Severely injured patients, burn patients, and those with sepsis often are hypermetabolic, so directed nutritional support within 24 to 48 hours of admission may be beneficial. For example, energy requirements may double and protein requirements may triple in severely burned patients. Conversely, the increase in basal metabolic rate that occurs during and soon after major uncomplicated elective surgery is less than 10%, so that providing glucose solutions (~500 kcal per day) in the postoperative period is sufficient, and further nutritional support does not improve outcome.
Minimally stressed patients require about 25 to 30 cal/kg and 1 g/kg of protein daily to remain in nitrogen and energy equilibrium. Moderately to severely stressed patients should be resuscitated first and then started on a hypocaloric regimen (20 cal/kg) until the stress response abates. Lipid calories from infusions of propofol may be significant and should be included when calculating caloric intake.9
Enteral Nutrition
Unless contraindicated (e.g., short gut syndrome, circulatory shock10), enteral nutrition is preferred over parenteral nutrition in almost every circumstance for the reasons mentioned earlier. Three decades ago, it was thought that the main goal of nutrition support in the hospitalized patient was to meet energy requirements and to make the patient anabolic. Current goals include meeting and attenuating the metabolic response to stress and, in addition, attenuating cellular injury and modulating the immune response to injury. Nutritional support of the moderately to severely injured patient includes enteral nutrition started sooner rather than later, pharmacotherapy (the provision of nutrients that modulate the body’s response to injury) and glycemic control. Delivering early nutrition support, primarily using the enteral route, is seen as a proactive therapeutic strategy that may reduce disease severity, diminish complications, decrease length of stay in the ICU, and favorably impact patient outcome after severe injury. A variety of enteral solutions containing various amounts of protein (amino acids), carbohydrates (glucose), fat (medium- and long-chain triglycerides), micronutrients, macronutrients, and electrolytes are available. No single formulation has been found to be ideal for all patients. Carbohydrates can be the source of up to 90% of the calories, which increases the osmolarity of these solutions. Fat has a higher caloric density than carbohydrates, and because it does not increase the osmolarity of the formula as much as carbohydrates, iso-osmolar solutions can be constituted. Unless the patient has maldigestion or malabsorption of fat (and even then a formula containing medium-chain triglycerides can be tried), formulas with a normal range of fat content (~30%) are preferred. Selection of a formula that provides sufficient total nitrogen as protein (1 to 1.5 g protein per kilogram per day) or amino acids is essential for all patients. It was once thought that low-protein formulations were indicated for patients with severe renal dysfunction; however, we now recognize that these patients require the same amount of protein as do other patients, even if one has to resort to dialysis to maintain homeostasis.11 Specialized formulas are available for nutritional deficiencies associated with renal disease, but they are rarely indicated.12 The same can be said for patients with liver disease—standard enteral formulas work well. The only exception is the patient with hepatic encephalopathy for whom an enteral or parenteral formula containing branched-chain amino acids may improve the encephalopathy, but if not, one should change back to a standardized formula.13 Increased amounts of protein are indicated when the nitrogen requirement is increased, as in patients with trauma, burns, or sepsis. The efficient use of protein for anabolism depends on adequate caloric intake. Enteral formulas containing glutamine, specifically when administered to patients with burns, reduce hospital and ICU length of stay, primarily through a reduction in infection.14,15
Enteral Tube Feeding
Enteral tube feeding may be necessary when patients are unable to consume nutritionally complete, liquefied food orally. Commercial formulations of natural foods can be so finely suspended that they pass through small-bore tubes. Defined-formula diets are necessary when luminal hydrolysis or absorption is impaired, as in malabsorption syndromes. An important consideration when utilizing enteral nutrition is placement and positioning of the small-bore (8 to 12 French) silastic delivery tube. Most often, patients receive continuous infusions of enteral nutrition through a nasoenteric tube positioned in the stomach, duodenum, or jejunum. Several groups of investigators have studied whether there is a clinical significance between gastric versus postpyloric feeding in various medical and surgical ICU settings. Two meta-analyses of these studies did not show a difference in the incidence of pneumonia whether the feeding tube was in the stomach or through the pylorus, nor was there a difference in mortality based on the position of the feeding tube; however, a contrary finding by Taylor was not included.16–18
Surgical placement of an esophagostomy or gastrostomy tube may be indicated for long-term feeding. For continuous enteral feeding, an automated infusion pump to control the rate of administration of the nutritional formula is useful. Indeed, absorption and tolerance are improved and the incidence of side effects is decreased by slow constant feeding over several hours. The rate of infusion is typically 100 to 120 mL per hour. This slow rate of infusion prevents the dumping syndrome, which may occur when hyperosmolar solutions are introduced rapidly into the small intestine.
Side Effects
Enteral feeding is frequently stopped because of patient’s complaints of bloating or distention; emesis; high gastric residuals (usually 200 to 250 mL); diarrhea; distended abdomen on physical exam; reduced passage, or absence, of flatus; or abnormal findings on abdominal radiographs. With the exception of high gastric residuals and diarrhea, these are legimate criteria for interrupting enteral feedings. One should not interrupt enteral feedings for gastric residual volumes of less than 500 mL, in the absence of other symptoms or signs of intolerance.19 The presence of diarrhea is always a concern, but one should consider alternative explanations before deciding that the diarrhea is caused by the osmolarity of the enteral product. Even if it is, osmotic diarrhea is relatively benign and short lasting.20 Osmotic diarrhea in this situation is a diagnosis of exlusion, and one must try to identify other causes such as enteral medications containing sorbitol, Clostridium difficile, or other infection, by performing an abdominal exam, sending stool for an assessment for fecal leukocytes, stool culture, and toxin assay.21 If clinically indicated, serum electrolyte levels should be measured to identify excessive loss or signs of dehydration.
Pulmonary aspiration is always a danger when enteral tube feeding is used. Patients should be maintained in a semi-sitting position (head of bed elevated 30 degrees) and, in patients at the highest risk of aspiration, the feeding tube should be placed through the pylorus. Preparations containing large amounts of electrolytes should be administered cautiously to patients with cardiovascular, renal, or hepatic disease. Many commercial formulas contain large amounts of sodium. Dry preparations mixed with water are excellent culture media unless they are kept sterile and refrigerated.
Parenteral Nutrition
Parenteral nutrition is indicated for patients who are unable to ingest or digest nutrients or to absorb them from the gastrointestinal tract. Parenteral nutrition using isotonic solutions delivered through a peripheral vein is acceptable when the patient requires less than 2,000 calories daily and the anticipated need for nutritional support is brief. Peripheral veins do not tolerate infusion of solutions with an osmolarity that exceeds 750 mOsm/L (equivalent to 12.5% glucose) thus limiting the number of calories that can be administered. When nutritional requirements are greater than 2,000 calories daily or prolonged nutritional support is required, a catheter is placed in the central venous system to permit infusion of a hypertonic (1,900 mOsm/L) nutrition solution.
Short-Term Parenteral Therapy
Short-term parenteral therapy (3 to 5 days in patients without nutritional deficits) after uncomplicated surgical procedures is most often provided by hypocaloric, non-nitrogen glucose-electrolyte solutions. For example, glucose solutions, 5% to 10%, with supplemental sodium, chloride, and other electrolytes are commonly administered for short-term therapy. These solutions provide total fluid and electrolyte needs and sufficient calories to decrease protein catabolism and prevent ketosis. For example, daily infusion of approximately 150 g of glucose maintains brain and erythrocyte metabolism and decreases protein catabolism from skeletal muscles and viscera.
Amino acids may have a greater protein-sparing effect than glucose, but amino acids without glucose do not completely prevent negative nitrogen balance after major surgery. The higher cost of amino acid solutions relative to potential benefit has prevented their popularity for use in place of glucose for short-term therapy.
Peripheral infusion of fat emulsions may be administered as a nonprotein source of calories to augment those supplied by glucose.
Long-Term TPN
TPN (IV hyperalimentation) is the technique of providing total nutrition needs by infusion of amino acids combined with glucose and varying amounts of lipids. Lean body mass is preserved, wound healing may be enhanced, and there may even be improvement of an impaired immune response mechanism.
TPN solutions contain a large proportion of calories from glucose and thus are hypertonic. For this reason, these solutions must be infused into a central vein with a high blood flow to provide rapid dilution. A catheter is often placed percutaneously into the subclavian vein and guided into the right atrium. The parenteral nutrition solution is usually infused continuously over 24 hours. Because the solutions in current use are not nearly as hypertonic and hypercaloric as they once were, there is little concern about the patient becoming hypoglycemic if the infusion is discontinued abruptly but should be considered.
Serum electrolytes, blood glucose concentrations, and blood urea nitrogen should be measured periodically during TPN. Tests of hepatic and renal function are also recommended but can be performed at less frequent intervals.
Side Effects
The side effects of TPN include infectious, mechanical, and metabolic complications. Amongst infectious complications, catheter-related sepsis is one of the most common and associated with significant morbidity. The mechanical complications such as pneumothorax and thrombosis if the catheter is left in place for extended periods, are complications related to the placement of a central line and with which anesthesiologists are familiar. There are a number of metabolic complications seen more often with parenteral nutrition than with enteral nutrition (Table 36-2).

Sepsis
TPN solutions infused through an IV catheter can support the growth of bacteria and fungi. A spiking temperature most likely reflects contamination via the delivery system or catheter. The catheter should be removed and the tip cultured to determine the appropriate antibiotic therapy. In view of the hazard of contamination, the use of a central venous hyperalimentation catheter for administration of medications, as during the perioperative period, or for sampling of blood is not recommended.
Fatty Acid Deficiency
Fatty acid deficiency may develop during prolonged TPN but only if no intralipid is administered as part of the 3-in-1 formulation (protein, glucose, lipid). Possible immunosuppressive effects of lipid emulsions and an increased incidence of infections has led to recommendations to limit fat calories to about 30% of total TPN calories.22
Hyperglycemia
Blood glucose concentrations should be monitored until glucose tolerance is demonstrated, which usually occurs after 2 to 3 days of therapy as endogenous insulin production increases. In addition, blood glucose concentrations should be periodically monitored during the perioperative period in patients maintained on TPN. The degree of hyperglycemia accompanying TPN is directly related to the rate of glucose infusion, and to the degree of stress. A 2001 study demonstrated improved outcomes in patients with hyperglycemia in whom blood glucose levels were kept below 110 mg/dL with intensive insulin therapy23; however, subsequent studies failed to confirm the original findings.24 Current guidelines suggest a target of 140 to 200 mg/dL and avoidance of targets below 140 mg/dL.25
Hypoglycemia
Accidental sudden discontinuation of the infusion of TPN solutions containing large amounts of glucose (catheter kink or disconnection) may cause hypoglycemia. Indeed, TPN infusion should be discontinued gradually over 60 to 90 minutes. Hypoglycemia occurs because the pancreatic insulin response does not always cease in parallel with discontinuation of the parenteral nutrition solution. As a result, a high plasma concentration of insulin may persist in the absence of continued infusion of glucose. If administration of the TPN solution must be stopped abruptly, exogenous glucose should be infused for up to 90 minutes to prevent hypoglycemia. The incidence of hypoglycemia has decreased because clinicans have a lower daily caloric goal (e.g., 1,400 to 2,000 kcal per day) compared to prior therapies (3,000 to 4,000 kcal per day).26
Hepatobiliary Complications
Excessive caloric intake is associated with hepatic steatosis and steatohepatitis. An increased alkaline phosphatase or serum bilirubin concentrations warrant additional evaluation (e.g., cholehepatic ultrasound).
Metabolic Acidosis
Hyperchloremic metabolic acidosis may occur because most of the amino acids in TPN are administered as their chloride salts.
Hypercarbia
In a patient with inadequate respiratory reserve, respiratoy failure can develop with aggressive nutritional support that increases carbon dioxide production. Because glucose has a respiratory quotient of 1, excessive glucose was blamed on the respiratory failure associated with TPN, but we now know that excessive calories per se independent of their source increases carbon dioxide production and leads to respiratoy failure in susceptible patients.27
Monitoring during TPN
Acutely ill patients receiving TPN must be followed closely for the development of treatment-related complications. Access sites are observed for signs of infection. Substitution of sodium or potassium acetate (metabolized to bicarbonate) for sodium or potassium chloride may be helpful should signs of hyperchloric metabolic acidosis appear. Plasma triglyceride concentrations may increase in patients with diabetes mellitus, sepsis, and impaired hepatic or renal function. Vitamin K may need to be added to the TPN or administered intravenously based on measurement of prothrombin and plasma thromboplastin times. Monitoring of daily caloric intake, to ensure that caloric goals are being met, and fluid intake and output is needed as patients who are critically ill often experience significant fluid shifts.
Preparation of TPN Solutions
TPN solutions are prepared from commercially available solutions by mixing hypertonic glucose with an amino acid solution. Sodium, potassium, phosphorus, calcium, magnesium, and chloride are added to TPN solutions. Trace elements including zinc, copper, manganese, chromium, and selenium must also be added if the need for parenteral therapy is prolonged. Requirements for vitamins may be increased, emphasizing the need to add a multivitamin preparation to TPN solutions. Vitamin B12 and folic acid may be administered as components of a multivitamin preparation or separately. Vitamin D should be used sparingly because metabolic bone diseases may be associated with use of this vitamin in some patients on long-term parenteral nutrition. Vitamin K can be administered separately once every week. The U.S. Food and Drug Administration (FDA) disallowed routine addition of vitamin K to TPN because of concern about side effects, and its routine administration would complicate the use of anticoagulants such as warfarin in patients who require such therapy. The serum albumin concentration will usually increase over several days to weeks as the stress response abates and if patients receive adequate nutrition support. The administration of supplemental albumin is not necessary in the absence of symptoms or signs of hypoalbuminemia, which usually do not occur until the serum albumin concentration is less than 2.4 g/dL.
Fat emulsions (Intralipid) can be administered separately or together with the glucose and amino acids to create a 3-in-1 TPN solution, as mentioned previously. To decrease the possibility of bacterial contamination, TPN solutions are prepared aseptically under a laminar air-flow hood, refrigerated, and administered within 24 to 48 hours.
Immunonutrition
Cellular immunity decreases during acute stress, as may accompany multiple organ system failure, sepsis, and shock. Immunonutrition is an attempt to enhance immunity and cellular integrity by incorporating specific additives (omega-3 fatty acids, arginine to enhance lymphocyte cytotoxicity, purines as a precursor of RNA and DNA and antioxidants) into enteral diets. Currently, there are no well-controlled clinical studies that demonstrate improved outcomes with immunonutrition in patient populations that might benefit from their use, and there are no clinical guidelines that suggest their routine use in these patient populations.
Vitamins, Dietary Supplements, and Herbal Remedies
Vitamins
Vitamins are a group of structurally diverse organic substances (water soluble or fat soluble) that must be provided in small amounts in the diet for subsequent synthesis of cofactors that are essential for various metabolic reactions (Table 36-3). Food is the best source of vitamins, and healthy persons consuming an adequate balanced diet will not benefit from supplemental vitamins. Nevertheless, many persons do not consume adequate amounts of vitamin-rich foods, especially patients with alcoholism and malabsorbtion syndromes, the elderly, and the economically disadvantaged.

Antioxidant vitamins can retard atherogenesis, and antioxidants may lower the risk of carcinogenesis. There is a demonstrated relationship between low dietary intake of antioxidants or low plasma concentrations of antioxidants and an increased risk of atherosclerosis and cancer. Studies have linked low plasma concentrations of folic acid, vitamin B6, and vitamin B12 with increased plasma concentrations of homocysteine and increased cardiovascular risks.28A vitamin supplement that combines antioxidants with zinc can slow progression of macular degneration. Individuals who consume multivitamins appear to have a decreased risk of cardiovascular disease and colon cancer, which may represent protection from folic acid and the B vitamins.29
It is clear that additional information and studies are necessary to clarify the need for vitamin supplements in the presence of an adequate diet. The present recommendation is for parturients, the elderly, and those individuals receiving a suboptimal nutritional diet to take a single multivitamin tablet daily. Strict vegetarians should take vitamin B12 supplements.
Use of megadose vitamin preparations is not encouraged. Brand name and so-called all-natural preparations are no more effective than generic vitamin preparations. Regardless, vitamin supplements should never be used as a substitute for a balanced healthful diet that provides abundant quantities of vitamin-rich foods.
Water-Soluble Vitamins
Water-soluble vitamins include members of the vitamin B complex (thiamine, riboflavin, nicotinic acid, pyridoxine, pantothenic acid, biotin, cyanocobalamin, folic acid) and ascorbic acid (vitamin C) (Fig. 36-1).

Thiamine
Thiamine (Vitamin B1) is converted to a physiologically active coenzyme known as thiamine pyrophosphate. This coenzyme is essential for the decarboxylation of α-keto acids such as pyruvate and in the use of pentose in the hexose-monophosphate shunt pathway. Indeed, increased plasma concentrations of pyruvate are a diagnostic sign of thiamine deficiency.
Causes of Deficiency
The requirement for thiamine is related to the metabolic rate and is greatest when carbohydrate is the source of energy. This is important in patients maintained by hyperalimentation in which the majority of calories are provided in the form of glucose. Such patients should receive supplemental amounts of thiamine. Thiamine requirements are also increased during pregnancy and lactation30 and in patients with chronic alcoholism.31
Symptoms of Deficiency
Symptoms of mild thiamine deficiency (beriberi) include loss of appetite, skeletal muscle weakness, a tendency to develop peripheral edema, decreased systemic blood pressure, and low body temperature. Severe thiamine deficiency (Korsakoff syndrome), which may occur in alcoholics, is associated with peripheral polyneuritis, including areas of hyperesthesia and anesthesia of the legs, impairment of memory, and encephalopathy. High-output cardiac failure with extensive peripheral edema reflecting hypoproteinemia is often prominent. There is flattening or inversion of the T-wave prolongation of the QTc interval on the electrocardiogram (ECG).
Treatment of Deficiency
Severe thiamine deficiency is treated with IV administration of the vitamin. Once severe thiamine deficiency has been corrected, oral supplementation is acceptable.
Riboflavin
Riboflavin (Vitamin B2) is converted in the body to one of two physiologically active coenzymes: flavin mononucleotide (FMN) or flavin adenine dinucleotide (FAD). Because of their ability to “accept” two hydrogen atoms, these coenzymes primarily influence hydrogen ion transport in oxidative enzyme systems, including cytochrome C reductase, succinic dehydrogenase, and xanthine oxidase.
Symptoms of Deficiency
Pharyngitis and angular stomatitis are typically the first signs of riboflavin deficiency. Later, glossitis, red denuded lips, seborrheic dermatitis of the face, and dermatitis over the trunk and extremities occur. Riboflavin deficiency is classically associated with angular cheilitis, photophobia, and scrotal dermatitis—the oral-ocular-genital syndrome. Anemia and peripheral neuropathy may be prominent. Corneal vascularization and cataract formation occur in some subjects. Treatment is with oral vitamin supplements that contain riboflavin.
Nicotinic Acid
Nicotinic acid (Niacin, B3) is converted to the physiologically active coenzyme nicotinamide adenine dinucleotide (NAD) and nicotinamide adenine dinucleotide phosphate (NADP); NAD is converted to NADP by phosphorylation. These coenzymes are necessary to catalyze oxidation-reduction reactions essential for tissue respiration.
Symptoms of Deficiency
Nicotinic acid is an essential dietary constituent, the lack of which leads to nausea, skin and mouth lesions, anemia, headaches, and tiredness. Chronic niacin deficiency is manifested by pellagra in which the skin characteristically becomes erythematous and rough in texture, especially in areas exposed to sun, friction, or pressure. The chief symptoms referable to the digestive tract are stomatitis, enteritis, and diarrhea. The tongue becomes very red and swollen. Salivary secretions are excessive, and nausea and vomiting are common. In addition to dementia, motor and sensory disturbances of the peripheral nerves also occur, mimicking changes that accompany a deficiency of thiamine.
The dietary requirement for niacin can be satisfied not only by nicotinic acid but also by nicotinamide and the amino acid tryptophan. The relationship between nicotinic acid requirements and the intake of tryptophan explains the association of pellagra with tryptophan-deficient corn diets. Carcinoid syndrome is associated with diversion of tryptophan from the synthesis of nicotinic acid to the production of serotonin (5-hydroxytryptamine), leading to symptoms of pellagra. Isoniazid inhibits incorporation of nicotinic acid into NAD and may produce pellagra.
Pellagra is uncommon in the United States, reflecting the supplementation of flour with nicotinic acid. Common causes of pellagra include chronic gastrointestinal disease and alcoholism, which are characteristically associated with multiple nutritional deficiencies. When pellagra is severe, IV administration of nicotinic acid is indicated. In less severe cases, oral administration of nicotinic acid is adequate. The response to nicotinic acid is dramatic, with symptoms waning within 24 hours after initiation of therapy.
Toxic effects of nicotinic acid include flushing, pruritus, hepatotoxicity, hyperuricemia, and activation of peptic ulcer disease. Nicotinic acid has also been prescribed to decrease the plasma concentrations of cholesterol and to increase the concentration of high-density lipoprotein (HDL).
Pyridoxine
Pyridoxine (Vitamin B6) is converted to its physiologically active form, pyridoxal phosphate, by the enzyme pyridoxal kinase. Pyridoxal phosphate serves an important role in metabolism as a coenzyme for the conversion of tryptophan to serotonin and methionine to cysteine.
Symptoms of Deficiency
Pyridoxine deficiency is uncommon and, when present, is associated with deficiencies of other vitamins and, if seen, is more likely to be seen in the elderly, patients with alcoholism, and patients who are severly malnourished. Other patients who are at increased risk of manifesting deficiency are those with chronic renal failure on dialysis, those with hepatic failure, patients with rheumatoid arthritis, women with type 1 diabetes, and those patients infected with HIV. Certain drugs such as anticonvulsants and corticosteroids can interfere with pyridoxine metabolism, as can isoniazid, cycloserine, penicillamine, and hydrocortisone. Seizures accompanying deficiency of pyridoxine and peripheral neuritis such as carpal tunnel syndrome are common. The lowered seizure threshold may reflect decreased concentrations of the inhibitory neurotransmitter γ-aminobutyric acid, the synthesis of which requires a pyridoxal phosphate-requiring enzyme.
As described previously, a person with a deficiency of pyridoxine may also have a deficiency of the other B vitamins.
Drug Interactions
Isoniazid and hydralazine act as potent inhibitors of pyridoxal kinase, thus preventing synthesis of the active coenzyme form of the vitamin. Indeed, administration of pyridoxine decreases the incidence of neurologic side effects associated with the administration of these drugs. Pyridoxine enhances the peripheral decarboxylation of levodopa and decreases its effectiveness for the treatment of Parkinson disease. There is a decrease in the plasma concentration of pyridoxal phosphate in patients taking oral contraceptives.
Pantothenic Acid
Pantothenic acid is converted to its physiologically active form, coenzyme A, which serves as a cofactor for enzyme-catalyzed reactions involving transfer of two carbon (acetyl) groups. Such reactions are important in the oxidative metabolism of carbohydrates, gluconeogenesis, and the synthesis and degradation of fatty acids.
Pantothenic acid deficiency in humans is rare, reflecting the ubiquitous presence of this vitamin in ordinary foods as well as its production by intestinal bacteria. No clearly defined uses of pantothenic acid exist, although it is commonly included in multivitamin preparations and in hyperalimentation solutions.
Biotin
Biotin is an organic acid that functions as a coenzyme for enzyme-catalyzed carboxylation reactions and fatty acid synthesis. In adults, a deficiency of biotin manifests as glossitis, anorexia, dermatitis, and mental depression. Seborrheic dermatitis of infancy is most likely a form of biotin deficiency. For this reason, it is recommended that formulas contain supplemental biotin.
Cyanocobalamin
Cyanocobalamin (Cobalamin, Vitamin B12) and vitamin B12 are generic designations that are used interchangeably to describe several cobalt-containing compounds (cobalamins). Dietary vitamin B12 in the presence of hydrogen ions in the stomach is released from proteins and subsequently binds to a glycoprotein intrinsic factor. This vitamin-intrinsic factor complex travels to the ileum, where it interacts with a specific receptor and is then transported across the intestinal endothelium. After absorption, vitamin B12 binds to a β-globulin, transcobalamin II, for transport to tissues, especially the liver, which serves as its storage depot.
Causes of Deficiency
Although humans depend on exogenous sources of vitamin B12, a deficient diet is rarely the cause of a deficiency state. Instead, gastric achlorhydria and decreased gastric secretion of intrinsic factor are more likely causes of vitamin B12 deficiency in adults. Antibodies to intrinsic factor may interfere with attachment of the complex to gastrin receptors in the ileum.32 Bacterial overgrowth may also prevent an adequate amount of vitamin B12 from reaching the ileum. Surgical resection or disease of the ileum predictably interferes with the absorption of vitamin B12. Nitrous oxide irreversibly oxidizes the cobalt atom of vitamin B12 such that the activity of two vitamin B12–dependent enzymes, methionine synthetase and thymidylate synthetase, are decreased.
Diagnosis of Deficiency
The plasma concentration of vitamin B12 (cobalamin) is less than 200 pg/mL when there is a deficiency state. Measurements of gastric acidity may provide indirect evidence of a defect in gastric parietal cell function, whereas the Schilling test (radioactivity in the urine measured after oral administration of labeled vitamin B12) can be used to quantitate ileal absorption of vitamin B12. Observation of reticulocytosis after a therapeutic trial of vitamin B12 confirms the diagnosis.
Symptoms of Deficiency
Deficiency of vitamin B12 results in defective synthesis of DNA, especially in tissues with the greatest rate of cell turnover. In this regard, symptoms of vitamin B12 deficiency manifest most often in the hematopoietic and nervous systems. Changes in the hematopoietic system are most apparent in erythrocytes, but when vitamin B12 deficiency is severe, a pronounced cytopenia may occur. Clinically, the earliest sign of vitamin B12 deficiency is megaloblastic (pernicious) anemia. Anemia may be so severe that cardiac failure occurs, especially in elderly patients with limited cardiac reserves.
Encephalopathy is a well-recognized complication of vitamin B12 deficiency, manifesting as myelopathy, optic neuropathy, and peripheral neuropathy, either alone or in any combination. Neurologic complications do not parallel the presence of megaloblastic anemia. Damage to the myelin sheath is the most obvious symptom of nervous system dysfunction associated with vitamin B12 deficiency. Demyelination and cell death occur in the spinal cord and cerebral cortex, manifesting as paresthesias of the hands and feet and diminution of sensation of vibration and proprioception with resultant unsteadiness of gait. Deep tendon reflexes are decreased, and, in advanced states, loss of memory and mental confusion occur. Indeed, vitamin B12 deficiency should be considered in elderly patients with psychosis. Folic acid therapy corrects the hematopoietic, but not nervous system, effects produced by vitamin B12 deficiency.
Treatment of Deficiency
Vitamin B12 is available in a pure form for oral or parenteral use or in combination with other vitamins for oral administration. These preparations are of little value in the treatment of patients with deficiency of intrinsic factor or ileal disease. In the presence of clinically apparent vitamin B12 deficiency, oral absorption is not reliable; the preparation of choice is cyanocobalamin administered intramuscularly. For example, in the patient with neurologic changes, leukopenia, or thrombocytopenia, treatment must be aggressive. Initial treatment is with intramuscular administration of vitamin B12 and oral administration of folic acid. An increase in the hematocrit does not occur for 10 to 20 days. The plasma concentration of iron, however, usually declines within 48 hours, because iron is now used in the formation of hemoglobin. Platelet counts can be expected to reach normal levels within days of initiating treatment; the granulocyte count requires a longer period to normalize. Memory and sense of well-being may improve within 24 hours after initiation of therapy. Neurologic signs and symptoms that have been present for prolonged periods, however, often regress slowly and may never return to completely normal function. Indeed, neurologic damage after pernicious anemia develops that is not reversed after 12 to 18 months of therapy is likely to be permanent. Once initiated, vitamin B12 therapy must be continued indefinitely at monthly intervals. It is important to monitor plasma concentrations of vitamin B12 and examine the peripheral blood cells every 3 to 6 months to confirm the adequacy of treatment.
Hydroxocobalamin has hematopoietic activity similar to that of vitamin B12 but appears to offer no advantage despite its somewhat longer duration of action. Furthermore, some patients develop antibodies to the complex of hydroxocobalamin and transcobalamin II. Large doses of hydroxocobalamin have been approved for treatment of cyanide poisoning due to nitroprusside. Conceptually, cyanide reacts with the cobalt in cyanocobalamin, decreasing cyanide ion concentration.
Folic Acid
Folic acid is transported and stored as 5-methylhydrofolate after absorption from the small intestine, principally the jejunum. Conversion to the metabolically active form, tetrahydrofolate, is dependent on the activity of vitamin B12. Tetrahydrofolate acts as an acceptor of 1-carbon units necessary for (a) conversion of homocysteine to methionine, (b) conversion of serine to glycine, (c) synthesis of DNA, and (d) synthesis of purines. Supplies of folic acid are maintained by ingestion of food and by enterohepatic circulation of the vitamin. Virtually all foods contain folic acid, but protracted cooking can destroy up to 90% of the vitamin.
Causes of Deficiency
Folic acid deficiency is a common complication of diseases of the small intestine, such as sprue, that interfere with absorption of the vitamin and its enterohepatic recirculation. Patients with alcoholism have reduced intake of folic acid because of their decreased intake of food, and enterohepatic recirculation may be impaired by the toxic effect of alcohol on hepatocytes. Indeed, alcoholism is the most common cause of folic acid deficiency, with decreases in the plasma concentrations of folic acid manifesting within 24 to 48 hours of continuous alcohol ingestion. Drugs that inhibit dihydrofolate reductase (methotrexate, trimethoprim) or interfere with absorption and storage of folic acid in tissues (phenytoin) may cause folic acid deficiency.
Symptoms of Deficiency
Megaloblastic anemia is the most common manifestation of folic acid deficiency. This anemia cannot be distinguished from that caused by a deficiency of vitamin B12. Folic acid deficiency, however, is confirmed by the presence of a folic acid concentration in the plasma of less than 4 ng/mL. Furthermore, the rapid onset of megaloblastic anemia produced by folic acid deficiency (1 to 4 weeks) reflects the limited in vivo stores of this vitamin and contrasts with the slower onset (2 to 3 years) of symptoms and signs of vitamin B12 deficiency.
Treatment of Deficiency
Folic acid is available as an oral preparation alone or in combination with other vitamins and either an oral preparation or as a parenteral injection. The therapeutic uses of folic acid are limited to the prevention and treatment of deficiencies. For example, pregnancy increases folic acid requirements, and oral supplementation, usually in a multivitamin preparation, is indicated. In the presence of megaloblastic anemia because of folic acid deficiency, the administration of the vitamin is associated with a decrease in the plasma concentration of iron within 48 hours, reflecting new erythropoiesis. Likewise, the reticulocyte count begins to increase within 48 to 72 hours, and the hematocrit begins to increase during the second week of therapy.
Folate Therapy
Vitamin therapy to lower homocysteine levels has been recommended for the prevention of restenosis after coronary angioplasty. This is based on the belief that homocysteine is thrombogenic and is a risk factor for coronary artery disease. Folate supplementation is an effective treatment of homocystinemia. Nevertheless, folate therapy (combination of folic acid, vitamin B6, and vitamin B12) may actually increase the risk of in-stent restenosis and the need for revascularization.33
Leucovorin
Leucovorin (citrovorum factor) is a metabolically active, reduced form of folic acid. After treatment with folic acid antagonists, such as methotrexate, patients may receive leucovorin (rescue therapy), which serves as a source of tetrahydrofolate that cannot be formed due to drug-induced inhibition of dihydrofolate reductase.
Ascorbic Acid
Ascorbic acid (Vitamin C) is a six-carbon compound structurally related to glucose. This vitamin acts as a coenzyme and is important in a number of biochemical reactions, mostly involving oxidation. For example, ascorbic acid is necessary for the synthesis of collagen, carnitine, and corticosteroids. Ascorbic acid is readily absorbed from the gastrointestinal tract, and many foods, such as orange juice and lemon juice, have a high content of ascorbic acid. When gastrointestinal absorption is impaired, ascorbic acid can be administered intramuscularly or intravenously. Apart from its role in nutrition, ascorbic acid is commonly used as an antioxidant to protect the natural flavor and color of many foods.
Despite contrary claims, controlled studies do not support the efficacy of even large doses of ascorbic acid in treating viral respiratory tract infections.34 A risk of large doses of ascorbic acid is the formation of kidney stones resulting from the excessive secretion of oxalate. Excessive ascorbic acid doses can also enhance the absorption of iron and interfere with anticoagulant therapy.
Symptoms of Deficiency
A deficiency of ascorbic acid is known as scurvy. Humans, in contrast to many other mammals, are unable to synthesize ascorbic acid, emphasizing the need for dietary sources of the vitamin to prevent scurvy. Specifically, humans lack the hepatic enzyme necessary to produce ascorbic acid from gluconate. Manifestations of scurvy include gingivitis, rupture of the capillaries with formation of numerous petechiae, and failure of wounds to heal. An associated anemia may reflect a specific function of ascorbic acid on hemoglobin synthesis. Scurvy is evident when the plasma concentration of ascorbic acid is less than 0.15 mg/dL.
Scurvy is encountered among the elderly, alcoholics, and drug addicts. Ascorbic acid requirements are increased during pregnancy, lactation, and stresses such as infection or after surgery. Infants receiving formula diets with inadequate concentrations of ascorbic acid can develop scurvy. Patients receiving TPN should receive supplemental ascorbic acid. Urinary loss of infused ascorbic acid is large, necessitating daily doses of 200 mg to maintain normal concentrations in plasma of 1 mg/dL. Increased urinary excretion of ascorbic acid is caused by salicylates, tetracyclines, and barbiturates.
Fat-Soluble Vitamins
The fat-soluble vitamins are vitamins A, D, E, and K (Fig. 36-2). They are absorbed from the gastrointestinal tract by a complex process that parallels absorption of fat. Thus, any condition that causes malabsorption of fat, such as obstructive jaundice, may result in deficiency of one or all these vitamins. Fat-soluble vitamins are stored principally in the liver and excreted in the feces. Because these vitamins are metabolized very slowly, overdose may produce toxic effects.


Full access? Get Clinical Tree
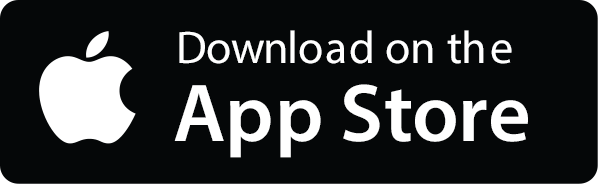
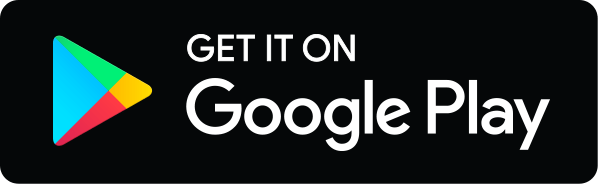