Pulse Oximetry: Clinical Considerations
In addition to SpO2, pulse oximeters provide an indication of tissue perfusion (pulse amplitude) and measure heart rate. Because SpO2 is normally close to 100%, only gross abnormalities are detectable in most anesthetized patients. Depending on a particular patient’s oxygen–hemoglobin dissociation curve, an SpO2 of 90% may indicate a PaO2 of less than 65 mm Hg. This compares with clinically detectable cyanosis, which requires 5 g of desaturated hemoglobin and usually corresponds to an SpO2 of less than 80%. Bronchial intubation usually goes undetected by pulse oximetry in the absence of lung disease or low fraction of inspired oxygen concentrations (FIO2).
Methemoglobinemia: Methemoglobin has the same absorption coefficient at both red and infrared wavelengths. The resulting 1:1 absorption ratio corresponds to a saturation reading of 85%.
Causes of pulse oximetry artifact: Excessive ambient light, motion, methylene blue dye, venous pulsations in a dependent limb, low perfusion, malpositioned sensor and leakage of light from the light-emitting diode to the sensor (bypassing the arterial bed).
Extensions of Pulse Oximetry Technology
Mixed venous blood oxygen saturation: Requires the placement of a pulmonary artery (PA) catheter containing fiberoptic sensors that continuously determine SVO2. A variation involves placing the sensor in the internal jugular vein, which provides measurements of jugular bulb oxygen saturation to assess cerebral oxygen delivery.
Noninvasive brain oximetry: Monitors regional oxygen saturation (rSO2) of hemoglobin in the brain. A forehead sensor emits light of specific wavelengths and measures the light reflected back to the sensor. In contrast to pulse oximetry, brain oximetry measures venous, capillary, and arterial saturation, thereby providing an average oxygen saturation of all regional hemoglobin (∼70%). Cardiac arrest, cerebral embolization, deep hypothermia, or severe hypoxia can cause a dramatic decrease in rSO2.
Capnography
Capnographs rely on the absorption of infrared light by CO2. Determination of end-tidal CO2 (ETCO2) concentration to confirm adequate ventilation is mandatory during all anesthetic procedures.
Capnography: Nondiverting (Flowthrough)
Nondiverting (mainstream) capnographs measure CO2 passing through an adaptor placed in the breathing circuit. Infrared light transmission through the gas is measured and CO2 concentration is determined by the monitor.
Capnography: Diverting (Aspiration)
Diverting capnographs continuously suction gas from the circuit into a sample cell in the monitor. CO2 concentration is determined by comparing infrared absorption in the cell with a chamber devoid of CO2.
Aspiration rates: High aspiration rates (up to 250 mL/min) and low-deadspace sampling tubing usually increase sensitivity and decrease lag time. If tidal volumes (VT) are small (e.g., pediatric patients), however, a high rate of aspiration may entrain fresh gas from the circuit and dilute ETCO2 measurement. Low aspiration rates (less than 50 mL/min) can underestimate ETCO2 during rapid ventilation.
Water precipitation: Diverting units are prone to water precipitation in the aspiration tube and sampling cell that can cause obstruction of the sampling line and erroneous readings.
Expiratory valve malfunction is detected by the presence of CO2 in inspired gas.
Capnography: Clinical Considerations
Capnographs reliably indicate esophageal intubation but do not reliably detect bronchial intubation. Sudden cessation of CO2 during the expiratory phase may indicate a circuit disconnection. A marked rise in ETCO2 may be caused by the increased metabolic rate associated with malignant hyperthermia.
The PaCO2-ETCO2 gradient (usually 2–5 mm Hg) reflects alveolar dead space (alveoli that are ventilated but not perfused). Capnographs display a CO2 waveform that allows recognition of a variety of conditions:
A. Normal capnograph with the three phases of expiration: phase I—dead space; phase II—dead space and alveolar gas; phase III—alveolar gas plateau.
B. Capnograph of severe chronic obstructive pulmonary disease. No plateau is reached before the next inspiration. The ETCO2 and arterial CO2 gradient is increased.
C. Depression during phase III indicates spontaneous respiratory effort.
D. Failure of the inspired CO2 to return to zero may be attributable to an incompetent expiratory valve or exhausted CO2 absorbent.
E. Persistence of exhaled gas during the inspiratory cycle signals the presence of an incompetent inspira-tory valve.
Anesthetic Gas Analysis
Anesthetic gas analysis is essential during any inhalational anesthetic use. There are no contraindications.
Infrared absorption: Relies on a variety of techniques similar those used in capnography. Based on the Beer-Lambert law; the absorption of infrared light passing through a solvent (inspired or expired gas) is proportional to the amount of the unknown gas. O2 and N2 do not absorb infrared light and must be measured by other means.
Piezoelectric analysis: Uses oscillating quartz crystals, one of which is covered in lipid. Volatile anesthetics dissolve in the lipid layer, and their concentration is determined by the change in oscillation frequency. This method cannot distinguish different anesthetic agents.
Oxygen Analysis
Galvanic cell: Galvanic cell hydroxyl ions are formed at the gold cathode and react with the lead anode. An electrical current is produced that is proportional to the amount of oxygen being measured.
Paramagnetic analysis: Oxygen is a nonpolar gas that is paramagnetic and expands when placed in a magnetic field. By switching the field on and off, the volume change can be used to measure O2 content.
Polarographic electrode: A semipermeable membrane separates a gold cathode and silver anode. A voltage is applied, and hydroxide ions are formed from O2; the resultant current is proportional to the amount of O2.
Spirometry
Anesthesia machines can measure and manage airway pressures, volume, and flow; calculate resistance and compliance; and then display the relationships of these variables as flow–volume or pressure–volume loops.
Low and high peak inspiratory pressure: Indicate circuit disconnect or airway obstruction, respectively.
Minute ventilation: Obtained by measuring VT and breathing frequency.
Spirometric loops and waveforms are altered by certain disease processes and events (e.g., obstruction, bronchial intubation).

Full access? Get Clinical Tree
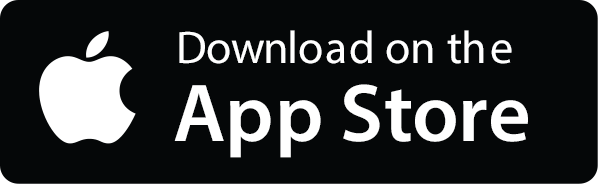
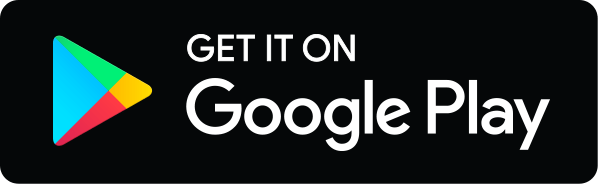