in 0.04% to 0.07% of cases. The clinical presentation in these patients includes hypoxia, pulmonary infiltrates, adult respiratory distress syndrome, myocardial infarction, ventricular fibrillation, and cardiogenic shock (213). Hypersensitivity reactions, including hypotension, angioedema, hypoxia, or bronchospasm, may occur in up to 10% of cases. Management is supportive, using intravenous fluids, antihistamines, acetaminophen, bronchodilators, and vasopressors (193).
Bevacizumab. This agent is associated with CHF, hypertension, and arterial thromboembolism. With bevacizumab monotherapy, 2% of patients developed moderate to life-threatening (grades 2 to 4) left ventricular dysfunction (see also Pulmonary Toxicities) (213). CHF developed in 14% of patients concurrently receiving anthracyclines, and in 4% of patients who had previously received anthracyclines or left chest wall irradiation (214). Clinical trials have also documented hypertension in 5% of patients, with reports of hypertensive crisis, hypertensive encephalopathy, and subarachnoid hemorrhage (193). The risk of fatal arterial thrombotic events is doubled to 5% in patients receiving intravenous 5-FU and bevacizumab (214,215).
Small Molecule Kinase Inhibitors
The drugs sunitinib, sorafenib, dasatanib, and lapatinib belong to the family of small molecule tyrosine kinase inhibitors (TKI). These agents interfere with VEGF, FLT, and PDGF-mediated pathways and have activity across a wide spectrum of malignancies.
Sunitinib
Hypertension induced by this agent is noted in 3% to 8% of individuals (216). Grade 3 or higher hypertension occurred in 7.3% of patients during phase I trials. In the phase II clinical trials of sunitinib for renal cell carcinoma 8.9% of patients developed a reduction in LVEF, and 3% developed NYHA stage 3 or 4 heart failure (216). Sunitinib-induced hypertension is usually reversible with discontinuation of drug (217); antihypertensive therapy is often necessary although no particular class has proved most efficacious in this setting.
Sorafenib
This agent is associated with an increased risk of myocardial ischemia and infarction of approximately 3% (218). In phase II studies with sorafenib 12% of patients developed grade 1 or 2 hypertension and 13.8% developed grade 3 hypertension (217). Antihypertensive therapy is often necessary with no particular class of medication favored.
Imatinib
This drug is used in the treatment of CML and ALL, and is associated with an incidence of symptomatic CHF of approximately 1% (219). Most patients will respond to diuresis and medical management.
Dasatanib
This is another TKI used in the treatment of CML and ALL and is associated with QT prolongation and CHF (<2%). No deaths have been attributed to cardiac adverse effects from dasatanib (220). Initial management includes reversal of other proximate causes of QT prolongation such as electrolyte derangements and discontinuation of medications affecting the QT interval.
Lapatinib
This agent, used in the treatment of herceptin refractory breast cancer that over-expresses HER2, is associated with an incidence of symptomatic heart failure in 1.3% patients treated longer than 6 months (221). Of note, many of these have already been exposed to anthracycline and herceptin-based therapy. Drug discontinuation and standard medical therapy for heart failure may be indicated.
Gastrointestinal Toxicities
Bevacizumab
Bevacizumab is associated with both bowel perforation in 0.2% to 3.2% of recipients, and grades 3 to 4 GI hemorrhage in 3.8% of patients (222). Perforation may occur at the site of a primary tumor, prior resection, prior radiation, or even in patients without evidence of peritoneal metastases. Although surgical exploration with repair or diversion is indicated in most patients with a perforated viscus, this decision must be made within the larger context of a patient’s overall prognosis and desire for aggressive interventions. When possible, surgery should be avoided for at least 28 days following bevacizumab.
Immunomodulatory Agents
The successful use of immunomodulatory antibodies in the treatment of melanoma and other malignancies heralds a new front in the treatment of cancer. The anti–cytotoxic T-lymphocyte–associated antigen 4 (CTLA-4) antibodies ipilimumab and tremelimumab (currently under development) as well as the anti–programmed cell death-1 receptor antibodies nivolumab and pembrolizumab carry a unique set immune–related toxicities affecting the skin, GI tract, liver, and endocrine system. These adverse events are triggered by a release of natural immunologic inhibition and are often successfully managed with a course of immunosuppressive therapy such as corticosteroids, tumor necrosis factor-alpha antagonists, or mycophenolate mofetil. The approach to treatment of these adverse events is based upon clinical experience, as there no prospective trials to guide therapy.
Diarrhea/Colitis
Diarrhea is a common side effect in patients undergoing treatment with checkpoint blocking antibodies and typically presents 6 weeks after initiation of therapy. It is more frequently seen in patients receiving anti–CTLA-4 immunotherapy than anti–PD-1 therapy (223,224). It is important to exclude other etiologies such as infection with bacterial or viral pathogens. Diarrhea was reported in approximately 30% of patients treated with ipilimumab for melanoma (223). Severe diarrhea—an increase of 7 or more stools above baseline—occurred in less than 10% of cases and significant colitis was reported in approximately 5% of patients (224).
Patients with mild symptoms (less than four stools per day over baseline) can be managed symptomatically with an American Dietetic Association colitis diet, antimotility agents, and oral budesonide. For patients with more than four to six stools per day above baseline, CT scan or, if unrevealing, colonoscopy may establish the diagnosis of colitis. If present, mild colitis is treated with IV corticosteroids (1 mg/kg prednisone). For patients with an increase of seven or more stools above baseline, high-dose corticosteroids (2 mg/kg prednisone) should be initiated. If the patient does not improve, infliximab at a dose of 5 mg/kg once every 2 weeks should be given, a regimen based upon treatment of inflammatory bowel disease (225,226). Mycophenolate may be necessary for colitis does not improve with infliximab.
Hepatotoxicity
Therapy with anti–CTLA-4 and PD-1 antibodies is associated with elevations in serum levels of the hepatic enzymes aspartate aminotransferase (AST) and alanine aminotransferase (ALT) (227,228). This occurs less than 10% of the time and is usually asymptomatic. Some patients have an associated fever. Hepatotoxicity most commonly occurs 8 to 12 weeks after initiation of treatment (229). CT scan may show mild hepatomegaly, periportal edema, or periportal lymphadenopathy (232). Liver biopsy, although not necessary to make the diagnosis, shows severe panlobular hepatitis with prominent perivenular infiltrate with endothelialitis (230).
Once elevated transaminases are identified, other causes of hepatotoxicity must be excluded. If no other etiology is identified, treatment with 1 to 2 mg/kg prednisone should be initiated as soon as possible. Elevated transaminases may persist for several weeks and may require prolonged or repeated corticosteroid tapers. Mycophenolate mofetil (500 mg every 12 hours) and antithymocyte globulin therapy may be used for patients with elevations in transaminases that do not improve with steroids (231).
Adrenal insufficiency is a rare but critical endocrinopathy associated with checkpoint blockade therapy, seen in less than 2% of cases (232). Patients present with hypotension, dehydration, and electrolyte derangements, which must be differentiated from other acute causes of hemodynamic instability. Emergent therapy includes intravenous corticosteroids (hydrocortisone 100 mg or dexamethasone 4 mg), aggressive management of fluid status, and correction of electrolyte abnormalities.
Key Points
Hypercalcemia
- The severity of symptoms correlates with both the degree of hypercalcemia and the rate at which the calcium is rising.
- Initial therapy consists of normal saline hydration to maintain a urine output of 150 to 200 mL/hr. Calcitonin and bisphosphonates are indicated for patients with severe hypercalcemia. Denosumab is indicated for patients with bisphosphonate-refractory hypercalcemia or renal insufficiency.
Tumor lysis syndrome
- Cornerstones of therapy include aggressive hydration with maintenance urine output of 80 to 100 mL/m2/hr or greater, frequent monitoring and correction of electrolyte derangements, and administration of a hypouricemic agent such as rasburicase or allopurinol.
SVCS
- CT scan with IV contrast can often establish the diagnosis, define the level of blockage, document collateral circulation, and identify the underlying cause.
- The relative ease and availability of endovascular stenting has obviated the need for emergent RT prior to histologic diagnosis in most cases.
Malignant Spinal Cord Compression
- Pretreatment neurologic function is the most important predictor of posttreatment outcome.
- The choice of definitive therapy must take into account the patient’s overall prognosis; Surgery is recommended for patients with unstable spine lesions or radiation resistant tumors.
Malignant Pericardial Effusion
- The diagnosis is suspected based on physical examination, ECG, chest x-ray, and echocardiography.
- Percutaneous and surgical approaches are both effective and lead to prompt improvement in hemodynamic compromise. When technically feasible, percutaneous drainage is preferred in most patients.
Neutropenic Enterocolitis
- Patients with uncomplicated NE should receive empiric broad-spectrum antibiotics, bowel rest, NG decompression, transfusional support, and hydration. Surgery is reserved for patients with overt perforation or uncontrolled bleeding.

Full access? Get Clinical Tree
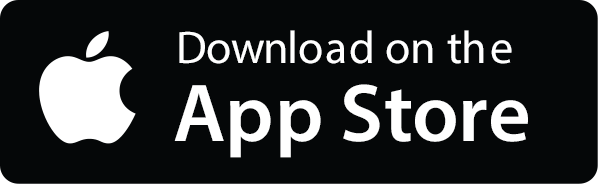
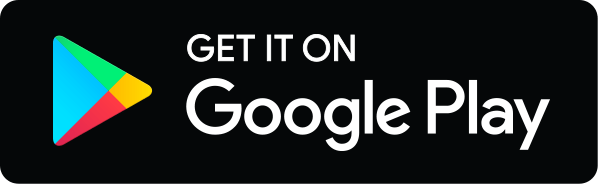