Occupational Health
Jonathan D. Katz
Robert S. Holzman
Key Points








Related Matter
Fixation Errors
ASA Seal
Needle Stick
Chain of Communication
The health-care industry has the dubious distinction of being one of the most hazardous places to work in the United States. According to the U.S. Bureau of Labor, health care is second only to manufacturing in the number of occupational illnesses and injuries sustained by their workers.1 Anesthesia personnel are at risk of acquiring a number of occupational illnesses and injuries that are specific to the high-intensity environment of the operating room. Workplace hazards include exposure to toxic chemicals and waste anesthetic gases, ionizing radiation, and infectious agents. They are vulnerable to injury from fires or explosions. In addition, anesthesia personnel are susceptible to emotional and psychological disorders, such as burnout and substance abuse, engendered at least in part by the high stress and other demands inherent in their work.
Only within the past few decades have comprehensive programs been developed to assess the health of anesthesia personnel and protect against and treat many of these occupational diseases. There are numerous potential health risks to anesthesia personnel, but with heightened awareness of the problems and the use of proper precautions, they are avoidable or manageable.
Physical Hazards
Anesthetic Gases
Concerns about possible toxic effects of occupational exposure to inhalation anesthetics have been expressed since their introduction into clinical practice. Investigations have included cellular research, in vitro and in vivo studies, and epidemiologic surveys. Specific focuses of the research have been whether or not occupational exposure to waste anesthetic gases is associated with cytotoxic and genotoxic changes, organ toxicity, adverse reproductive outcomes, impairment of psychomotor skills, or premature death.
Evidence exists to show that cellular damage can result from chronic exposure of cells to high concentrations of anesthetic gases in the laboratory.2 However, it can be misleading to extrapolate data from studies in cellular cultures or experimental animals to the real-world experience of humans working in operating rooms. The reports regarding possible cellular injury from waste anesthetic gases are inconsistent. Several studies testing for chromosomal aberrations, sister chromatid exchanges, or changes in peripheral lymphocytes have found no evidence of cellular damage among clinicians exposed to the levels of anesthetic gases that are encountered in an adequately ventilated operating room.3 On the other hand, individuals who are exposed to high ambient concentrations of waste gases, such as in anesthetizing locations where there is inadequate ventilation or scavenging, may be subject to a dose-dependent increase in cytotoxic changes.4 Reports on cellular changes thought to result from waste anesthetic gas exposure are difficult to compare because of the inability to standardize other risk factors to which operating room personnel are exposed, such as radiation, long work hours, and stress. Most sources agree that occupational exposure to the low levels of anesthetics found in operating rooms with effective waste gas scavenging is not associated with significant cellular effects.5
Nitrous oxide exposure is a special situation.6 Nitrous oxide can irreversibly oxidize the cobalt atom of vitamin B12 to an inactive state. This inhibits methionine synthetase and prevents the conversion of methyltetrahydrofolate to tetrahydrofolate, which is required for DNA synthesis, assembly of the myelin sheath, and methyl substitutions in neurotransmitters. At clinically utilized concentrations of nitrous oxide, this inhibition could result in anemia and polyneuropathy. As with the halogenated hydrocarbon anesthetics, these effects with nitrous oxide have not been demonstrated in operating rooms with effective waste gas scavenging.
Reproductive Outcomes
An adverse impact of waste anesthetic gases on reproductive outcomes has been a persistent concern. The earliest reports suggested an association between long-term exposure to waste anesthetic agents among health-care personnel and infertility, spontaneous abortions, and congenital abnormalities.7 The American Society of Anesthesiologists (ASA) and the National Institute for Occupational Safety and Health (NIOSH) published a large retrospective study in 1974 in which 49,585 operating room personnel who had potential exposure to waste anesthetic gases were compared with a nonexposed group of 23,911 health-care professionals.8 Among the findings in this study was an increased risk of spontaneous abortion and congenital abnormalities in children of women who worked in the operating room and an increased risk of congenital abnormalities in offspring of unexposed wives of male operating room personnel.
In response to criticism of the methodology and data analysis in this study, ASA commissioned a group of epidemiologists and biostatisticians to do a meta-analysis using data from this and 16 other published epidemiologic surveys.9 They found only six studies on spontaneous abortion and congenital abnormalities in offspring of anesthesia personnel that they felt were free of errors in study design or statistical analysis. From these studies, they reported the relative risks of spontaneous abortion for female physicians and female nurses working in the operating room were 1.4 and 1.3, respectively (a relative risk of 1.3 represents a 30% increase in risk when compared with the risk of the control population). The increased relative risk for congenital abnormalities was of borderline statistical significance for exposed physicians only. Although they did find a statistically significant relative risk of spontaneous abortion and congenital abnormalities in women working in the operating room, the relative risk was small compared with other, better-documented maternal risk factors, such as cigarette smoking and long working hours. The reviewers also pointed out that duration and levels of anesthetic exposure were not measured in any of the studies and that other confounding factors were not adequately controlled.
Other medical specialties with occupational exposure to anesthetic gases have also conducted studies among their health-care professionals. Studies of female dental assistants have revealed a reduction in fecundability and an increased rate of spontaneous abortion among women who worked in offices not using scavenging equipment.10,11 In offices where scavenging devices were used to vent waste nitrous oxide, there was no evidence of an increased risk for adverse reproductive outcomes. Findings from both of these studies of dental assistants must be viewed with caution because the estimates of nitrous oxide exposure were based solely on respondents’ retrospective reports.
Veterinarians and others who work in animal hospitals are a third group of professionals who may have frequent exposure to waste anesthetic gases. In a study of 744 pregnant female veterinarians in Australia, there was a 2.5-fold increase in the risk of preterm delivery in those exposed to unscavenged anesthetic gases for one or more hours per week as compared with unexposed workers.12 Data collected by the same group demonstrated no increased prevalence of birth defects as a result of maternal exposure to anesthetic gases.13
A meta-analysis in 1997, of 19 epidemiologic studies, which included hospital workers, dental assistants, veterinarians, and
veterinary assistants, demonstrated an increased risk of spontaneous abortion in women with occupational exposure to anesthetic gases (RR—1.48).14 Stratification by job category indicated that the relative risk was greatest for veterinarians (RR—2.45), followed by dental assistants (RR—1.89) and hospital workers (RR—1.30). When the meta-analysis was confined to five studies that controlled for several nonoccupational confounding variables, had appropriate control groups, and had a sufficient response rate, the relative risk for spontaneous abortion was 1.90. The author noted that the routine use of scavenging devices had been implemented since the time that most of the studies in this meta-analysis were performed and that there was no increased risk of spontaneous abortion in studies of personnel who worked in environments where waste gases were scavenged.
veterinary assistants, demonstrated an increased risk of spontaneous abortion in women with occupational exposure to anesthetic gases (RR—1.48).14 Stratification by job category indicated that the relative risk was greatest for veterinarians (RR—2.45), followed by dental assistants (RR—1.89) and hospital workers (RR—1.30). When the meta-analysis was confined to five studies that controlled for several nonoccupational confounding variables, had appropriate control groups, and had a sufficient response rate, the relative risk for spontaneous abortion was 1.90. The author noted that the routine use of scavenging devices had been implemented since the time that most of the studies in this meta-analysis were performed and that there was no increased risk of spontaneous abortion in studies of personnel who worked in environments where waste gases were scavenged.
It is likely that other job-associated conditions besides exposure to trace anesthetic gases may account for many of the adverse reproductive outcomes reported among some health-care workers (HCWs). These include stress, infections, long work hours, shift work, and radiation exposure. A survey of 3,985 Swedish midwives demonstrated that night work was significantly associated with spontaneous abortions after the 12th week of pregnancy (odds ratio 3.33), while exposure to nitrous oxide appeared to have no effect.15 Preterm birth in obstetric and neonatal nurses was associated with increased work hours, hours worked while standing, and occupational fatigue.16 And in a study of female veterinarians, the risks of birth defects were highest among those exposed to radiation and to long working hours (>45 hours/week) but not to those exposed to waste anesthetic gases.12

Cancer
A 1968 report on the health of anesthesiologists concluded that male anesthesiologists had an increased risk of malignancies of the lymphoid and reticuloendothelial tissues but a lower death rate from lung cancer.17 Data from a subsequent prospective study by the same group contradicted the original findings and found no evidence that lymphoid malignancies were an occupational hazard for anesthesiologists.18
The 1974 ASA-sponsored study (vide supra) found no differences in cancer rates between men exposed and those not exposed to trace concentrations of anesthetic gases.8 There was a 1.3- to 2-fold increase in the occurrence of various forms of cancer among exposed women, predominantly from an increase in leukemia and lymphoma. The subsequent reanalysis of these data confirmed an increase in relative risk of cancer in exposed women (RR 1.4) but attributed the increase solely to cervical cancer (RR 2.8).9 These authors also noted that the original study did not assess the effect of confounding variables, such as sexual or smoking history, that may have contributed to the findings. It is doubtful that these purported carcinogenic effects of anesthetics would be sex-related, and the conflicting results for men and women, especially in light of the low statistical significance of the data, cast doubt that anesthetics were the causative agents.
A subsequent ASA-sponsored study of anesthesiologists, covering the period from 1976 to 1995, used data on the cause of death from the National Death Index.19 The mortality risks of a cohort of 40,242 anesthesiologists were compared with a matched cohort of internists. There was no difference between the two groups in overall mortality risk or mortality from cancer.
Behavioral Effects
One of the principal reasons identified by the NIOSH for limiting occupational exposure to waste anesthetic gases is to prevent “decrements in performance, cognition, audiovisual ability and dexterity”.20 The clearest evidence for behavioral effects from trace gas exposure comes from work with laboratory animals where concentration and duration of exposure can be precisely controlled. In a study of rats exposed for 30 days to 1/10 minimum alveolar concentration (MAC) of halothane, sevoflurane, or desflurane, there were significant alterations of exploratory activity, curiosity, anxiety, learning, and memory functions in the exposed animals.21
The effect on psychomotor performance among human volunteers varies considerably depending upon the concentration of anesthetic gas to which the subjects are exposed. Temporary lethargy and fatigue are the most common symptoms described after brief exposures to subanesthetic concentrations of anesthetic gases. Longer exposures and higher concentrations have been associated with changes such as headache, depression, anxiety, loss of appetite, loss of memory, decreased reaction time, and decrements in cognitive function. Most of the measurable psychomotor and cognitive impairments produced by brief exposures are short-lived and disappear within 5 minutes of cessation of exposure.22
One of the most dramatic demonstrations of psychomotor impairment resulting from chronic anesthetic gas exposure appeared as a case report in which two anesthesiologists in the same institution developed chronic toxic encephalopathy.23 Each of them had spent many years doing pediatric cases requiring induction and maintenance of anesthesia by mask in two operating rooms that were subsequently shown to have insufficient fresh air supply resulting in high ambient concentrations of anesthetic gases. In both cases, symptoms gradually improved after the individuals were removed from the offending environment.
Levels of Trace Anesthetic Gases
The first report of occupational exposure to modern anesthetics was by Linde and Bruce24 in 1969 who noted an average concentration of halothane of 10 ppm and of nitrous oxide of 130 ppm in the air surrounding an active anesthesia machine. End-expired air samples taken from anesthesiologists after work were as high as 12 ppm of halothane. It was later demonstrated that with appropriate scavenging equipment and with adequate air exchange in the operating room, levels of waste anesthetic gases could be significantly reduced.25
Elevated levels of volatile anesthetics have also been identified in the postanesthesia care unit (PACU). This typically occurs as a product of exhaled gases from recovering patients. In one study, the time-weighted average (TWA) concentrations for isoflurane, desflurane, and nitrous oxide were 1.1, 2.1, and 29 ppm, respectively, in the breathing zone of PACU nurses.26 In this study, the tracheas of half of the patients were intubated on arrival in the PACU, suggesting that the patients were still partially anesthetized and were exhaling a greater concentration of anesthetic gases than if they had more completely recovered in the operating room. In contrast, in two institutions where nitrous oxide was discontinued at the end of surgery approximately 5 minutes before the patient left the operating room and adequate air exchanges were maintained in the PACU, the time-weighted nitrous oxide levels were less than 2.0 ppm.27 Minimal levels of anesthetic gases can be obtained in the PACU by ensuring adequate room ventilation and fresh gas exchange and by discontinuing the anesthetic gases in sufficient time before leaving the operating room.
Recommendations of the National Institute of Occupational Safety and Health (NIOSH) and the Occupational Safety and Health Administration (OSHA)
In 1977, NIOSH issued a criteria document that included recommended exposure limits (RELs) for waste anesthetic gases of 2 ppm (1-hour ceiling) for halogenated anesthetic agents when used alone or 0.5 ppm for halogenated agent and 25 ppm of nitrous oxide when used together (TWA during the period of anesthetic administration).20 In addition, it stated that operating room employees should be advised of the potential harmful effects of anesthetics. The guidelines proposed that annual medical and occupational histories be obtained from all personnel and that any abnormal outcomes of pregnancies should be documented. The publication also included information on scavenging procedures and equipment and methods for monitoring concentrations of waste anesthetic gases in the air.
In a subsequent publication, NIOSH issued an alert to warn health-care personnel that exposure to nitrous oxide may produce “harmful effects”.28 In this document, NIOSH recommends the following to reduce nitrous oxide exposure: Monitoring the air in operating rooms, implementation of appropriate engineering controls, work practices, and equipment maintenance procedures, and institution of a worker education program.
NIOSH has not yet developed RELs for the agents most commonly used in current practice (isoflurane, sevoflurane, and desflurane). These volatile agents have potencies, chemical characteristics, and rates and products of metabolism that differ significantly from older anesthetics.
Occupational Safety and Health Administration (OSHA), which is the federal agency that is responsible for enacting job health standards and investigating work site violations has never adopted the NIOSH criteria document and has not set a standard permissible exposure limit for waste anesthetic gases. However, most states have instituted regulations calling for routine measurement of ambient gases in operating rooms and have mandated that levels not exceed an arbitrary maximum. Other organizations, such as the American Conference of Governmental Industrial Hygienists (www.acgih.org), have established their own occupational exposure limits for waste anesthetic gases, in many cases greater than those recommended by NIOSH.
It is prudent to institute measures that reduce waste anesthetic levels in the operating room environment to as low as possible. To ensure reduced occupational exposure, facilities where anesthetics are administered should have procedures to monitor for detection of leaks in anesthesia machines and contamination as a result of faulty anesthetic techniques (e.g., poor mask fit, leaks around endotracheal tube cuffs and laryngeal mask airways, and scavenging system malfunctions) (Table 3-1). When there have been leaks of anesthetic gases, dispersion and removal of the pollutants depends upon adequate room ventilation, at least 15 to 21 air exchanges per hour with three bringing in outside air. Through the use of scavenging equipment, equipment maintenance procedures, appropriate anesthetic work practices, and efficient operating room ventilation systems, the environmental anesthetic concentration can be reduced to minimal levels.
Chemicals
Methyl methacrylate is commonly used in various surgical procedures. Known cardiovascular complications of methyl methacrylate in surgical patients include hypotension, bradycardia, and cardiac arrest. Reported risks from repeated occupational exposure to methyl methacrylate include skin irritation and burns, allergic reactions and asthma, eye irritation including possible corneal ulceration, headache, and neurologic signs. Airborne concentrations greater than 170 ppm have been associated with chronic lung, liver, and kidney damage. In one report, a HCW suffered significant lower limb neuropathy after repeated occupational exposure to methyl methacrylate.29 Concentrations as high as 280 ppm have been measured when methyl methacrylate is prepared for use in the operating room. OSHA recommends use of scavenging devices in order to maintain an 8-hour TWA exposure to methyl methacrylate of 100 ppm.
Table 3-1. Sources of Operating Room Contamination | |||||||
---|---|---|---|---|---|---|---|
|
Allergic Reactions
Allergic reactions to volatile anesthetic agents and to some muscle relaxants have been associated with contact dermatitis, hepatitis, and anaphylaxis in individual anesthesiologists.30,31 Analyses of sera from pediatric anesthesiologists exposed to halothane demonstrated an increased prevalence of autoantibodies to cytochrome P450 2E1 and hepatic endoplasmic reticulum protein (ERp58).32
Despite the presence of these autoantibodies, only 1 of 105 anesthesiologists had findings of any hepatic injury. These data suggest that although autoantibodies may occur in anesthesiologists frequently exposed to volatile anesthetics, they do not appear to commonly cause anesthetic-induced hepatitis.
Despite the presence of these autoantibodies, only 1 of 105 anesthesiologists had findings of any hepatic injury. These data suggest that although autoantibodies may occur in anesthesiologists frequently exposed to volatile anesthetics, they do not appear to commonly cause anesthetic-induced hepatitis.
Table 3-2. Types of Reactions to Latex Gloves | ||||||||||||||||||||||||||||
---|---|---|---|---|---|---|---|---|---|---|---|---|---|---|---|---|---|---|---|---|---|---|---|---|---|---|---|---|
|
Latex in surgical and examination gloves has become a common source of allergic reactions among operating room personnel. In many cases, HCWs who are allergic to latex experience their first adverse reactions while they are patients undergoing surgery. The prevalence of latex sensitivity among anesthesiologists is approximately 12%.33
The latex found in medical products is actually a composite of many substances including proteins, polyisoprenes, lipids, and phospholipids combined with preservatives, accelerators, antioxidants, vulcanizing compounds, and lubricating agents (such as cornstarch or talc). The protein content is responsible for most of the generalized allergic reactions to latex-containing surgical gloves. These reactions are exacerbated by the presence of powder that enhances the potential of latex particles to aerosolize and to spread to the respiratory system of personnel and to environmental surfaces during the donning or removal of gloves.
Irritant or contact dermatitis accounts for the majority of reactions resulting from wearing latex-containing gloves (Table 3-2). True allergic reactions present as T-cell–mediated contact dermatitis (type IV) or as an immunoglobulin E–mediated anaphylactic reaction.
Anesthesiologists who believe that they are allergic to latex must avoid all direct contact with latex-containing products. It is also important that coworkers wear non-latex or powderless, low latex-allergen gloves to limit the levels of ambient allergens.
Radiation
Anesthesia personnel are at risk of exposure from both direct and indirect sources of ionizing radiation. Direct sources of radiation include exposure from the primary x-ray beam and leakage from other sites within the x-ray equipment. Indirect exposure results from scattered radiation reflected off surfaces such as tables, other equipment, and patient.
The biologic consequences of radiation exposure vary depending on age, gender, and specific organ site of exposure. The deterministic effects of radiation cause cell death and organ injury and are cumulative in a dose-related fashion. Common examples of deterministic injuries include skin damage, infertility, and certain types of cataracts. Stochastic effects of radiation are those that result in DNA injury and the development of cancer. There is no known threshold below which the risk of developing cancer completely disappears. And there can be a long latency period before the clinical presentation of an induced neoplasm.
OSHA has published occupational limits for workers exposed to ionizing radiation.34 The annual limit is 5 rem with an allowable long-term limit of (N – 18) × 5 rem where N is the age in years. The recommended maximum occupational exposure to a declared pregnant worker should not exceed a monthly limit of 0.5 mrem or a total exposure of 5 mrem (excluding medical and natural background radiation).
Early studies found the exposure to radiation among anesthesia personnel to be safely below the OSHA limits.35 However, more recent studies, conducted subsequent to the increased utilization of ionizing radiation in operating rooms, cardiac catheterization, and other interventional radiology suites, have revealed a worrisome trend toward increased exposure among anesthesia personnel (although still well below OSHA limits).36,37,38 In one study, there was a doubling of the aggregate radiation exposure to members of a department of anesthesiology in the year following the introduction of an electrophysiology laboratory38 (Fig. 3-1). Anesthesia personnel in this study increased their average exposure to almost 500 mrem on an annualized basis. Preventative strategies for anesthesiologists to minimize their risk of radiation-induced injury include limiting the intensity and exposure time, distancing oneself from the source of the radiation, and using maximal shielding from both primary and scattered sources of radiation.
A second form of radiation with potential health hazards comes in the form of chronic exposure to low-frequency electromagnetic
fields such as those emitted by MRI equipment.39 It is often necessary for the anesthesia care provider to remain in close proximity to the patient, and thus the magnet core, during MRI studies. Data are not yet available to determine the safety of long-term exposure to high-intensity magnetic fields. Therefore, until such time that safety thresholds have been determined for this type of exposure, anesthesiologists should obey the general admonition regarding all radiation exposure. That is, keep exposure as low as reasonably achievable.
fields such as those emitted by MRI equipment.39 It is often necessary for the anesthesia care provider to remain in close proximity to the patient, and thus the magnet core, during MRI studies. Data are not yet available to determine the safety of long-term exposure to high-intensity magnetic fields. Therefore, until such time that safety thresholds have been determined for this type of exposure, anesthesiologists should obey the general admonition regarding all radiation exposure. That is, keep exposure as low as reasonably achievable.
Noise Pollution
Noise is quantified by determining both the intensity of the sound and the duration of the exposure. OSHA has determined that the maximum level for safe noise exposure is 90 dB for 8 hours.40 Each increase in noise of 5 dB halves the permissible exposure time, so that 100 dB is acceptable for just 2 hours per day. The maximum allowable exposure in an industrial setting is 115 dB.
Noise levels in a modern operating room frequently exceed OSHA limits and are a potential health hazard. Ventilators, suction equipment, music, and conversation produce background noise at a level of 75 to 90 dB. Superimposed on these are sporadic noises caused by dropped equipment, surgical saws and drills, and alarms. Resultant noise levels can exceed 100 dB over 40% of the time with peak levels in excess of 120 dB, which is comparable to the clamor of a busy freeway or a rock and roll band 41 (Table 3-3).
Excessive levels of noise can adversely influence an anesthesiologist’s capacity to perform common clinical tasks. Mental efficiency, short-term memory, and ability to multitask and perform complex psychomotor tasks are all diminished by exposure to excess noise.
Noise also interferes with the ability to hear alarms. This is especially apparent in the magnetic resonance imaging suite where noise from the scanner can mask many anesthesia alarms. Similarly, excessive noise can interfere with crucial verbal communication. Voice levels must be at least 20 dB above background noise to be heard and understood.
There are also chronic health ramifications of long-term exposure to excessive noise. Chronic exposure has been associated with elevated levels of endogenous catecholamines and heightened levels of stress, increased irritability, and elevated blood pressure.
Table 3-3. Noise Levels in a Modern Operating Room Frequently Exceed Osha Limits | ||||||||||||||||||||
---|---|---|---|---|---|---|---|---|---|---|---|---|---|---|---|---|---|---|---|---|
|
Ultimately, exposure to excessive noise levels will result in hearing loss. Although no direct connection has been established with excess noise experienced in the operating room, it is interesting to note that more than 50% of anesthesiologists have a substantial hearing deficit and 7% have deficits that potentially interfere with their ability to hear operating room alarms.42
One form of background noise, music, can provide a number of beneficial effects. Music has proved advantageous as a supplement to sedation and analgesia for surgical patients.43 Self-selected background music can contribute to reducing autonomic responses in surgeons and improving their performance.44 The beneficial effects are less pronounced when a third party chooses the music. The selection of music, and the volume at which it is played, should be determined by mutual agreement of all parties present in the operating room.
Ergonomics/Human Factors
Human factor analysis, also called ergonomics, is the study of the interaction between humans and machines and the impact of equipment design on their use. It is a multidisciplinary science that applies diverse disciplines such as anthropometry, ethnography, biomechanics, industrial and social psychology, architecture, education, and information technology, toward developing user-friendly equipment and a safer workplace.
Human factor analysis has been most widely employed in industries such as aviation, nuclear power, and oil exploration where human error frequently has contributed significantly to catastrophic accidents. The work performed by an anesthesiologist shares many of the characteristics found in these industries, including the intricacy of the tasks, a narrow margin of error, and the vulnerability to human error.



A number of other work place factors conspire to interfere with anesthesiologists’ ability to perform their complex tasks. Even seemingly trivial aspects of an anesthesiologist’s work that require the expenditure of excessive energy produce a decrement in performance over the course of time. For example, if the anesthesiologist must make frequent rapid changes in observation from a dim, distant screen to a bright, nearby one, the continuous muscular activity required for pupil dilation and constriction and lens accommodation promotes fatigue and hinders performance.
The detrimental effects of unnecessary energy expenditure can be mental as well as physical. As a result of the ever-increasing number of monitors to be observed and amounts of data to be processed during the course of a surgical procedure, larger amounts of mental work must be expended. The cognitive burden varies directly with the difficulty encountered in extracting information from the monitors and displays that compete for the anesthesiologist’s attention. Poor engineering of the monitor displays, so that mode of presentation, signal frequency, or strength is suboptimal, can adversely influence the operator’s performance.
Even the alarms that have been developed with the specific goal of augmenting the task of vigilance can have considerable drawbacks. In general, alarms are nonspecific (the same alarm signaling as many as 12 different deviations from “normal”) and can be a source of frustration and confusion. They are susceptible to many artifacts and false positives that can cause “alarm fatigue” and distract the observer from more clinically significant information. It is not unusual for distractive alarms to be ignored or inactivated.46 A positive trend that is emerging in alarm technology is the development of “knowledge-based alarms” that can integrate information from more than one monitor and suggest a list of diagnostic and therapeutic possibilities.

Effective conflict resolution is an important element of the teamwork necessary for successful surgical outcomes. Conflict and unpleasant interpersonal interactions among team members are among the most stressful aspects of the job of an anesthesiologist and can hinder safe anesthetic care. Some degree of conflict occurs during the management of as many as 78% of patients in high-intensity areas such as operating rooms or critical care units.47
Successful resolution of conflict is a skill that can be learned.48 Mutual respect is required among team members along with a willingness to carefully listen and recognize differences of opinion. Intervention by a neutral third party is frequently helpful in finding an innovative solution. The airline industry has successfully implemented crew resource management programs to improve the performance of cockpit teams.49
“Production pressure” is an organizational concern that has the potential to create an environment in which issues of productivity supersede those of safety.50 Production pressure has been associated with the commission of errors resulting from haste and/or deliberate deviations from known safe practices.
The application of simulation technology is gaining acceptance as a tool to study and teach human performance issues in anesthesiology. It appears to be particularly suited to training nontechnical skills such as resource management, teamwork, and communication.51
Work Hours, Night Call, and Fatigue

Sleep loss and fatigue can have deleterious effects upon work practices. In general, workers who are sleep deprived suffer a decrement in performance and are at greater risk of committing workplace errors. Fatigued individuals are more susceptible to “microsleeps” which are brief, uncontrolled, and spontaneous episodes of physiologic sleep that may last as little as a few seconds. Fatigued workers also incur more work-related accidents.52 The susceptibility to accident is not limited to work hours and
extends to other activities of daily living, such as driving. The changes resulting from sleep deprivation bear a striking similarity to that seen with alcohol intoxication.53 Significant individual variations in impairment due to fatigue have been identified.54
extends to other activities of daily living, such as driving. The changes resulting from sleep deprivation bear a striking similarity to that seen with alcohol intoxication.53 Significant individual variations in impairment due to fatigue have been identified.54
The contribution of sleep loss and fatigue to accidents has been well documented in many well-publicized industrial catastrophes. Sleep deprivation was a contributing factor to industrial accidents such as those that occurred at Chernobyl, Three Mile Island, Exxon-Valdez, and the Challenger space shuttle catastrophe.
A number of reports have also identified sleep deprivation as a causative factor in errors occurring in the health-care industry.55 As early as 1971, Friedman et al. reported that interns made almost twice as many errors reading electrocardiograms after an extended work shift than after a night of sleep.56 Intubation skills were reduced among emergency room physicians working night shifts when compared with other staff members working days.57 Physicians in both groups were more likely to commit errors during a simulated triage test toward the end of their work shifts. And in a study that examined the management by medical interns of medical admissions, 35.9% more medical errors were committed while on a “traditional” schedule (24 hours plus work shifts) than when they worked a schedule that eliminated extended work shifts and reduced the number of hours worked per week.58 Mistakes committed by these interns included 20.8% more medication errors and 5.6 times as many diagnostic errors.
Anesthesiologists who take night call commonly suffer from each of the three well-defined classes of sleep deprivation: Total, partial, and selective sleep deprivation. Interruption of sleep during call commonly occurs between the hours of 2 AM and 4 AM when humans are most vulnerable to fatigue-induced errors.
A number of specific consequences of sleep deprivation have the potential to adversely impact the conduct of a safe anesthetic, including impaired cognition, short-term memory and clinical decision making, prolonged reaction time, and reduced attention, vigilance, and performance. In a report by Howard et al.,59 sleep-deprived residents managing a 4-hour simulated anesthetic demonstrated progressive impairment of alertness, mood, and performance and had longer response latency to vigilance probes.
Performance after sleep deprivation does not return to normal levels until 24 hours of rest and recovery has occurred after a period of sleep deprivation. Residents in their routine, non-post call state continue to suffer from chronic sleep deprivation and have the same degree of sleepiness as measured in residents finishing 24 hours of in-house call.60
An interesting phenomenon is the “end-spurt,” in which previously deteriorated performance shows improvement when the subject expects that the task is nearly completed. However, if the procedure is unexpectedly prolonged, a “let-down” occurs with additional deterioration in performance.
Despite adequate evidence of performance impairment due to fatigue, the precise role of sleep deprivation on the specific end point of clinical outcomes remains unclear. A number of studies have reported a detrimental effect on patient outcomes of sleep deprivation among providers. In two studies of American anesthesia caregivers, more than 50% reported having committed an error in medical judgment that they attributed to fatigue.50,61 And 58% of New Zealand anesthesiologists reported that they had exceeded their self-defined limit for safe continuous administration of anesthesia and 86% reported that they had committed a fatigue-related error.62 Similar reports of fatigue-related complications appear in the surgical literature with as many as 16% of preventable adverse surgical events attributed to surgeon fatigue.63,64 However, others have reported no evidence of suboptimal clinical management or poor outcomes from sleep-deprived clinicians. Chu et al. found no increase in mortality or major complications in 4,000 consecutive cardiac surgical procedures performed by surgeons who had varying degrees of sleep (ranging from 0 to >6 hours) the night before surgery.65 Other studies of surgeons and critical care specialists have agreed with these findings.66,67
Several factors help to explain the apparent disparity between reports of fatigue-related performance impairment and the failure to conclusively link these with medical errors or adverse outcomes. The distinction between sleep deprived and well rested is often arbitrarily defined with varying definitions of the non-rested state. For example, in one study the general conclusion was that there were no overall difference in complication rates between surgeons who had operated during the previous night (“post-nighttime”) and rested surgeons.68 However, when sleep patterns were stratified, there was a substantially elevated rate of complication (6.2% vs. 3.4%) if the surgeon had less than 6 hours sleep during the on-call night. Also, there is great difficulty in eliminating confounding variables such as the impact of loss of continuity of care, errors that can occur during “hand-offs” of critically ill patients, and the reallocation of many medical tasks from physicians to nonphysician providers. Finally, it can be difficult to extrapolate findings from simulation studies of volunteers in a laboratory to clinicians in real-life work conditions.
Medicine remains significantly behind other industries, most notably the transport and airline industries, in identifying and regulating work practices that permit excessively long shifts. It was not until the well-publicized Libby Zion case in 1984 (in which it was charged that fatal, avoidable mistakes were made by exhausted, unsupervised residents) that medical organizations and state legislatures took action to limit excessive work hours among residents. In 2000 the Accreditation Council for Graduate Medical Education (ACGME) established the first set of standards to limit resident duty hours. These standards were revised by the ACGME in 2011.69 Policies established by the ACGME include the following:
Work hours must be limited to 80 hours per week, averaged over a 4-week period inclusive of all in-house call activities and all moonlighting.
Duty periods of PGY-1 residents must not exceed 16 hours in duration.
Duty hours of PGY-2 residents and above may be scheduled to a maximum of 24 hours of continuous duty in the hospital.
Strategic napping, especially after 16 hours of continuous duty and between the hours of 10:00 PM and 8:00 AM, is strongly suggested.
Residents should have 10 hours and must have 8 hours, free of duty between scheduled duty periods and at least 14 hours free of duty after 24 hours of in-house duty.
Somewhat ironically, at the end of a long list of duty hours restrictions, item VI.G.5.a-c states, “Residents in the final years of education (as defined by the Review Committee) must be prepared to enter the unsupervised practice of medicine and care for patients over irregular or extended periods.” This statement recognizes that these restrictions on duty periods apply only to trainees and that work hours in medical practice remain unregulated.
Prolonged work hours and sleep deprivation are a ubiquitous component of many anesthesiologists’ professional lives. Many academic faculty members now work longer hours than they did prior to house staff work hour limitations because of the shift of work from residents to faculty. Attending anesthesiologists and nurse anesthetists commonly work 10- to 12-hour workdays and 24-hour on-call shifts. Gravenstein et al. reported that the average anesthesiologist’s work week was 56 hours and that 74% of the respondents had worked without a break for longer periods than they personally thought was safe.61
Several strategies have been devised to reduce fatigue and limit the adverse effects of sleep deprivation when long work periods are necessary. Recommendations include minimizing sleep debt by maximizing sleep before on-call shifts and utilizing maneuvers to overcome sleep inertia such as increasing ambient light levels and stretching, taking frequent breaks, and napping when possible. A number of pharmaceutical aids, such as caffeine and modafinil (a schedule IV drug), have been approved for military use and, if used under supervision and carefully monitored, arguably may be helpful for clinicians with shift-work sleep disorder.70
Infectious Hazards
Anesthesia personnel are at risk for acquiring infections from patients and from other personnel. This risk is likely to increase because as antimicrobial agents become more effective, the pathogens develop resistance and new survival strategies. In addition, increasingly immune-compromised patients become vectors for these resistant, opportunistic organisms. Furthermore, globalization brings with it increasing spread of organisms from less developed areas in the world, such as the 1999 outbreak in New York of West Nile Virus encephalitis and in 2003 of the epidemic of severe acute respiratory syndrome (SARS) in Hong Kong. Moreover, diseases that were once thought to be noninfectious, such as peptic ulcer disease (Helicobacter pylori), invasive cervical cancer (human papillomavirus), Kaposi’s sarcoma (human herpesvirus type 8), and certain lymphomas (Epstein–Barr virus [EBV]) are now better understood as a long-term consequence of infection, making occupational health precautions even more important.
Viral infections are the most significant threat to HCWs. Most commonly, these are spread through the respiratory route. Other infections are spread by hand-to-hand transmission, and hand washing is the single most important protection strategy.71 Immunity against some viral pathogens, such as hepatitis B, can be provided through vaccination.72 Transmission of blood-borne pathogens such as hepatitis (HBV, HCV) and human immunodeficiency virus (HIV) can be prevented with mechanical barriers blocking portals of entry.73 Current recommendations from the Centers for Disease Control and Prevention (CDC) for preemployment screening, infection control practices, vaccination, postexposure treatment, and work restrictions for infected personnel should be consulted for specific information related to each pathogen.74,75,76
OSHA Standards, Standard Precautions, and Transmission-Based Precautions

Standard precautions include the appropriate application and use of hand washing, personal protective equipment (PPE), and respiratory hygiene/cough etiquette. The selection of specific barriers or PPE should be commensurate with the task being performed. Gloves may be all that is necessary during many procedures that involve contact with mucous membranes or oral fluids, such as during routine endotracheal intubation or during insertion of a peripheral intravenous catheter. However, additional personal protection, such as gown, mask, and face shield, may be required during endotracheal intubation when the patient has hematemesis or during bronchoscopy or endotracheal suctioning. Respiratory hygiene/cough etiquette has been added to standard precautions to prevent droplet transmission of respiratory pathogens, especially during seasonal outbreaks.
OSHA has promulgated standards to protect employees from occupational exposure to blood-borne pathogens.78 These standards require that there must be an exposure control plan specifically detailing the methods that the employer is providing to reduce employees’ risk of exposure to blood-borne pathogens. Among other requirements, the employer must encourage strategies to reduce blood exposures, furnish appropriate PPE (e.g., gloves, gowns), offer the HBV vaccine at no charge to personnel, and provide an annual educational program to inform employees of their risk for blood-borne infection.
Implementation of standard precautions and OSHA regulations has been effective in decreasing the number of exposure incidents that result in HCW contact with patient blood and body fluids.
The institution’s employee health service is required to obtain and record a contagious disease history from new employees and provide immunizations and annual purified protein derivative (PPD) skin testing. In addition, the employee health service must have protocols for dealing with workers exposed to contagious diseases and those percutaneously or mucosally exposed to the blood of patients infected with HIV or hepatitis B or C virus. Free consultation is available from the CDC Postexposure Prophylaxis Hotline (PEPline) at 1-888-HIV-4911. Protocols are also needed for dealing with caregivers who have common contagious diseases and for those who have less common but high-visibility public health problems.
Respiratory Viruses
Respiratory viruses account for half or more of all acute illnesses and are usually transmitted by one of two routes. First, small-particle aerosols from viruses such as influenza and measles are produced by coughing, sneezing, or talking and can be propelled over large distances. Second, large droplets that have been produced by coughing or sneezing can contaminate the donor’s hands or an inanimate surface. The virus is then transferred to the oral, nasal, or conjunctival mucous membranes of a susceptible person by self-inoculation. Rhinovirus and human respiratory syncytial virus (HRSV) are spread by this process.
Influenza Viruses
Influenza viruses, members of the Orthomyxoviridae family, are designated as type A, B, or C based on characteristics of the nucleoprotein (NP) and matrix (M) protein antigens. Influenza A viruses are further subtyped on the basis of the surface hemagglutinin (H) and neuraminidase (N) antigens. Individual strains are designated according to the site of origin, isolate number, year of isolation, and subtype, for example, influenza A/California/07/2009 (the infamous H1N1). Influenza A has 16 H subtypes and 9 N subtypes, of which only H1, H2, H3, N1, and N2 have
been associated with epidemics of disease in humans. Because influenza viruses are easily transmitted and are highly infectious, large outbreaks occur annually. Acutely ill patients shed virus for as long as 5 days after the onset of symptoms via small-particle aerosols. Because of their contact with nasopharyngeal secretions, anesthesiologists can easily become vectors in the spread of influenza virus in hospitals.
been associated with epidemics of disease in humans. Because influenza viruses are easily transmitted and are highly infectious, large outbreaks occur annually. Acutely ill patients shed virus for as long as 5 days after the onset of symptoms via small-particle aerosols. Because of their contact with nasopharyngeal secretions, anesthesiologists can easily become vectors in the spread of influenza virus in hospitals.
Table 3-4. Standard Precautions | ||||||||||||||||||||||||||||||||||||||||||||||||||||||||||||||||||||||||||||||||||||||||||||||||||
---|---|---|---|---|---|---|---|---|---|---|---|---|---|---|---|---|---|---|---|---|---|---|---|---|---|---|---|---|---|---|---|---|---|---|---|---|---|---|---|---|---|---|---|---|---|---|---|---|---|---|---|---|---|---|---|---|---|---|---|---|---|---|---|---|---|---|---|---|---|---|---|---|---|---|---|---|---|---|---|---|---|---|---|---|---|---|---|---|---|---|---|---|---|---|---|---|---|---|
|
Anesthesia personnel who routinely care for patients in high-risk groups should be immunized annually (October or November) with the inactivated (killed virus) influenza virus vaccine.76 There is evolving precedent to make this a condition of employment and medical staff credentialing.79 Available inactivated vaccines have been highly purified and are associated with few reactions. Since the vaccine used in the United States and many other countries is produced in eggs, individuals with true hypersensitivity to egg products either should be desensitized or should not be vaccinated. A live attenuated influenza vaccine, approved for use in healthy nonpregnant persons 2 to 49 years of age and administered by intranasal spray, is now available.
Influenza Pandemics
Influenza outbreaks occur almost every year, although their extent and severity vary widely. In the past century, there have been three influenza pandemics (1918, 1957, and 1968) with the “Great Influenza” in 1918 killing between 40 and 50 million people worldwide. The most recent pandemic emerged in March 2009 and was caused by an influenza A/H1N1 virus that rapidly spread worldwide over several months. The large number of infected patients strained local and global resources, such as a large requirement for respirators for personnel and ventilators for patients. Containment to prevent spread requires early identification and isolation of infected individuals to limit disease transmission. For patients requiring hospitalization, specific wards should be established with dedicated staff. NIOSH-certified respirators (N95 or higher) should be used by personnel during activities or procedures likely to generate infectious respiratory aerosols.
Avian Influenza A
In 1997, human cases of influenza caused by avian influenza viruses (A/H5N1) were detected in Hong Kong during an extensive outbreak of influenza in poultry. Between that time and February 2010, 478 cases of avian influenza in humans were reported in Asia and the Middle East. Nearly all of these cases were associated with contact with infected poultry. Efficient person-to-person transmission was not observed. Mortality rates have been high (60%). Because of the absence of widespread immunity to the H5, H7, and H9 viruses, concern persists that avian-to-human transmission might also contribute to the emergence of a pandemic strain.80 A vaccine for prophylaxis against avian influenza H5N1 was approved for use in the United States in 2007.
Human Respiratory Syncytial Virus
HRSV is the most common cause of serious bronchiolitis and lower respiratory tract disease in infants and young children worldwide. During periods when HRSV is prevalent in the community (usually late November through May in the United States), many hospitalized infants and children may carry the virus. Large amounts of virus are present in respiratory secretions of infected children, and although viable virus can be recovered for up to 6 hours on contaminated environmental surfaces, it is readily inactivated with soap and water and disinfectants. Infection of susceptible people occurs by self-inoculation when HRSV
in secretions is transferred to the hands, which then contact the mucous membranes of the eyes or nose.81 Although most children have been exposed to HRSV early in life, immunity is not permanent and reinfection is common.
in secretions is transferred to the hands, which then contact the mucous membranes of the eyes or nose.81 Although most children have been exposed to HRSV early in life, immunity is not permanent and reinfection is common.
HRSV may also be a significant cause of illness in healthy elderly patients and those with chronic cardiac or pulmonary disease.82 HRSV is shed for approximately 7 days after infection. Hospitalized patients with the virus should be isolated, but during seasonal outbreaks large numbers of patients may make isolation impractical.77 Careful hand washing and the use of standard precautions have been shown to reduce HRSV infection in hospital personnel.
Severe Acute Respiratory Syndrome (SARS)
SARS is a respiratory tract infection produced by SARS-associated coronavirus (SARS-CoV). SARS typically presents with a fever greater than 38.0°C followed by symptoms of headache, generalized aches, and cough. Severe pneumonia may lead to acute respiratory distress syndrome and death. The mechanisms of transmission of SARS are incompletely understood. Spread may occur by both large and small aerosols and perhaps by the fecal–oral route, as well as close person-to-person contact. Some ill individuals (“super-spreaders”) appear to be hyperinfectious and are capable of transmitting infection to as many as 40 contacts.83 The virus can also be spread when an individual touches a contaminated object and then inoculates the mouth, nose, or eyes. Aerosolization of respiratory secretions during coughing or endotracheal suctioning has been associated with transmission of the disease to HCWs, including anesthesiologists and critical care nurses.

Full access? Get Clinical Tree
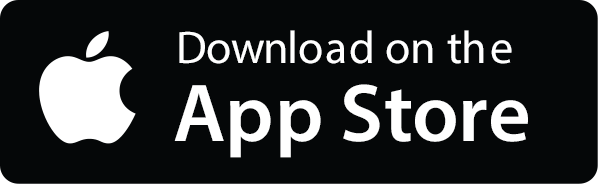
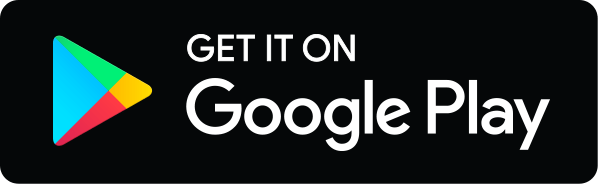
