Nonsteroidal anti-inflammatory drugs (NSAIDs) are a diverse group of chemically unrelated compounds that are classified together based on their therapeutic property of possessing anti-inflammatory action ( Fig. 40.1 ). NSAIDs are the most widely prescribed drugs for the treatment of acute and chronic pain, and they account for about 6 to 7 billion dollars in sales worldwide.

Extracts from the bark and leaves of willow, myrtle, and other plants have therapeutic effects because of the presence of salicylic acid and were first used for the treatment of fever in 1763 by Edward Stone. In 1829, Henri Leroux in France obtained a compound of salicylic acid (known as salicin) in crystalline form and succeeded in splitting it to obtain the acid in its pure state. Professor Hermann Kolbe in Germany discovered the compound’s chemical structure and succeeded in synthesizing it in 1859. Sodium salicylate was first used for the treatment of rheumatic fever in 1875. Bayer Pharmaceutical started manufacturing aspirin (acetylsalicylic acid) by 1914 and currently sells about 11 billion tablets annually. Shortly after aspirin was manufactured, other drugs with diverse chemical structures but similar antipyretic, anti-inflammatory, and analgesic properties were discovered; these drugs were grouped together as NSAIDs. John R. Vane elucidated the mechanism of NSAIDs in 1971 and shared the Nobel Prize in Physiology and Medicine with Sune Bergström and Bengt Samuelsson in 1982.
Mechanism of Action
Prostaglandin Synthesis and Pharmacology
Von Euler coined the term prostaglandin (PG) to describe an extract from semen that contracted uterine smooth muscle. Although PGs are derived from arachidonic acid and other polyunsaturated fatty acids, the 20-carbon polyunsaturated essential fatty acid (arachidonic acid) is the major source in mammalian tissues. PGs derived from arachidonic acid (prostaglandin E 2 [PGE 2 ], thromboxane, and prostacyclin) contain two double bonds. Analogous compounds synthesized from eicosatrienoic (linoleic) and eicosapentaenoic acids contain one less or more double bond in the side chains (PGE 1 , PGE 3 , respectively). The various groups of PGs, thromboxanes, hydroxy acids, and leukotrienes that retain the 20-carbon unsaturated fatty acid structure are collectively known as eicosanoids. The three fatty acid precursors (eicosapentaenoic acid) are derived directly or indirectly from dietary fat and are esterified into cell membranes. Their release, usually as a result of trauma, is the major stimulus for eicosanoid production because PGs cannot be stored and are released as soon they are synthesized. Disruption of the cell membrane leads to the release of phospholipid, which is converted to arachidonic acid by the action of phospholipase A 2 . Arachidonic acid in turn acts as a substrate for cyclooxygenase (COX). COX-catalyzed peroxidation of arachidonic acid results in a cyclic structure, whereas peroxidation catalyzed by lipoxygenase produces straight-chain hydroxy-peroxy acids, which can then be converted to hydroxy acids ( Fig. 40.2 ).

PGs are also involved in the pyretic response. After injection of pyrogens, there is a rise in PG levels in cerebrospinal fluid (CSF) that is prevented by pretreatment with aspirin. It is of interest that acetaminophen, which is antipyretic but not anti-inflammatory, blocks brain PG synthetase. Prostanoids do not generally activate nociceptors directly but sensitize them to mechanical stimuli and chemical mediators of nociception, such as bradykinin. PGE 2 is the predominant eicosanoid released from the endothelial cells of small blood vessels and a key mediator of both peripheral and central pain sensitization. Because PGE 2 is the prostanoid most associated with inflammatory responses, the formation of PGE 2 at inflammatory sites is often considered an indicator of local COX activity, and suppression of PGE 2 is an indicator of a decreased inflammatory process. Production of PGE 2 is slow in onset and of long duration in response to inflammation. It is currently recognized that COX is encoded by two genes. Although the isomerization of PGH 2 into PGE 2 has been well characterized biochemically and pharmacologically, the enzyme responsible, PGE synthase, has been purified and cloned only rather recently. This enzyme is one of the membrane-associated proteins in the eicosanoid and glutathione metabolism superfamily, which consists of six proteins with divergent functions.
COX-1 and COX-2 Selectivity
The existence of a COX isoform that is positively and negatively regulated by cytokines and glucocorticoids, respectively, was long suspected, but it was only in the early 1990s that an inducible COX isoenzyme (COX-2) was successfully cloned. The identification of two COX isoforms, COX-1 and COX-2, has generated intense effort to characterize the relative contribution of each isoform to prostanoid production in specific situations. The development of isoform-specific antagonists has been extremely useful, first as experimental tools and, more recently, for clinical therapy.
Both COX-1 and COX-2 isoenzymes are composed of three independent folding units: (1) an epidermal growth factor–like domain, (2) a membrane-binding moiety, and (3) an active enzymatic domain that consists of a long hydrophobic channel. Despite their remarkable structural similarity, the two COX isoforms have different gene expression profiles, distinct kinetic properties, and different interactions with phospholipase A 2 and synthases. The genes for COX-1 and COX-2 are located on human chromosomes 9 and 1, respectively. Whereas COX-1 represents a housekeeping gene that lacks a TATA box, the promoter of the immediate-early gene COX-2 contains a TATA box and binding sites for several transcription factors, including the nuclear factor κB (NF-κB), the nuclear factor for interleukin-6 (IL-6) expression (NF IL-6), and the cyclic adenosine monophosphate (cAMP) response element–binding protein.
COX-1 is expressed constitutively and produces prostanoids that fine-tune physiologic processes requiring instantaneous or continuous regulation (e.g., hemostasis). COX-2 expression is usually low but can be induced by numerous factors, including neurotransmitters, growth factors, proinflammatory cytokines, lipopolysaccharide, calcium, phorbol esters, and small-peptide hormones. However, there are exceptions to the original constitutive versus inducible theory of COX expression. COX-1 expression can be induced in some stress conditions, such as nerve injury, whereas many tissues, including the central nervous system (CNS) and kidney, constitutively express COX-2. In the spinal cord there are detectable basal levels of both COX-1 and COX-2, which might enable immediate reactions to release of transmitter that result in prostanoid production.
It has been suggested that there is another COX enzyme formed as a splice variant of COX-1, known as COX-3. Canine COX-3 expressed by transfected insect cells can be inhibited by therapeutic concentrations of analgesic or antipyretic drugs, such as acetaminophen, phenacetin, antipyrine, and dipyrone. However, it has been reported that this COX-1 variant (COX-3) is not expressed in humans, rats, or mice because the corresponding additional intron 1 sequence in the mRNA transcript spans 94 (human) or 98 (rat and mouse) nucleotides, thus shifting the coding sequence out of frame.
Peripheral and Central Induction of Cox-2
The original hypothesis formulated by Vane in his Nobel Prize–winning work on the mechanism of action of NSAIDs was that these compounds inhibit prostanoid production in the periphery, thereby preventing a sensitizing action of PGE 2 on the peripheral terminals of sensory fibers. More recently, peripheral inflammation has also been shown to induce a widespread increase in COX-2 and PGE synthase expression in the CNS. The proinflammatory cytokine IL-Iβ is upregulated at the site of inflammation and plays a major role in inducing COX-2 in local inflammatory cells by activating NF-κB. IL-1β is also responsible for induction of COX-2 in the CNS in response to peripheral inflammation, but this is not the result of neural activity arising from sensory fibers innervating the inflamed tissue or the result of systemic IL-lβ in plasma. Instead, peripheral inflammation produces some other signaling molecule that enters the circulation, crosses the blood-brain barrier, and acts to elevate IL-lβ levels, which leads to COX-2 expression in neurons and non-neuronal cells in many different areas of the spinal cord. An elevation in COX-2 also occurs at many levels in the brain and spinal cord, mainly in the endothelial cells of the brain vasculature.
Thus, there appear to be two forms of input from peripheral inflamed tissue to the CNS. The first is mediated by electrical activity in sensitized nerve fibers innervating the inflamed area, which signals the location of the inflamed tissue, as well as the onset, duration, and nature of any stimuli applied to this tissue. This input is sensitive to peripherally acting COX-2 inhibitors and neural blockade with local anesthetics, such as occurs with epidural anesthesia. The second is a humoral signal originating from the inflamed tissue that acts to produce widespread induction of COX-2 in the CNS. This input is not affected by regional anesthesia and can be blocked only by centrally acting COX-2 inhibitors. This implies that patients undergoing neuraxial anesthesia for surgery also need a centrally acting COX-2 inhibitor for optimal reduction of postoperative pain and the postoperative stress response.
It is therefore clear that the permeability of the blood-brain barrier to currently used NSAIDs and COX-2 inhibitors is important. Inhibitors of COX-2 that penetrate the blood-brain barrier more effectively might represent more efficient painkillers and could also act to reduce many of the more diffuse aspects of inflammatory pain, such as generalized aches and pains, depression, and loss of appetite, which are key aspects in determining the quality-of-life response to treatment. The main process whereby a drug passes from the bloodstream to the CNS is passive diffusion, for which lipophilicity and ionization are critical determinants of transfer. CSF represents a convenient sampling point for drugs that enter the CNS; however, there are very few NSAIDs for which CSF pharmacokinetics has been defined. For example, the CSF-to-plasma concentration ratio of oxyphenbutazone has been determined to be 0.0074 (0.7%). This ratio is close to the free fraction of oxyphenbutazone in plasma, which is about 0.5%. The high lipid solubility of indomethacin allows it to cross into CSF rapidly and equilibrate with the free plasma concentration. Similar results have been noted with ketoprofen.
Prostanoid Receptors: Expression, Regulation, and Function
The first prostanoid receptor to be isolated and cloned was the thromboxane A 2 (TXA 2 ) receptor, a guanine (G) protein–coupled receptor with seven transmembrane domains. Homology screening of complementary DNA (cDNA) libraries has resulted in the isolation and identification of seven other prostanoid receptors that had been predicted pharmacologically: the PGD receptor (DP), four PGE receptors (EP 1 , EP 2 , EP 3 , and EP 4 ), the PGF receptor (FP), and the PGI receptor (IP). Prostanoid receptors are expressed in many tissues and cell types. Among the EP receptors, EP 3 and EP 4 are the most widely distributed, whereas the distribution of EP 1 and EP 2 is restricted to the kidney, stomach, and uterus, as well as to neuronal and non-neuronal cells in the nervous system. EP 1 , EP 3 , and EP 4 mRNA is expressed in primary sensory neurons in the dorsal root and trigeminal ganglia, thus suggesting involvement in PGE 2 -mediated peripheral sensitization. Inflammation affects the level of expression of many prostanoid receptor subtypes. Further studies are needed to determine which of the EP receptors are involved in analgesia and their respective antagonists.
Inhibition of PG biosynthesis may only partly explain the therapeutic effects of NSAIDs. Another postulated mechanism includes interactions with the adenylate cyclase system. Indomethacin and some other NSAIDs inhibit phosphodiesterase, thereby elevating the intracellular concentration of cAMP.
Pharmacokinetics
NSAIDs are weak acids with p K a values typically lower than 5. Because weak acids will be 99% ionized 2 pH units above their p K a , these anti-inflammatory agents will be present in the body mostly in the ionized form.
General Factors Affecting Nsaid Pharmacokinetics
Although NSAIDs differ in their individual pharmacokinetic properties, some general factors affecting NSAID pharmacokinetics can enable clinicians to select among the different agents available.
Absorption
Most NSAIDs are absorbed rapidly following oral administration, and peak plasma concentrations are generally reached within 2 to 3 hours after administration. Slow-release dosage forms have been developed to maintain active plasma levels for prolonged periods. Factors affecting gastric emptying may have a profound effect on the time course of the clinical effect of the NSAID administered. The extent of drug absorption from the gastrointestinal (GI) tract is more important than the rate. The rectal route of administration has been used to minimize GI side effects, which are a common feature of these agents. In general, the rate and extent of NSAID absorption are comparable for the rectal and oral routes.
Distribution
All NSAIDs are lipid soluble, weakly acidic, and highly bound to plasma proteins, with the exception of aspirin. In most cases, less than 1% of the total plasma concentration is in the unbound form. Albumin is the major binding protein for NSAIDs. Disease conditions that cause hypoalbuminemia will result in an increased free fraction of NSAIDs in plasma, thus affecting the distribution and elimination of these agents. Most NSAIDs have distribution volumes between 0.1 and 0.15 L/kg.
In inflammatory joint diseases, the effect of an NSAID is related to its level in the affected synovial fluid. This level correlates closely with the concentration of drug at the active site because there is simple transport of drugs across the synovial membrane. The amount of drug in synovial fluid is dependent on the level of albumin in the joint, which is lower than in plasma.
Elimination
Hepatic biotransformation is the main elimination pathway for most NSAIDs. Most are metabolized by cytochrome P-450–mediated oxidation, glucuronide conjugation, or both. Renal excretion of nonmetabolized drug is a minor elimination pathway for most NSAIDs and accounts for less than 10% of the administered dose. Some NSAID metabolites are also excreted to a significant extent in bile.
Pathophysiologic Conditions Affecting Nsaid Kinetics
Renal failure and hepatic disease are common disorders that affect the kinetics of NSAIDs.
Renal Failure
Renal failure has a variety of influences on drug kinetics, not only by reducing the renal excretion of drugs and metabolites normally excreted in urine but also by affecting the absorption, distribution, and biotransformation of drugs.
Absorption and Distribution
Absorption of NSAIDs is not impaired in patients with renal failure. However, the plasma protein binding of many acidic compounds such as the NSAIDs is impaired in those with renal failure. The result is an increase in the volume of distribution of the unbound fraction of the drug in plasma.
Elimination
An increase in the unbound fraction of the drug in plasma may lead to an increase in total plasma clearance of the NSAID. The clearance of NSAIDs (e.g., diflunisal, ketoprofen, naproxen, indoprofen, benoxaprofen, tiaprofenic acid) for which the formation of acyl glucuronides is a major elimination pathway is significantly reduced in patients with renal failure. The effect of renal failure on the oral clearance of several other NSAIDs (e.g., ibuprofen, fenbufen, isoxicam, piroxicam) is small. Most of these compounds are metabolized by oxidative pathways. All NSAIDs are highly bound to plasma proteins, and hemodialysis will probably not result in increased elimination of these agents. No dosage adjustments are therefore necessary for patients being administered NSAIDs who are undergoing hemodialysis.
Hepatic Disease
Impaired liver function can affect the disposition of NSAIDs.
Absorption and Distribution
Most NSAIDs are low-clearance drugs, and liver disease should theoretically not be expected to interfere with the oral bioavailability of these agents. Because the liver is the major organ for the synthesis of albumin, the major binding protein for NSAIDs in plasma, it would be expected that hepatic dysfunction could lead to alterations in the unbound drug fraction present in plasma.
Elimination
Because most NSAIDs have low total intrinsic clearance (oral clearance) relative to blood flow, hepatic clearance is essentially independent of flow and reflects drug-metabolizing capacity. Elimination of ibuprofen does not seem to be affected in patients with liver disease.
Pharmacokinetics
NSAIDs are weak acids with p K a values typically lower than 5. Because weak acids will be 99% ionized 2 pH units above their p K a , these anti-inflammatory agents will be present in the body mostly in the ionized form.
General Factors Affecting Nsaid Pharmacokinetics
Although NSAIDs differ in their individual pharmacokinetic properties, some general factors affecting NSAID pharmacokinetics can enable clinicians to select among the different agents available.
Absorption
Most NSAIDs are absorbed rapidly following oral administration, and peak plasma concentrations are generally reached within 2 to 3 hours after administration. Slow-release dosage forms have been developed to maintain active plasma levels for prolonged periods. Factors affecting gastric emptying may have a profound effect on the time course of the clinical effect of the NSAID administered. The extent of drug absorption from the gastrointestinal (GI) tract is more important than the rate. The rectal route of administration has been used to minimize GI side effects, which are a common feature of these agents. In general, the rate and extent of NSAID absorption are comparable for the rectal and oral routes.
Distribution
All NSAIDs are lipid soluble, weakly acidic, and highly bound to plasma proteins, with the exception of aspirin. In most cases, less than 1% of the total plasma concentration is in the unbound form. Albumin is the major binding protein for NSAIDs. Disease conditions that cause hypoalbuminemia will result in an increased free fraction of NSAIDs in plasma, thus affecting the distribution and elimination of these agents. Most NSAIDs have distribution volumes between 0.1 and 0.15 L/kg.
In inflammatory joint diseases, the effect of an NSAID is related to its level in the affected synovial fluid. This level correlates closely with the concentration of drug at the active site because there is simple transport of drugs across the synovial membrane. The amount of drug in synovial fluid is dependent on the level of albumin in the joint, which is lower than in plasma.
Elimination
Hepatic biotransformation is the main elimination pathway for most NSAIDs. Most are metabolized by cytochrome P-450–mediated oxidation, glucuronide conjugation, or both. Renal excretion of nonmetabolized drug is a minor elimination pathway for most NSAIDs and accounts for less than 10% of the administered dose. Some NSAID metabolites are also excreted to a significant extent in bile.
Pathophysiologic Conditions Affecting Nsaid Kinetics
Renal failure and hepatic disease are common disorders that affect the kinetics of NSAIDs.
Renal Failure
Renal failure has a variety of influences on drug kinetics, not only by reducing the renal excretion of drugs and metabolites normally excreted in urine but also by affecting the absorption, distribution, and biotransformation of drugs.
Absorption and Distribution
Absorption of NSAIDs is not impaired in patients with renal failure. However, the plasma protein binding of many acidic compounds such as the NSAIDs is impaired in those with renal failure. The result is an increase in the volume of distribution of the unbound fraction of the drug in plasma.
Elimination
An increase in the unbound fraction of the drug in plasma may lead to an increase in total plasma clearance of the NSAID. The clearance of NSAIDs (e.g., diflunisal, ketoprofen, naproxen, indoprofen, benoxaprofen, tiaprofenic acid) for which the formation of acyl glucuronides is a major elimination pathway is significantly reduced in patients with renal failure. The effect of renal failure on the oral clearance of several other NSAIDs (e.g., ibuprofen, fenbufen, isoxicam, piroxicam) is small. Most of these compounds are metabolized by oxidative pathways. All NSAIDs are highly bound to plasma proteins, and hemodialysis will probably not result in increased elimination of these agents. No dosage adjustments are therefore necessary for patients being administered NSAIDs who are undergoing hemodialysis.
Hepatic Disease
Impaired liver function can affect the disposition of NSAIDs.
Absorption and Distribution
Most NSAIDs are low-clearance drugs, and liver disease should theoretically not be expected to interfere with the oral bioavailability of these agents. Because the liver is the major organ for the synthesis of albumin, the major binding protein for NSAIDs in plasma, it would be expected that hepatic dysfunction could lead to alterations in the unbound drug fraction present in plasma.
Elimination
Because most NSAIDs have low total intrinsic clearance (oral clearance) relative to blood flow, hepatic clearance is essentially independent of flow and reflects drug-metabolizing capacity. Elimination of ibuprofen does not seem to be affected in patients with liver disease.
Specific Drugs
Generic and trade names of NSAIDs are listed in Table 40.1 .
Generic Name | Trade Names |
---|---|
Aspirin | |
Diflunisal | Dolobid |
Diclofenac | Voltaren, Cataflam, Arthrotec (combination with misoprostol |
Indomethacin | Indocin |
Sulindac | Clinoril |
Tolmetin | Tolectin |
Ketorolac | Toradol |
Etodolac | Lodine, Lodine XL |
Ibuprofen | Motrin, Advil, Vicoprofen (combination with hydrocodone), Combunox (combination with oxycodone) |
Flurbiprofen | Ansaid |
Naproxen | Naproxen, Aleve |
Ketoprofen | Orudis |
Fenoprofen | Nalfon, Nalfon 200 |
Oxaprozin | Daypro |
Mefenamic | Ponstel |
Meclofenamic | Meclomen |
Phenylbutazone | Butazolidin |
Piroxicam | Feldene |
Nabumetone | Relafen |
Acetaminophen | Tylenol |
Celecoxib | Celebrex |
Rofecoxib | |
Valdecoxib | |
Meloxicam | Mobic |
Salicylates
The major salicylates currently used are aspirin, diflunisal, choline magnesium trisalicylate, and salsalate.
Aspirin
Aspirin is the most studied and commonly used NSAID. Aspirin has an elimination half-life that increases from 2.5 hours at low doses to 19 hours at high doses. It is well absorbed in the stomach and small intestine, with a peak blood level attained 1 hour after an oral dose. Rapid conversion of aspirin to salicylates then takes place as a result of a high first-pass effect, which occurs in the wall of the small intestine and in the liver. The metabolic pathways follow first-order and zero-order kinetics.
Aspirin inhibits the biosynthesis of PGs by irreversible acetylation and consequent inactivation of COX. This is in contrast to the action of newer NSAIDs, which are reversible inhibitors of the COX enzyme. Although most cells can synthesize new COX, platelets cannot; thus, the acetylation of their microsomal enzyme lasts for the life of the platelet (10 to 14 days). The ability of aspirin to acetylate proteins may be one reason for its superiority over other salicylate derivatives as an anti-inflammatory agent. Of all the NSAIDs, only aspirin has been associated with Reye’s syndrome, a combination of seizures, coma, and sometimes death related to the use of aspirin during a viral illness in children.
Diflunisal
Diflunisal is potentially better tolerated in the GI system because it is not metabolized to salicylic acid in plasma, based on a study comparing diflunisal, 250 and 500 mg twice daily, with aspirin, 600 mg four times daily. It has a short half-life relative to aspirin and causes less inhibition of platelets than aspirin does.
Choline Magnesium Trisalicylate and Salsalate
Both are nonacetylated salicylates that have minimal effect on platelet function and less effect on the GI mucosa than their acetylated counterparts do. They produce similar analgesia and blood levels of salicylate as those of the acetylated class.
Acetaminophen
Acetaminophen is a p -aminophenol derivative with analgesic and antipyretic properties comparable to those of aspirin. Antipyresis probably occurs because of direct action on the hypothalamic heat-regulating centers via the inhibiting action of endogenous pyrogen. Recently, it has been hypothesized that acetaminophen may be acting via the serotonergic pathway to provide analgesia. Though equipotent to aspirin in inhibiting central PG synthesis, acetaminophen exhibits no significant peripheral PG synthetase inhibition. Doses of 650 mg have been shown to be more effective than doses of 300 mg, but little additional benefit is seen at doses higher than 1000 mg, thus indicating a possible ceiling effect.
Acetaminophen has few side effects in the usual dosage range; no significant GI toxicity or functional changes in platelets occur. It is almost entirely metabolized in the liver, and the minor metabolites are responsible for the hepatotoxicity seen with overdose. Inducers of the cytochrome P-450 enzyme system in the liver (e.g., alcohol) increase the formation of metabolites and therefore increase hepatotoxicity. In certain patients (chronic ethanol users, patients suffering from malnutrition, and fasting patients), repeating therapeutic or slightly excessive doses may precipitate hepatotoxicity. A dosage of 2600 to 3200 mg/day may represent a safer chronic daily dosage, and dosing should not exceed 4 g/day. Toxic doses may be a function of baseline glutathione levels and other dose-related factors. Acetaminophen is completely and rapidly absorbed following the oral route. Peak serum concentrations are achieved within 2 hours of a therapeutic dose; therapeutic serum concentrations are 10 to 20 µg/mL. About 90% of acetaminophen is hepatically metabolized to sulfate and glucuronide conjugates for renal excretion, with a small amount being secreted unchanged in urine.
The bioavailability of rectally administered acetaminophen is variable. It is approximately 80% that of tablets, and the rate of absorption is slower, with the maximum plasma concentration being achieved about 2 to 3 hours after administration. Doses of 40 to 60 mg/kg of rectal acetaminophen have been shown to have an opioid-sparing effect in postoperative pain models. Propacetamol, an injectable prodrug of paracetamol, is completely hydrolyzed within 6 minutes of administration, and 1 g of propacetamol yields 0.5 g of paracetamol. Injectable paracetamol has been shown to reduce opioid consumption by about 35% to 45% in postoperative pain studies. In a study examining the analgesic serum concentrations of paracetamol, it was demonstrated that a ceiling effect of paracetamol may be present at intravenous doses of 5 mg/kg, which corresponds to a serum concentration of 14 mg/mL. Recently, an intravenous form of acetaminophen has become available. One gram of injectable acetaminophen is equivalent to 2 g of propacetamol and has a lower incidence of pain at the injection site. Intravenous acetaminophen has been demonstrated to be an effective adjunctive analgesic after orthopedic, ear-nose-throat, and dental surgery. Intravenous acetaminophen also provided analgesia superior to that with oral acetaminophen after cardiac surgery.
Acetic Acid Derivatives
This group of NSAIDs contains two subclasses, pyrrole acetic acids (indomethacin, sulindac, tolmetin, ketorolac, etodolac) and phenylacetic acids (diclofenac, bromfenac).
Indomethacin
Indomethacin has good oral and rectal absorption, although the extent of absorption varies widely among patients. There is also large interpatient variability in its elimination half-life as a result of extensive enterohepatic recirculation of the drug. It is highly bound to albumin. Metabolism involves demethylation and deacetylation in the liver, with subsequent excretion of inactive metabolites and unchanged drug in bile and urine. Its clinical application is somewhat limited by a relatively high incidence of side effects (primarily gastritis and renal dysfunction), but it is used in patients with acute gouty arthritis and osteoarthritis.
Sulindac
Sulindac was developed as the result of a search for a drug similar to indomethacin but with lower toxicity. The GI toxicity associated with sulindac may be lower because it is an inactive prodrug that is converted after absorption by liver microsomal enzymes to sulindac disulfide, which appears to be the active metabolite. It is eliminated from plasma, predominantly by excretion in bile, but is subject to enterohepatic circulation. Peak plasma levels of 0.1 to 0.2 mg/100 mL occur 2 to 3 hours after an oral dose of 200 mg. Steady-state plasma levels of the sulfide are reached after 4 to 5 days of therapy with 200 mg twice daily by mouth. It is available in 150- and 200-mg tablets and administered twice daily for the treatment of gouty arthritis, osteoarthritis, and various inflammatory diseases.
Huskisson and Scott reported that only 25% of patients have GI problems with sulindac; constipation is common. Sulindac seems to have less effect on the CNS than indomethacin does, possibly because it has an indene nucleus, rather than the indole nucleus present in indomethacin and serotonin, an important mediator in the CNS.
Tolmetin and Etodolac
Both these drugs have fewer side effects than other NSAIDs do. Tolmetin is available in 100- and 200-mg tablets and has a half-life of 60 minutes. It is excreted in urine partly unchanged, partly conjugated, and as an inactive dicarboxylic acid metabolite. Tolmetin can cause edema because of sodium retention and abnormal liver function, both of which are reversible on discontinuation of this NSAID.
Etodolac is an acidic compound with a p K a of 4.65. It is manufactured as tablets and capsules, with a normal dosage ranging from to 1 to 1.2 g daily. Clinical doses of 200 to 300 mg twice daily for the relief of low back or shoulder pain have been equated to analgesia with naproxen, 500 mg twice daily. From large clinical trials it has been found that the incidence of abdominal pain and dyspepsia is similar to that with several other NSAIDs, and GI ulceration develops in less than 0.3% of patients. Dyspepsia occurs in 10% of patients taking etodolac, with a somewhat lower incidence of abdominal pain.
Ketorolac
Ketorolac is currently the most commonly used parenteral NSAID in the United States. Although indomethacin has been available as an injectable form for years, it was used only in low dosages for the treatment of patent ductus arteriosus. Ketorolac is almost entirely bound to plasma proteins (more than 99%), which results in a small apparent volume of distribution, and it is extensively metabolized by conjugation and excreted via the kidney. Its analgesic effect occurs within 30 minutes, its maximum effect takes place in 1 to 2 hours, and it has a duration of analgesia of 4 to 6 hours. Ketorolac has been demonstrated to be efficacious in treating postoperative pain in several studies, both as a single agent and as an adjunct to opioids. There is evidence that ketorolac may be acting at the CNS in addition to its peripheral mode of action. Dental surgery studies with ketorolac have also indicated that it acts centrally, but measurement of drug levels in CSF has demonstrated poor penetration of the compound from plasma.
Oral ketorolac was approved for use in the United States approximately 3 years after the parenteral form and has an efficacy similar to that of naproxen and ibuprofen. However, the parenteral form can be administered at single doses of 30 mg intravenously or up to 60 mg intramuscularly. A 30-mg dose can be repeated intravenously or intramuscularly every 6 hours. The oral dose is limited to 10 to 20 mg. Ketorolac should not be used for more than 5 days because of GI toxicity. Since there have been reports of deaths caused by GI and operative site bleeding, the drug’s license was suspended in Germany and France. In response to these adverse events, the drug’s manufacturer recommended reducing the dose of ketorolac from 150 to 120 mg/day. The European Committee for Proprietary Medicinal Products has recommended a further maximal daily dose reduction to 60 mg for older patients and 90 mg for younger adults. Recent studies have examined the efficacy of this drug when administered intranasally. The intranasal route of administration may offer the added advantage of better brain penetration via the olfactory route and might provide higher levels of the drug in the CNS and CSF, with minimal GI side effects.
Diclofenac
Diclofenac has a carboxylic acid functional group and is rapidly and completely absorbed after oral administration. Oral preparations include diclofenac potassium, which is rapidly absorbed in the stomach, and enteric-coated diclofenac sodium, which resists dissolution at low pH and is released in the duodenum. A parenteral form has been used in Europe, with one study showing it to be effective in reducing opioid requirements and reducing pain after thoracotomy. Diclofenac sodium has also been tested in clinical trials in a gel form for relief of pain from superficial burns, with good success. A Cochrane collaborative meta-analysis showed that diclofenac was effective in treating postoperative pain, with a dose-response relationship demonstrating increasing efficacy of diclofenac at 25- , 50-, and 100-mg doses.
Diclofenac binds extensively to plasma albumin. Substantial concentrations of the drug are attained in synovial fluid, which may be one of the sites of action of diclofenac. Concentration-effect relationships have been established for total bound, unbound, and synovial fluid diclofenac concentrations. Diclofenac is eliminated following biotransformation to glucuroconjugated and sulfate metabolites, which are excreted in urine, and very little drug is eliminated unchanged.
Diclofenac differs from other NSAIDs in that it has a high first-pass effect and hence lower oral bioavailability. Diclofenac may also be associated with a significantly higher incidence of hepatotoxicity than other NSAIDs. The excretion of conjugates may be related to renal function. Accumulation of conjugates occurs in patients with end-stage renal disease; however, no accumulation is apparent on comparison of younger and older adults. Dosage adjustments in older adults, children, or patients with various disease states (e.g., hepatic disease, rheumatoid arthritis) may not be required. Significant drug-drug interactions have been demonstrated with aspirin, lithium, digoxin, methotrexate, cyclosporine, cholestyramine, and colestipol.
Propionic Acid Derivatives
This class of compounds includes ibuprofen, fenoprofen, ketoprofen, flurbiprofen, and naproxen. A newer drug in this class is oxaprozin, which has received attention because of its once-daily dosage regimen, but it has no distinct advantage over other NSAIDs.
Ibuprofen
Ibuprofen is well absorbed, and peak plasma levels of 15 to 20 µg/mL are achieved about 1 to 2 hours after a single dose. Its half-life is about 3.5 hours. An intravenous form of ibuprofen has been developed, and an 800-mg intravenous dose is well tolerated and results in faster and higher peak drug levels but a similar total bioavailability and elimination profile as oral dosing. The drug is metabolized mostly in the liver, with less than 10% excreted unchanged in urine and bile. Ibuprofen at a dose of 1200 mg/day produces effective analgesia in arthritis patients. At the high end of the recommended dosage, 2400 mg/day, it produces GI side effects, of which nausea and dyspepsia are predominant. Renal side effects of ibuprofen appear to be dose dependent and have not been reported at the recommended over-the-counter drug dosage (0.2 to 0.8 g/day). Even at anti-inflammatory dosages (>1.6 g/day), renal side effects are almost exclusively encountered in patients with low intravascular volume and low cardiac output, particularly in older adults. Ibuprofen antagonizes the irreversible platelet inhibition induced by aspirin. Therefore, treatment of patients at increased cardiovascular risk with ibuprofen may limit the cardioprotective effects of aspirin.
Ketoprofen
Ketoprofen is available in oral form and reaches its peak plasma level in 0.5 to 2 hours. Its half-life is 2.4 hours, with an analgesic duration of 4 to 6 hours. The maximum recommended dosage is 300 mg/day. Pharmacokinetic data indicate that plasma levels of ketoprofen with oral administration (100 mg) are higher than when applied via a patch. Ketoprofen, 100 mg, has been tried as a patch to directly deliver the compound to the site of injury. Because the patch facilitates delivery of ketoprofen over a 24-hour period, the drug remains continually present in the tissue adjacent to the site of application. High tissue but low plasma ketoprofen concentrations mean that although tissue concentrations are high enough to exert a therapeutic effect, plasma concentrations remain low enough to minimize systemic adverse events.
Fenoprofen
The calcium derivative of fenoprofen is more commonly used; it is well absorbed and achieves a peak plasma level of 20 to 30 µg/mL 2 hours after a single oral dose, with a plasma half-life of 2 to 3 hours. The drug is available as 300-mg capsules, and the recommended dosage is 2.4 g/day. Steady-state plasma levels are reached within the first 24 hours of therapy. Fenoprofen is well tolerated in comparison to aspirin and causes minimal occult GI bleeding; nevertheless, dyspepsia remains its most common side effect. Most of the drug is excreted as glucuronide in urine.
Naproxen
Naproxen is well absorbed in the upper GI tract and is highly bound to plasma albumin. Because of its long half-life of 13 hours, it is suitable for twice-daily administration. Equilibrium is reached in about 3 days. Excretion is almost entirely in urine, mainly as an inactive glucuronide metabolite.
Naproxen is available as 250-, 375-, and 500-mg tablets and has been used for the treatment of arthritis and other inflammatory diseases, with efficacy superior to that of aspirin. It causes less GI irritation than aspirin does. Naproxen increases bleeding time by inhibiting platelet aggregation. When given during pregnancy, it can cross the placenta in 20 minutes and cause neonatal jaundice.
Oxaprozin
Oxaprozin is effective in the management of adult rheumatoid arthritis, osteoarthritis, ankylosing spondylitis, soft tissue disorders, and postoperative dental pain. It has high oral bioavailability (95%), with peak plasma concentrations being achieved 3 to 5 hours after dosing. It is metabolized in the liver by oxidative and conjugative pathways and readily eliminated by the renal and fecal routes. The strong analgesic qualities of oxaprozin are particularly useful for painful musculoskeletal conditions because, for example, it inhibits COX-1 and COX-2 isoenzymes, inhibits nuclear translocation of NF-κB and metalloproteinases, and modulates the endogenous cannabinoid system. In a randomized study of patients with refractory shoulder pain, oxaprozin, 1200 mg once daily, was superior to three doses per day of diclofenac (50 mg) in reducing pain and improving quality of life.
Oxaprozin can diffuse easily into inflamed synovial tissue after oral administration. Though discovered more than 20 years ago, it is now under intensive investigation because of its unusual pharmacodynamic properties. Other than being a nonselective COX inhibitor, the drug is capable of inhibiting both anandamide hydrolase in neurons, with consequent potent analgesic activity, and NF-κB activation in inflammatory cells. Moreover, oxaprozin induces apoptosis of activated monocytes in a dose-dependent manner. Because the monocyte, macrophage, and NF-κB pathways are crucial for the synthesis of proinflammatory and histotoxic mediators in inflamed joints, oxaprozin appears to have pharmacodynamic properties exceeding those presently assumed as markers of classic NSAIDs.
Oxicam Derivatives
The only NSAID in this class in clinical use is piroxicam. Unlike other NSAIDs, the peak serum concentration following oral dosing is attained more slowly, with a duration of 5.5 hours. It is notable for its long elimination half-life, 48.5 hours, so up to 1 week may be needed to achieve steady-state blood concentrations, although it does also allow once-daily dosing.
Pyrazolone Derivatives
The only drug in clinical use in this class is phenylbutazone. Although phenylbutazone is a very effective anti-inflammatory and analgesic, its clinical use has been severely limited because of an association with aplastic anemia and agranulocytosis.
Anthranilic Acid Derivatives
These NSAIDs are unique because they block PG synthesis and the tissue response to PGs. Mefenamic acid has been associated with severe pancytopenia and many other side effects. Therefore, it cannot be used for longer than 1 week. Meclofenamate has a high incidence of GI toxicity and is also not a first-line drug. It is well absorbed orally; peak plasma levels are reached after 2 hours, with a half-life of about 4 to 6 hours.
Naphthylalkanones
This newer class of NSAIDs is most noted for its nonacidic chemical structure, similar to naproxen but unlike that of other clinically used NSAIDs. The only clinically available NSAID in this class is nabumetone. Studies have shown that its use results in fewer gastric lesions than occur with aspirin, naproxen, or ibuprofen. Also, dosages of 1 g/day for 7 days in volunteers resulted in no changes in bleeding time. Only 35% of the drug is converted to its active form after oral administration. None of the parent drug can be measured in plasma after oral administration because of the rapid biotransformation that occurs during the first-pass effect, which makes nabumetone a prodrug.
Meloxicam
Meloxicam is a relatively new NSAID approved for the treatment of osteoarthritis in the United States. It has also been evaluated for the treatment of rheumatoid arthritis, ankylosing spondylitis, and acute rheumatic pain. Meloxicam has been shown to be COX-2 preferential, particularly at its lowest therapeutic dose, and to exert an anti-inflammatory action by inhibiting prostanoid synthesis in inflammatory cells. Because it is COX-2 preferential, it would be expected to have less GI toxicity than nonselective NSAIDs do. In clinical trials of meloxicam for osteoarthritis, it was found to be as effective as piroxicam, diclofenac, and naproxen, with fewer GI symptoms and a lower incidence of perforation, obstruction, and bleeding.
Meloxicam has a plasma half-life of approximately 20 hours and is convenient for once-daily administration in 7.5- and 15-mg tablets. Neither moderate renal nor hepatic insufficiency has significantly altered the pharmacokinetics of meloxicam in short-term studies; however, it should not be used in patients with renal failure. Furthermore, dose adjustment is not required in older adults. Drug-drug interaction studies have demonstrated that meloxicam interacts with some medications, including cholestyramine, lithium, and some inhibitors of cytochrome P-450 2C9 and 3A4. Consequently, increased clinical vigilance should be maintained when co-prescribing some medications with meloxicam. No interactions have been observed following the concomitant administration of food, antacid, aspirin, β-acetyldigoxin, methotrexate, warfarin, or furosemide.
Concentration-dependent therapeutic and toxicologic effects have yet to be extensively elucidated for meloxicam. Its pharmacokinetic profile is characterized by prolonged, almost complete absorption, and the drug is more than 99.5% bound to plasma proteins. Meloxicam is metabolized to four biologically inactive main metabolites, which are excreted in urine and feces. Steady-state plasma concentrations are achieved within 3 to 5 days. The pharmacokinetic parameters of meloxicam are linear over the dose range of 7.5 to 30 mg, and bioequivalence has been shown for a number of different formulations.
COX-2 Inhibitors
COX-2–specific inhibitors were developed with the aim of reducing the incidence of serious GI adverse effects associated with the administration of traditional NSAIDs, the assumption being that these side effects were mediated by COX-1. Assessment of COX-1 and COX-2 selectivity in vitro in whole-blood assays ( Table 40.2 ) of the cellular capacity to produce prostanoids has shown that selectivity is a continuous variable of COX inhibitors.
Drug | IC 50 Ratio |
---|---|
Lumiracoxib | 700 |
Etoricoxib | 344 |
Rofecoxib | 272 |
Valdecoxib | 61 |
Celecoxib | 30 |
Meloxicam | 18 |
Naproxen | 0.7 |
Ibuprofen | 1.5 |
Indomethacin | 0.02 |
Aspirin | 0.007 |
The initial COX-2 inhibitors approved by the U.S. Food and Drug Administration (FDA) were celecoxib and rofecoxib. Overall, a meta-analysis of clinical studies evaluating COX-2 inhibitors versus traditional NSAIDs for postoperative pain has shown that the analgesic efficacy of COX-2 inhibitors 0 to 6 hours postoperatively is similar to or better than that of ibuprofen. The initial clinical trials using COX-2 inhibitors for the management of pain evaluated efficacy in the immediate postoperative period and demonstrated a reduction in postoperative opioid consumption. A meta-analysis examined the advantage of multimodal analgesia with acetaminophen, with NSAIDs, and when COX-2 inhibitors were added to patient-controlled analgesia with morphine. The results suggested that all the analgesic agents provide an opioid-sparing effect (25% to 55%). The use of nonselective NSAIDs was associated with a decrease in the incidence of postoperative nausea, vomiting, and sedation. In addition, the use of COX-2 inhibitors or acetaminophen did not decrease the incidence of opioid-related adverse events when compared with placebo. Clinical trials of COX-2 inhibitors used during the preoperative period and into the postoperative period (2 weeks) for patients undergoing both major surgery and minimally invasive surgery have demonstrated improved clinical outcomes. Perioperative administration of COX-2 inhibitors for total joint arthroplasty has been shown to result in a reduction in perioperative pain and improvement in outcomes without the added risk of increased perioperative bleeding.
It was recently demonstrated that preoperative administration of oral COX-2 inhibitors can reduce CSF PGE 2 levels in humans during the perioperative period, which has resulted in improved outcomes following hip replacement surgery. In addition to the effect on CSF PGE 2 , COX-2 inhibitors were able to modulate the level of CSF IL-6. The exact mechanism responsible for the reduction in interleukin level has yet to be determined but is probably related to the PGE 2 pathway.
Celecoxib
Celecoxib was the first COX-2 inhibitor approved by the FDA (December 1998). It has now been approved for the relief of pain from osteoarthritis, rheumatoid arthritis, acute pain, dysmenorrhea, and familial adenomatous polyposis. It has good selectivity for the COX-2 enzyme (see Table 40.2 ). Celecoxib is available in 100-, 200-, and 400-mg capsules, with a maximum recommended dosage of 400 mg/day for chronic pain. The dose recommended for the management of acute pain is 400 mg followed by 200 mg within the first 24 hours. If celecoxib is administered with aluminum- or magnesium-containing antacids, plasma levels of celecoxib are reduced. Peak plasma levels occur 3 hours after oral administration, and the drug crosses into CSF. Celecoxib is 97% protein bound, with an apparent volume of distribution of 400 L. It is metabolized via cytochrome P-450 2C9 and eliminated predominantly by the liver. It is not indicated for pediatric use and is a category C drug for pregnancy. The drug has a half-life of about 11 hours. Adverse events noted in the various clinical trials include headache, edema, dyspepsia, diarrhea, nausea, and sinusitis. It is contraindicated in patients with sulfonamide allergy or known hypersensitivity to aspirin or other NSAIDs. Because celecoxib does not interfere with platelet function, it can be administered perioperatively as a multimodal analgesic without increasing the risk for bleeding. The incidence of NSAID gastropathy may also be lower with celecoxib than with nonselective NSAIDs.
Rofecoxib
Rofecoxib is a selective COX-2 inhibitor indicated for use in patients with osteoarthritis, rheumatoid arthritis, dysmenorrhea, and acute pain. The drug is administered orally, its bioavailability is 93%, and 87% of the absorbed dose is bound to plasma proteins. The metabolism of rofecoxib in the liver yields metabolites that have no COX-1 or COX-2 activity. Though metabolized by the liver, adjustment in dosage for patients with liver disease is not necessary. The metabolites are predominately eliminated from the body in urine. Analgesic efficacy occurs 0.7 to 1.5 hours after oral dosing and continues for more than 24 hours. Steady-state plasma concentrations of rofecoxib are achieved after 4 days, a function of its 17-hour half-life. It is supplied in 12.5-, 25-, and 50-mg tablets, including a suspension (5 mL contains 12.5mg/5mL or 25mg/5mL).
When compared with other NSAIDs, rofecoxib has less effect on the GI mucosa and therefore has less likelihood of GI complications; adverse events reported with rofecoxib include dyspepsia, peripheral edema, and hypertension. Because rofecoxib has no effect on platelet aggregation and has minimal interaction with warfarin, it has been used extensively in the perioperative arena as a preemptive analgesic for various types of surgeries. Larger clinical trials and long-term follow-up studies undertaken to demonstrate the efficacy of this compound in the prevention of cancer have demonstrated an increased incidence of cardiovascular events, which prompted voluntary withdrawal of rofecoxib by the manufacturer in 2004 (see more detailed discussion later).
Valdecoxib and Parecoxib
Valdecoxib is a derivative of isoxazole and binds noncovalently to COX-2 to form a tight and relatively stable enzyme-inhibitor complex. It is a potent inhibitor of PGE 2 production in humans. Valdecoxib and its metabolites are also the active moieties of a parenteral COX-2 inhibitor, parecoxib sodium.
Valdecoxib has good oral bioavailability (83%) and a minimal first-pass effect. It achieves its maximal plasma concentration in 3 hours, with an elimination half-life of about 8 to 11 hours. It is metabolized by the liver via the cytochrome P450 3A4 isoenzyme. About 70% of the dose is excreted in urine as metabolites. Valdecoxib has been approved for use in patients with osteoarthritis (10 mg), rheumatoid arthritis (10 mg), and acute pain (up to 40 mg). Several clinical studies have demonstrated the efficacy of valdecoxib in patients undergoing oral surgery and major orthopedic surgery at doses of 40 mg. Common adverse events reported include GI and renal toxicity. Clinical studies in high-risk cardiac patients in which a significantly increased incidence of major cardiovascular adverse events and increased risk for serious skin reactions (e.g., toxic epidermal necrolysis, Stevens-Johnson syndrome, erythema multiforme) were demonstrated led to withdrawal of this COX-2 inhibitor from the U.S. market in 2005.
New COX-2 inhibitors that are in clinical use in Europe include etoricoxib and lumiracoxib. However, these drugs have not yet been approved by the FDA. Concerns about their cardiovascular risk profile are as yet unresolved.
Etoricoxib
Etoricoxib (MK-663) is a dipyridinyl derivative that contains a phenyl group attached to the central ring. Etoricoxib is highly selective for COX-2 (IC 50 ratio, 344; see Table 40.2 ), with substantial distribution into tissue and 92% bound to plasma. It is distributed rapidly, with the peak concentration being reached within 1 to 2 hours, and has an elimination half-life of approximately 22 hours. Etoricoxib is metabolized via cytochrome P-450–dependent oxidation, which results in prolonged elimination in patients with liver disease. The highest recommended daily dosage for chronic use is 60 to 90 mg; for acute pain, the dose is 120 mg.
Clinical trials of etoricoxib have demonstrated its analgesic efficacy for various types of arthritis, acute dental pain, dysmenorrhea, and chronic pain conditions such as back pain. Adverse effects include GI, renal, and cardiovascular manifestations. There is a 40% reduction in the relative risk for GI side effects with etoricoxib in comparison to other NSAIDs.
Lumiracoxib
Lumiracoxib is structurally distinct from other COX-2 inhibitors; it is a phenylacetic acid derivative with a short mean plasma half-life of 4 hours, but it provides analgesic efficacy for 24 hours with a single dose. It is 99% protein bound, with a volume of distribution of 13 L and a bioavailability of 74% after oral administration. Lumiracoxib has been found to bind and interact with the COX-2 enzyme via a mechanism different from that of other COX-2–selective inhibitors and carboxylate-containing nonselective COX inhibitors. The carboxylate group of lumiracoxib forms hydrogen bonds with the catalytic Tyr385 and with Ser530 on COX-2 rather than with the larger hydrophobic side pocket or with Arg120. Lumiracoxib is highly selective for COX-2 (see Table 40.2 ). It has been shown clinically to provide analgesia for the treatment of knee osteoarthritis, acute pain after dental surgery, and pain after major joint replacement surgery. Like other COX-2 inhibitors, lumiracoxib has been shown to have a reduced incidence of GI side effects. In a large controlled study (TARGET study) including 18,325 patients, lumiracoxib, 400 mg daily, was shown to cause fewer gastric ulcers than other NSAIDs do; no increase in cardiovascular risk was demonstrated in this study.
Combination Drugs
To enhance the efficacy and safety of NSAID analgesia, drugs have been formulated in combination with NSAIDs. Formulations of ibuprofen-containing hydrocodone are available, and diclofenac has also been formulated in combination with misoprostol. Although such combinations are more convenient, there is no evidence that they are any more effective or safer than when administered separately. Caffeine, long sold in combination with acetaminophen and aspirin in over-the-counter analgesic preparations, has also been studied in combination with ibuprofen. The effect of the added analgesia from caffeine is measurable but not substantial. The enhanced NSAID analgesia seen in combination with caffeine probably does not result from alterations in absorption or distribution of the NSAID.
An oxycodone-ibuprofen combination, 5 mg/400 mg, is manufactured as Combunox. It is an oral fixed-dose combination tablet with analgesic, anti-inflammatory, and antipyretic properties. It is approved in the United States for short-term use (up to 7 days) in the management of acute, moderate, and severe pain and is the first and only fixed-dose combination of ibuprofen and oxycodone. A single dose of oxycodone-ibuprofen (5 mg/400 mg) provided better analgesia than did low-dose oxycodone or ibuprofen administered alone in most trials. It is generally well tolerated after single or multiple doses, and short-term use is not expected to produce any of the serious adverse effects typically associated with the long-term use of opioids or NSAIDs.

Full access? Get Clinical Tree
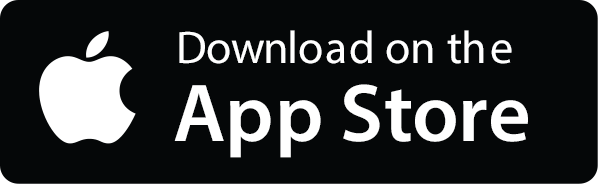
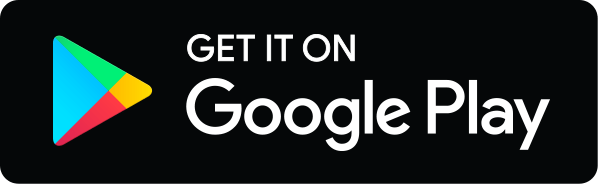