Chapter 46
Obstetric Anesthesia
Anatomic and Physiologic Changes During Normal Pregnancy
Cardiovascular Changes
Cardiovascular changes begin as early as the fourth week of pregnancy and then continue into the postpartum period.1 The heart rate is increased by 20% to 30% at term. This increase begins in the first trimester and peaks by 32 weeks of gestation.2 Normal heart rate (HR) variability does not appear to be changed until late in pregnancy, when tachyarrhythmias are more common.3 Cardiac output increases by approximately 40% over nonpregnant values.4 This increase in cardiac output begins in the fifth week of pregnancy and results from an increase in stroke volume (SV) (20%-50%) and, to a lesser extent, heart rate.5 Cardiac output increases consistently throughout pregnancy.6 Some studies previously indicated cardiac output decreased in the third trimester, but these results were likely due to aortocaval compression from studying subjects in the supine position.7 At term, approximately 10% of the cardiac output perfuses the gravid uterus.8,9 When a woman is in labor, cardiac output increases during uterine contractions as a result of autotransfusion from the contracting uterus to the central circulation.10
Immediately after delivery, cardiac output increases as much as 80% above predator values as a result of an increase in central volume from the now contracted uterus and relief of aortocaval compression. As a result, patients with preexisting cardiac anomalies are at an increased risk for decompensation in the immediate postpartum period. Cardiac output gradually returns to baseline within 14 days as HR and SV normalize.11
During pregnancy the diaphragm rises, shifting the heart up and to the left, making the cardiac silhouette appear enlarged on x-ray examination. The ventricular walls thicken and end-diastolic volume increases. A physical examination of the pregnant patient may appear to elicit abnormal findings. A benign grade 1 or 2 systolic murmur or a third heart sound may be heard on auscultation.12 These findings would not be unusual; however, if the systolic murmur is greater than grade 3 or accompanied by chest pain or syncope, further evaluation is necessary. Diastolic murmurs and cardiac enlargement are considered pathologic. Normal pregnancy also may result in signs of cardiac abnormality such as exercise intolerance, shortness of breath, and edema.
Total blood volume increases 25% to 40% throughout pregnancy, in part to prepare for the normal blood loss associated with delivery. Plasma volume increases 40% to 50% whereas red blood cell (RBC) volume increases by only 20%.13 As a result, a relative or dilutional anemia is commonly seen as the plasma volume increases to a greater extent relative to the actual RBC volume. The increased plasma volume is likely the result of greater circulating levels of progesterone and estrogen resulting in enhanced renin-angiotensin-aldosterone activity.14 RBC volume increases as a result of elevated erythropoietin levels seen after the eighth week of gestation.15 The average blood loss for a vaginal delivery is 500 mL, and for an uncomplicated cesarean section, the blood loss is 800 to 1000 mL. Normal blood losses at delivery are generally well tolerated in the healthy parturient as a result of these compensatory mechanisms. In labor each contraction moves 300 to 500 mL of blood from the contracting uterus to the central circulation.16 Pregnant women have greater baroreflex-mediated changes in HR at term than at 6 to 8 weeks postpartum.17 In the presence of adequate neuraxial analgesia and little sympathetic stimulation, there is often a corresponding decrease in maternal heart rate during uterine contractions due to the transiently increased preload.
Systemic vascular resistance (SVR) decreases as much as 21% by the end of a term pregnancy, owing in large part to decreased resistance in the uteroplacental, pulmonary, renal, and cutaneous vascular beds.18,19 At term gestation 10% of the cardiac output perfuses the low resistance intervillous space of the uterus. Baseline central sympathetic outflow is twice as high in normal, term pregnant women as in nonpregnant women.20 The venous capacitance system loses tone, allowing pooling of the larger blood volume. This decrease in SVR results in little overall systolic blood pressure change during normal pregnancy, despite the increased blood volume.21 A decrease in diastolic blood pressure of up to 15 mmHg may occur, resulting in a decrease in mean pressure.
Aortocaval Compression
In the early 1950s a syndrome of supine hypotension was identified in term or near-term pregnant women.22,23 This syndrome is caused by compression of the vena cava by the gravid uterus, which restricts venous return to the heart when the parturient lies in the supine position. Compression can be more severe when the abdomen is tense or when the uterus is larger than normal, as in polyhydramnios or multiple gestation pregnancies. This decreased venous return results in a significant reduction in SV and, ultimately, cardiac output. The resultant hypotension can be severe enough to cause loss of consciousness in some women. Maximal decreases in blood pressure may require up to 10 minutes to develop; however, some women experience the decrease almost immediately. The normal physiologic responses to aortocaval compression are tachycardia and vasoconstriction of the lower extremities. Despite this attempted compensation, uterine blood flow and therefore fetal oxygenation are reduced.24 Figure 46-1 depicts changes in aortocaval compression with changes in position.
FIGURE 46-1 Effects of left uterine displacement on the diameter of the abdominal aorta and vena cava.
Compression of the aorta and vena cava can usually be relieved by shifting the uterus to the left (left uterine displacement) or by lying on the side.25 Left uterine displacement can be accomplished by rotation of the operating room table 15 degrees to the left or by placing a 15-cm-high wedge under the parturient’s right hip and back, as shown in Figure 46-1. Most anesthetists have been shown to underestimate the angle of tilt they provide, so care should be taken to ensure adequate left uterine displacement.26 In women with an exceptionally large uterus, greater displacement may be necessary to be effective. In a small percentage of women, right uterine displacement may be more effective than left displacement, although in the majority of cases, right uterine displacement results in stroke volumes that are no better than those measured with the patient in the supine position.
Neuraxial anesthetics result in an autonomic sympathectomy, thereby reducing compensatory lower-extremity vasoconstriction resulting in a greater potential for hypotension. There has been much debate regarding the optimal position for performing neuraxial anesthesia for labor analgesia and cesarean delivery. Many clinicians feel that the lateral position is optimal to facilitate both maternal and fetal hemodynamics. Researchers compared four positions: supine with a 15-degree left tilt, sitting with neck and hips flexed, and flexed left lateral and flexed right lateral positions. They found that maternal cardiac index (CI) was significantly higher in the right lateral position compared with the sitting and supine positions. It was also higher in the left lateral compared with sitting position. Maternal stroke volume index, heart rate, and systolic blood pressure were higher in the lateral positions compared with the sitting and supine-tilt positions. There were no significant differences in fetal heart rate, pulsatility index, or resistivity index among positions. Although positioning for neuraxial anesthesia may influence maternal hemodynamic variables, there were no difference in healthy fetal blood flow indices among positions, suggesting that these changes are not clinically significant.27
Hematologic Changes
In general, the parturient is said to be “hypercoagulable.” Levels of factors VII through X and fibrinogen are increased. In the nonpregnant state, fibrinogen levels average from 200 to 400 mg/dL. Late in pregnancy, fibrinogen levels are normally at least 400 mg/dL and may be as high as 650 mg/dL. These increased levels place the parturient at risk for thromboembolic events, which remain one of the leading causes of maternal mortality. The platelet count remains stable or is decreased slightly in the third trimester. The prevalence of maternal thrombocytopenia (platelet count less than 150 × 109/L) in normal pregnancy has been shown to be 11.6% 28 and is not associated with increased morbidity or mortality. The pathogenesis is not well understood but may involve factors such as hemodilution and/or accelerated platelet clearance.29,30 The white blood cell (WBC) count rises in pregnancy. In the third trimester, the mean is 10,500/mm3, and in labor the count may increase to 20,000 to 30,000/mm3.31
Respiratory Changes
The respiratory changes that accompany normal pregnancy are of particular importance to the anesthesia provider. Capillary engorgement in the upper airway results in a narrowed glottic opening and edema in the nasal and oral pharynx, larynx, and trachea. These changes carry over into labor, as has been shown by the Mallampati score changing as labor progresses.32 Airway tissues are friable and susceptible to damage and bleeding during placement of airway adjuncts. For this reason, nasal intubation in the parturient should generally be avoided. A 6.5- to 7.0-mm cuffed oral endotracheal tube is recommended when intubation is necessary. Obese patients with enlarged breasts may benefit from the use of a short-handled laryngoscope.
Term pregnancy is accompanied by an increase in oxygen (O2) consumption of up to 33% at rest and 100% or more during the second stage of labor. Minute ventilation at term is increased by 50%. This is primarily due to a 40% increase in tidal volume, whereas the respiratory rate is stable or increased by only 10%.33 By 12 weeks’ gestation the normal arterial partial pressure of carbon dioxide (CO2) decreases to approximately 30 to 32 mmHg, and remains in this range throughout pregnancy.34 Metabolic alkalosis is rarely seen because there is a compensatory decrease in the serum bicarbonate from 26 to 22 mEq/L. The normal arterial partial pressure of O2 is greater than 100 mmHg.
The functional residual capacity (FRC), expiratory reserve volume, and residual volume are decreased primarily as a result of upward pressure on the diaphragm, with results functionally similar to restrictive lung disease. The FRC plays an important role in preserving O2 saturation during periods of hypoventilation or apnea. The decrease in FRC (20%) combined with the increase in O2 consumption in pregnancy commonly results in rapid arterial desaturation in the apneic pregnant patient.35 Morbid obesity, labor, and sepsis exaggerate this effect.36 For this reason, pre-oxygenation with 100% O2 prior to induction of general anesthesia is important. Closing capacity (CC) does not change, which results in a decreased FRC/CC ratio often leading to small-airway closure before the tidal volume has been exhaled. This mechanism may explain the reduction in O2 saturations seen in parturients during natural sleep. When compared with nonpregnant controls, whose average oxygen saturation during sleep was 98.5%, healthy near-term pregnant women averaged only 95.2%, with temporary desaturations below 90% not being uncommon.37 The increased cardiac output and a shift to the right in the oxyhemoglobin dissociation curve help to maximize oxygen delivery.
Minute ventilation can increase up to 300% during contractions and cause maternal arterial partial pressure of CO2 to drop below 15 mmHg. This alkalemia can cause hypoventilation between contractions, resulting in hypoxemia. Some evidence indicates that hyperventilation may cause a decrease in uterine blood flow. However, in animal studies this finding has usually been associated with stressful events such as intubation and invasive procedures. In pregnant, laboring human volunteers, hyperventilation to an arterial partial pressure of CO2 of 20 mmHg does not appear to harm the fetus. Specifically, the fetus does not develop hypoxia or acidosis as determined by analysis of a scalp blood sample. However, in the presence of a complicated labor with preexisting fetal compromise, the effects could be detrimental.38
Nervous System Changes
Beginning in the first trimester pregnant women have an increased sensitivity to local and general anesthetics.39–42 The exact mechanism remains unclear, but animal studies have demonstrated a variable reduction in the minimum alveolar concentration (MAC) of inhalation agents in rabbits chronically exposed to progesterone.39 In pregnant rats, the effects of endorphins on pain thresholds are also demonstrated, which may also be an important factor. Additionally, research and clinical experience have demonstrated an increase in the sensitivity of nerves to local anesthetics during pregnancy.39–43 Mechanical changes within the epidural space also may play a role in the increased block height seen in pregnancy. Epidural veins become engorged as a result of increased intraabdominal pressure and cause a decrease in the volume of both the epidural and subarachnoid spaces.
Gastrointestinal Changes
The parturient is at increased risk for regurgitation and aspiration of gastric contents because of anatomic and physiologic changes associated with pregnancy. A significant number of pregnant women, and women immediately postpartum, have gastric volumes in excess of 25 mL and gastric pH below 2.5.44 Ultrasound has demonstrated solid food in the stomach of almost two thirds of women in whom neuraxial analgesia had been instituted and in the stomach of more than 40% of laboring women who had not eaten in 12 to 24 hours.45 Increased levels of gastrin during pregnancy result in greater gastric volume and lower pH. Upward displacement of the stomach by the gravid uterus may result in mechanical obstruction to outflow through the pylorus, delayed gastric emptying, and increased intragastric pressure. Elevated levels of progesterone, a smooth-muscle relaxant, also decrease gastric motility and cause a reduction in lower-esophageal sphincter tone. This explains the heartburn frequently experienced by pregnant women. Gastrointestinal changes do not completely normalize until several weeks postpartum.
The onset of labor is accompanied by a further reduction in the rate of gastric emptying in part due to pain and the use of parenteral opioids. Recently published practice guidelines allow for the modest amounts of clear fluids in uncomplicated laboring patients. Uncomplicated patients undergoing elective cesarean delivery may have modest amounts of clear liquids up to 2 hours prior to induction of anesthesia. The same guidelines state that patients with additional risk factors for aspiration (e.g., morbid obesity, diabetes, difficult airway), or patients at increased risk for operative delivery (e.g., nonreassuring fetal heart rate pattern) may have further restrictions of oral intake.46 Administration of nonparticulate antacids, H2 receptor antagonists (to decrease gastric pH), and metoclopramide (to promote gastric emptying, reduce nausea and vomiting, and increase lower esophageal sphincter tone) may be beneficial prior to anesthesia and should be considered. The use of these drugs is advocated when a general anesthetic becomes necessary. When a general anesthetic is used, a rapid sequence induction with cricoid pressure using a cuffed endotracheal tube is necessary from the twelfth week of gestation to the immediate postpartum period.
Hepatic Changes
During pregnancy, levels of aspartate aminotransferase, alanine aminotransferase, lactate dehydrogenase, and alkaline phosphatase increase to the upper limits of nonpregnant normal levels. Serum albumin concentration decreases somewhat, and this decrease may result in increased free fractions of highly protein-bound drugs. Serum cholinesterase activity decreases by 30% or more during the first or second trimester. Although activity recovers slightly by term, it remains reduced compared with prepregnant values. Despite these decreases in cholinesterase activity, clinically relevant prolongation of the duration of action of drugs that depend on cholinesterase for elimination, such as succinylcholine and remifentanil, is uncommon in women with genotypically normal cholinesterase enzymes.
Renal Changes
During pregnancy, increased cardiac output leads to increased renal plasma flow and increased glomerular filtration rate (GFR). Creatinine clearance rises to 140 to 160 mL/min. As a result, the level of blood urea nitrogen decreases to approximately 8 mg/dL and creatinine decreases to approximately 0.5 mg/dL. Urinary excretion of glucose is common in the absence of disease and is attributable to increased GFR and reduced renal absorption. The urinary excretion of protein in normal pregnancy is slightly elevated toward the upper limits of normal.47 The gravid uterus may occasionally produce mechanical obstruction of a ureter. Some key points to remember regarding physiologic changes in pregnancy are given in Box 46-1. A summary of the physiologic changes in pregnancy at term appears in Table 46-1.
Uterine Blood Flow
The fetus sends O2-poor blood to the placenta via two umbilical arteries. These vessels perfuse capillary networks within placental villi that protrude into the pool of maternal blood. Placental villi are small, fingerlike projections, the purpose of which is to maximize the placental surface area in contact with maternal blood. Each villus contains a capillary network that exchanges respiratory gases, nutrients, and wastes with maternal blood (Figure 46-2). Both O2 and CO2 diffuse through placental tissue readily and are considered to be “perfusion limited,” meaning their transfer to the fetus is limited only by the perfusion of the placenta, not by the rate of diffusion of the gases. Therefore, decreases in maternal uterine artery blood flow or increases in placental vascular resistance decrease fetal oxygenation.
Autoregulation of intervillous blood flow does not seem to occur. The spiral arteries, however, do constrict in response to α-agonists (e.g., phenylephrine). However, recent human studies indicate phenylephrine does not appear to be harmful to the fetus when used at clinically relevant doses.48 Patients with preeclampsia (formerly called pregnancy-induced hypertension, or PIH) can develop placental insufficiency at systolic pressures greater than 100 mmHg. Unlike patients who receive neuraxial anesthesia, patients receiving inhalation anesthesia seem to maintain adequate placental blood flow, despite somewhat reduced blood pressure; this may be a function of altered uterine blood flow, altered fetal O2 requirements, or both.
Placental Transfer and Fetal Effects of Drugs
The acid-base status of the fetus may affect the accumulation of a drug. A fetus who has become acidotic will alter the degree of ionization of a drug, potentially resulting in “ion trapping” leading to accumulation. For a complete discussion of fetal ion trapping see Chapter 10.
Labor and Delivery
First-stage labor pain is primarily the result of cervical distension, stretching of the lower uterine segment, and possibly, myometrial ischemia. The resultant nonspecific nociceptor visceral stimulation is carried to the central nervous system by afferent unmyelinated C fibers that enter the cord at the T10, T11, T12, and L1 segments (Table 46-2 and Figure 46-3). Thus the pain of first-stage labor is frequently described as nonlocalized aching or cramping. Second-stage labor begins when cervical dilation is complete and the presenting part descends into the pelvis. At this time, a second pain pathway becomes important. Compression and stretching of the pelvic musculature and perineum produce pain that is mediated by somatic afferent fibers carried via the pudendal nerves that enter the spinal cord at the S2, S3, and S4 levels. A neuraxial anesthetic that extends from T10 to the sacral nerve roots can be effective in relieving the pain of both first- and second-stage labor.
TABLE 46-2
Area | Innervation | Comments |
Uterus and cervix | T10 to L1-L2 | Pain impulses carried in visceral afferent type C fibers |
Perineum | S2, S3, and S4 | Pain impulses carried by somatic nerve fibers; pudendal nerves |
The experience of pain is a highly personal phenomenon, and pain tolerance varies widely from one individual to the next. Many factors outside a parturient’s control are also related to the perception of pain; these range from the presence or absence of social support to physical factors such as fetal presentation. Labor and vaginal delivery can be described as a process in which three essential components must work in concert. First, the fetus must be properly positioned and of an appropriate size to ensure passage through the bony pelvis, dilating cervix, and vagina. Second, the uterus must contract regularly and effectively. Third, the pelvic outlet itself must be configured in such a way that the fetus can pass. A flaw in any of these three elements can result in a difficult, painful, and prolonged labor. A few women are able to tolerate labor and delivery without significant discomfort; others experience pain in excess of their ability to cope. When women choose labor analgesia, it can make the birth process more enjoyable and provide the opportunity to better control their bodies and environment, thereby allowing them to maintain personal dignity.
Stages of Labor and Delivery
Labor is defined as progressive dilation of the cervix in response to uterine contractions. Although sporadic and irregular third-trimester contractions are common, labor is not said to begin until those contractions are regular and result in a change to the cervix. Labor is generally divided into three stages. First-stage cervical changes consist of effacement and dilation. The first stage is further divided into at least the latent and active phases. The latent phase is of variable duration and is defined as the period between the onset of labor and the point at which the cervix begins to rapidly change.49 Active phase usually begins at 2 to 3 cm dilation and is the period during which the cervix undergoes its maximum rate of dilation.
The second stage begins at full cervical dilation (10 cm) and ends with delivery of the fetus. The third stage encompasses the delivery of the placenta. The length of time it takes to progress through these stages is dependent on parity, effective uterine contractions, the size and type of pelvis, and fetal presentation. The Friedman curve is used to track the normal progress of labor (Figure 46-4). Cervical dilation normally progresses 1 to 1.2 cm/hr during the active phase of labor. When labor progress no longer follows the normal pattern, it is considered a “dysfunctional labor,” and may require the use of oxytocin to augment contractions.
Intrapartum Fetal Evaluation
Intrapartum fetal heart rate (FHR) monitoring is now used routinely in the United States. Although continuous or intermittent FHR monitoring is not a specific predictor of fetal well-being, it is the most readily available method for the assessment of fetal condition. Intermittent monitoring can be performed using a manual stethoscope known as a fetoscope. Continuous FHR monitoring is accomplished using either external Doppler ultrasonography or an internal fetal electrocardiography electrode. The internal method requires ruptured amniotic membranes and a partially dilated cervix through which the electrode can be inserted. The continuous FHR is recorded in a two-channel format with the FHR displayed directly above a graphic representation of the uterine contractions. In this way it is possible to relate FHR changes to uterine activity. The most commonly used scaling is a paper speed of 3 cm/min on the horizontal axis and 30 beats/min per centimeter of paper for the FHR (vertical axis).50 In this manner each sequential vertical line is 10 seconds apart and every sixth vertical line (bold) represents a period of 1 minute.
Uterine contractions are monitored either externally via a tocodynamometer or internally with an intrauterine pressure catheter attached to a transducer placed between the fetus and uterine wall. Data obtained from external contraction monitoring is limited to temporal elements such as contraction duration and interval and cannot be used to estimate contraction strength. External monitoring is also subject to a great deal of artifact from maternal movement and errors in transducer placement. Internal contraction monitoring is more reliable and allows for precise determination of intrauterine pressure and, hence, contraction strength. Its use is also predicated on ruptured amniotic membranes and a partially dilated cervix. Internal pressure monitoring is commonly seen with high-risk parturients or those in whom labor augmentation with oxytocin is being used. Most commercially available intrauterine pressure catheter systems also allow for the infusion of fluids into the amniotic space (amnioinfusion), which is thought to reduce the risk of fetal aspiration of meconium-stained amniotic fluid.51
Fetal pulse oximetry has been introduced recently but is largely investigational at this time. A wide range in normal values has been reported, and use of oximetry has not been shown to decrease overall cesarean section rates, making its ultimate benefit questionable.52
Information about the baseline status of a fetus should be obtained during the preanesthetic assessment. The FHR also can reveal information about the fetal response to anesthetic interventions. This information is useful before neuraxial anesthetic placement, for assisted or operative delivery, and for nonobstetric surgery during pregnancy. Current American Association of Nurse Anesthetists (AANA) practice guidelines state that the Certified Registered Nurse Anesthetist (CRNA) shall be aware of the fetal status prior to each anesthetic intervention and that the fetal status be monitored and documented in the patient’s record.53
Changes in Fetal Heart Rate
The individual components of the FHR pattern do not occur alone and changes generally evolve over time. Therefore, a full description of a FHR tracing requires a qualitative and quantitative description of baseline rate, variability, presence of accelerations or decelerations, and trends over time.54 In the presence of a normally functioning uteroplacental unit, fetal oxygenation is limited primarily by uterine blood flow. As stated before, uterine arteries are maximally dilated in pregnancy, which results in the inability of the uterus to autoregulate blood flow. Decreases in maternal blood pressure and uterine artery blood flow ultimately compromise placental blood flow, resulting in fetal hypoxia and acidosis, which can be manifested as fetal heart rate changes. The normal FHR ranges from 110 to 160 beats per minute (bpm). An immature fetus has a higher heart rate. Tachycardia is defined as a HR greater than 160 bpm in a term fetus. It can result from fetal asphyxia, fetal arrhythmias, maternal fever, chorioamnionitis, and maternally administered drugs such as terbutaline and atropine. Ephedrine can increase both FHR and variability if given to the parturient in large enough doses.55 Fetal bradycardia is present when the FHR is less than 110 bpm. Bradycardia can be caused by maternally administered drugs, hypoxia (fetal or maternal), or fetal head or cord compression.
Variability
FHR variability is thought to be the single best indicator of fetal well-being because it likely indicates adequate fetal oxygen reserve. Variability is the result of interaction between the fetal sympathetic and parasympathetic autonomic nervous systems. Baseline variability is defined as fluctuations in the FHR of two cycles per minute or greater that are irregular in amplitude and frequency.54 It is quantified by measuring the amplitude from peak to trough in beats per minute. Variability is described as absent, minimal (less than 5 bpm), moderate (6-25 bpm), and marked (greater than 25 bpm). In general, baseline FHR variability increases with advancing gestational age. Variability represents an intact central nervous system (CNS) and normal cardiac function. Hypoxia causes CNS depression, which results in decreased variability. Other causes of decreased variability include fetal sleep, acidosis, anencephaly, drugs (CNS depressants or autonomic agents), and defects of the fetal cardiac conduction system. Administration of opioids to the mother, for example, can decreases FHR variability for up to 30 minutes.56 Maternal magnesium sulfate administration also may attenuate FHR variability.57 Variability refers to the baseline FHR and, as such, does not include accelerations or decelerations. Beat-to-beat variability can be accurately assessed only by direct FHR monitoring with a fetal scalp electrode. Examples of absent and moderate FHR variability are shown in Figure 46-5.
Accelerations and Decelerations
Early Decelerations
Late Decelerations
Late decelerations begin late in the contraction and represent uteroplacental insufficiency. The lowest point of the deceleration occurs after the peak of the contraction. Like early decelerations, they are smooth in both onset and recovery. Late decelerations are normally repetitive. Beat-to-beat variability may or may not be present during the deceleration, depending on the degree of fetal hypoxia and myocardial depression. Late decelerations are nonreassuring and should be investigated for potential causes. Figure 46-6 shows a FHT with late decelerations and minimal variability.
Late decelerations have the following characteristics:
Analgesia For Labor and Vaginal Delivery
Intravenous Analgesia in the Parturient
Although less effective than neuraxial analgesia, systemic medications can be used for labor pain relief when neuraxial analgesia is unavailable, refused, or contraindicated. From an anesthesia perspective, the use of systemic medications presents several disadvantages. The pain relief they afford is often inadequate, and both fetal and maternal respiratory depression, nausea, vomiting, and decreased lower esophageal sphincter tone may result. Controlled, randomized trials investigating neuraxial versus intravenous analgesia in labor are difficult to design and execute due to high protocol failure rates. Namely, large numbers of parturients cross over from the IV to the neuraxial groups. However, when performed, overall patient satisfaction and pain relief scores are higher in women who receive neuraxial anesthetics compared with those who receive intravenous analgesia.58,59
Because opioids are lipid soluble and relatively small (less than 500 Da), they rapidly cross the placenta to gain access to the fetal circulation. Opioids may be administered by IV bolus or patient-controlled analgesia (PCA). The advantage of PCA is it allows the parturient greater control over drug dosing and has resulted in greater patient satisfaction. However, in one study PCA was compared with nurse-administered boluses, and there was no difference in pain scores or maternal and fetal side effects.60 The use of systemic medications in early labor has declined as the practice of obstetric anesthesia has evolved to include those patients in early labor as candidates for neuraxial analgesia. Current American College of Obstetrics and Gynecology (ACOG) guidelines state that maternal request is a sufficient reason for labor analgesia.61
Meperidine
Meperidine crosses the placenta easily and has been recovered from the fetus within 2 minutes of IV administration. Like all opioids, it is capable of causing neonatal respiratory depression, although less so than morphine or methadone. Because of differences in pH and protein binding, the level of meperidine in the fetus is likely to be higher than the maternal blood level. Normeperidine is an active metabolite of meperidine with an elimination half-life of 30 hours. Normeperidine remains in the neonate for several days after delivery and may lead to depression of neonatal behavioral assessment scores.62
Fentanyl
The pharmacologic profile of fentanyl, that is, its high potency and short duration of action, make it a reasonable choice for labor. It is highly lipid soluble and can be detected in fetal circulation after 1 minute of IV administration. Depressant effects, including a reduction in beat-to-beat variability, have been seen.63 Dosages for labor range from 25 to 100 mcg IV in hourly increments.64 or PCA bolus 25-50 mcg with a lockout interval of 3 to 6 minutes and a 4-hour limit of 1-1.5 mg.63,65
Morphine
Morphine has been used in early labor to provide analgesia and sedation but is no longer widely accepted due to high rates of maternal sedation, neonatal depression, and an undesirable prolonged duration.66 Morphine crosses the placenta easily and, in animal studies, crosses the fetal blood-brain barrier more readily than in adults.67
Butorphanol and Nalbuphine
Butorphanol and nalbuphine are opioid agonist-antagonists that are associated with a “ceiling effect” whereby incrementally higher doses do not result in increasing respiratory depression. They may also result in less nausea and vomiting than pure opioids. Agonist-antagonists have typically been used in first-stage latent-phase labor to provide sedation and a period of rest. A typical butorphanol dose for labor is 1 to 2 mg IV or IM (intramuscularly). Butorphanol is five times as potent as morphine and has a half-life of 3 hours. Unlike morphine, butorphanol increases pulmonary artery pressure and myocardial work.64 Butorphanol has no active metabolites. The nalbuphine dose in labor is 5 to 10 mg IV, IM, or SC (subcutaneous) and is equivalent to morphine 10 mg. Nalbuphine results in a greater reduction in FHR variability compared with meperidine.68 Because these compounds possess significant antagonist properties, their use in opioid-dependent patients should be avoided.
Remifentanil
Remifentanil is an ultra–short-acting opioid receptor agonist. It is rapidly hydrolyzed by plasma esterases to an inactive metabolite. The context-sensitive half-life remains short (3.2 min) regardless of prolonged administration times.69 This unique pharmacokinetic profile makes it suitable for PCA use in labor. It crosses the placenta readily but is similarly rapidly redistributed and metabolized by the fetus.70 Douma et al.71 compared remifentanil, fentanyl, and meperidine PCA in labor and found that remifentanil PCA was associated with the greatest reduction in pain scores; however, the relief was described as mild to moderate. The same group has found that remifentanil PCA is less effective when compared with standard epidural labor analgesia.72 A PCA bolus of 0.25 mcg/kg with a lockout interval of 2 minutes, a 4-hour limit of 3 mg, and a background infusion of 0.025-0.05 mcg/kg/min is common.63,73,74 Remifentanil use in labor is a relatively new technique that appears to have an acceptable safety profile. However, its use requires careful monitoring due to the potential for adverse maternal events.75
Ketamine
A single induction dose of 1 mg/kg of ketamine increases blood pressure during induction. This may be desirable in an emergent cesarean section where the mother is hypovolemic. The hypertensive effects, however, may be problematic in the parturient with hypertension or preeclampsia. It is the agent of choice for induction in the patient with acute asthma requiring urgent cesarean section. The emergence delirium and hallucinations from ketamine limit the effectiveness of ketamine for routine use in obstetrics. Uterine arterial blood flow does not decrease after ketamine administration. Because it has a high lipid solubility, ketamine crosses the placenta easily. Neonatal depression has not been demonstrated after maternal ketamine doses of up to 1 mg/kg. Doses larger than 1 mg/kg may result in neonatal respiratory depression, muscular hypertonicity, and lower Apgar scores.76
Nonpharmacologic Methods
A parturient may very well not choose a neuraxial anesthetic for labor analgesia if that is her informed choice. A multitude of nonpharmacologic techniques are currently in use but, as Wong has stated, the effectiveness of these techniques generally lacks rigorous scientific study and conclusions regarding their efficacy cannot be made.77

Full access? Get Clinical Tree
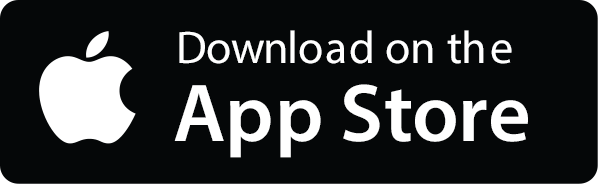
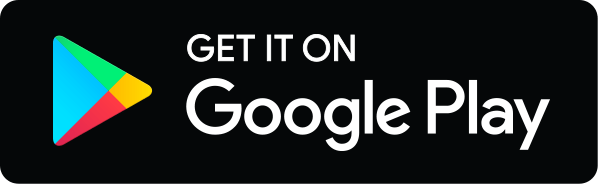