The synapse between the primary afferent and secondary afferent neurons in the dorsal horn of the spinal cord is a critical site for the modulation of ascending pain pathways. Glutamate is the primary excitatory amino acid (EAA) involved in excitation of the secondary afferent neuron and is primarily stored in the presynaptic vesicles of the primary afferent neuron. Upon membrane depolarization, glutamate is released and targets postsynaptic receptors, which can be either metabotropic or ionotropic. Ionotropic glutamate receptors are ligand-gated channels that regulate ion conductance of calcium and sodium. There are three major subclasses of ionotropic receptors: a-amino-3-hydroxy-5-methyl-4-isoxazolepropionic acid (AMPA), kainate, and N-methyl-D-aspartate (NMDA). The discovery of several pharmacologic agents, beginning with compound (+) MK-801, that are highly selective antagonists for specific subtypes of glutamate receptors has helped clarify the characteristics and functions of the various glutamate receptors and in particular the role of the NMDA calcium channel in the central nervous system (CNS).1
Among various locations in the CNS, NMDA receptors are found on the postsynaptic terminal of second-order afferent neurons in the dorsal horn of the spinal cord that are involved in the transmission of pain signals. With the cloning of individual NMDA receptor subunit DNA sequences, it was ultimately discovered that NMDA receptors consist of glycine-binding GluN1 subunit and glutamate-binding GluN2 subunits. NMDA receptors are calcium ion channels composed of four individual subunits, including two GluN1 subunits and two GluN2 subunits, and the receptor requires coactivation by two ligands, glutamate and glycine. There is considerable diversity among receptor subtypes, which imparts distinct functional and pharmacologic properties to the NMDA receptor.1 A unique property of the NMDA receptor is that in addition to being ligand activated, its activation is also voltage dependent, that is, dependent on the resting cell potential. At a normal resting membrane state, the NMDA calcium channel is blocked by magnesium and is in an inactive state. When the resting membrane potential of the afferent neuron is changed as a result of prolonged stimulus, the channel loses the magnesium ion block, and calcium moves into the cell, raising the membrane potential, resulting in neuronal hyperexcitability.2
Sound in vivo evidence indicates that peripheral tissue or nerve injury causes not only an increase in the sensitivity of primary afferent nociceptors at the site of tissue injury but also leads to NMDA receptor–mediated central changes in synaptic excitability and, consequently, on a clinical level, a reduction in opioid responsiveness, hyperalgesia, and allodynia.3,4
The NMDA receptor is a key component in the modulation of the strength of synaptic pathways, a property referred to as synaptic plasticity. This is demonstrated in its involvement in the states of windup and central sensitization. Windup refers to reversible synaptic changes that can result in neuronal hyperexcitability in response to a progressive or sustained noxious stimulus. In response to a sustained C-fiber or nociceptive stimulus, primary afferents express synaptic glutamate and other neurotransmitters, such as substance P, which then traverse the synapse to reach NMDA and other glutamatergic receptors on the secondary afferent neuron. The summation of these repeated synaptic discharges produces a crescendo depolarization of the secondary afferent neuron that leads to the loss of the magnesium ion blockade in voltage-gated NMDA receptors, allowing increased calcium conduction and more sustained depolarization of the secondary afferent neuron. When this process is sustained, it contributes to a cascade of phosphorylation and other intracellular transcription and translational events that potentiate the more established hyperexcitable state of central sensitization. Clinically, this sustained robust synaptic transmission leads to a reduction in pain threshold, an augmentation of pain responses, and an expansion of pain sensitivity to tissue regions that have not been injured.5,6
Clearly, the involvement of the NMDA receptor in the establishment and fortification of robust pain transmission pathways exposes a potential target for pain therapy. Several NMDA antagonist medications have been developed and extensively researched and are now becoming more broadly clinically applied, offering considerable benefit in the prevention of pain in both acute perioperative and chronic neuropathic clinical settings.
The NMDA receptor is found in many different levels of the CNS, including not only the afferent pain pathways in the dorsal horn of the spinal cord but also regions of the brain involved in motor and cognitive processes. As it is prominently involved in controlling synaptic plasticity, NMDA receptors have been determined to have a critical role in cognition and memory function.6,7 NMDA receptor antagonists, therefore, expectedly, have multiple sites of action. Unfortunately, because NMDA receptor antagonists also impair normal synaptic transmission in nonpathologic processes, they can cause numerous side effects such as memory impairment, psychotomimetic effects, ataxia, and motor incoordination.
A major goal in pharmacologic development is the development of agents that have specificity for NMDA receptors that are involved in pathologic processes and do not interfere with normal physiologic roles in non–pain-related centers. NMDA channel blockers act in a noncompetitive manner that is use dependent, that is, they can only access their target subunit binding site and block the channel in its open conformation. These agents differ from each other in their binding affinities for their target subunits sites on the NMDA receptor, which result in different pharmacologic and physiologic characteristics. It has been clarified that NMDA antagonist agents with high affinity for the receptor subunits are more likely to disrupt normal physiologic processes and that noncompetitive agents with lower affinity for the receptor subunit target binding sites offer a better therapeutic benefit to side effect profile. Currently, available agents, such as memantine, ketamine, and dextromethorphan (DM), are clinically used agents that meet this lower affinity profile.2–4
Ketamine is an NMDA receptor antagonist that has, arguably, the most clearly defined clinical utility. It has been available for research purposes for nearly 50 years and has been available in clinical practice for more than 30 years. It has various industrial names such as Ketalar and Ketajet as well as street names for illicit use such as Special K and Vitamin K. Ketamine has multiple target receptors, including NMDA, AMPA, and kainate. Ketamine is involved in the inhibition of voltage-gated sodium and potassium channels and serotonin and dopamine reuptake inhibition and has weak agonist properties at nicotinic receptors and antagonist properties at muscarinic receptors. Ketamine is available as a racemic mixture of the S and R isomers. The S isomer appears to cause greater unwanted psychic side effects than the R isomer.
Ketamine has several cardiovascular effects. It is a direct myocardial depressant; however, this effect is generally overridden by its sympathetic stimulation as well as the release of and reuptake inhibition of catecholamines. Ketamine generally causes an increase in systemic arterial pressure, heart rate, and cardiac output unless a patient is depleted of catecholamines in which case myocardial depression can be appreciated.
Ketamine has effects on the pulmonary system. Most important, it is a bronchial smooth muscle relaxant. The effect of ketamine on bronchial smooth muscle is as effective as inhalational agents in preventing bronchospasm; however, it must be acknowledged that ketamine causes an increase in pulmonary arterial pressure and in the acute care setting causes an increase in salivary and tracheobronchial secretions.
Ketamine has several important effects on the neurologic examination. Most prominently, nystagmus is noted frequently at doses of 0.25 to 0.5 mg/kg. Ketamine does not alter the seizure threshold, but it does increase the cerebral metabolic rate of oxygen (CMRO2) as well as cerebral blood flow, and critically, it can cause an increase in intracranial pressure.
Ketamine has high (93%) bioavailability with intravenous (IV) administration yet because of high first-pass metabolism has a low oral bioavailability of 16%. However, because of a significantly higher accumulation of norketamine with the oral route, the effective oral dose is roughly one-third the effective IV dose. The metabolism of ketamine occurs via N-demethylation to norketamine primarily via the CYP384 system and less so via the CYP2B6 and CYP2C9 cytochrome systems. The metabolite norketamine has been shown to have 20% to 30% of the physiologic activity of ketamine. The metabolite norketamine is eventually hydroxylated to hydroxynorketamine and conjugated with glucuronate and finally is excreted into urine, where 80% is eliminated as the conjugated derivatives of ketamine.
Clinical research has revealed several major therapeutic advantages of ketamine use, including decreased opioid requirements in the postsurgical setting, attenuated opioid tolerance, the suppression of the onset of opioid-induced hyperalgesia.8
In a 2006 review, Bell et al. reviewed the role of perioperative ketamine usage in the management of acute postoperative pain. A total of 37 trials were reviewed, including 2240 participants and including randomized controlled trials (RCTs) of patients treated with perioperative ketamine versus placebo. The authors concluded that subanesthetic doses of ketamine indeed reduced the need for rescue analgesia doses, reduced overall pain intensity scores, reduced postoperative Patient-controlled morphine consumption, and reduced postoperative nausea and vomiting; adverse effects were found to be mild or even absent.9
In 2005, Joly et al. published a study involving 75 patients who underwent major upper abdominal surgery. Treatment groups were randomized to receive intraoperative remifentanil in two different groups of eightfold dosage difference plus a third group receiving a remifentanil plus ketamine infusion of 0.5 mg/kg/min initiated just after incision followed by an infusion for 48 hours of 2 µg/kg/min. Results demonstrated that hyperalgesia in the high-dose remifentanil group was substantially greater than hyperalgesia in the other two groups. Furthermore, it was shown that this hyperalgesia can be prevented by low-dose ketamine infusions.
In 2005, a study by Adam et al. involved 40 patients undergoing total knee arthroplasty under general anesthesia and continuous femoral nerve block. Treatment groups were randomized to involve a ketamine 0.5-mg/kg bolus before skin incision followed by infusion at 3 µg/kg/min until emergence from anesthesia followed by infusion at 1.5 µg/kg/min for 48 hours; a second group received only blinded placebo. Results demonstrated that the ketamine group required less overall morphine, reached 90-degree flexion more rapidly, and was able to achieve these goals without any additional side effects from ketamine therapy.11
A 2004 study by Kwok et al. involved 135 patients in three separate treatment groups investigating the role of ketamine as a preemptive analgesic. Group 1, the preincision group, received ketamine at 0.15 mg/kg immediately before induction of anesthesia; group 2, the postoperative group, received ketamine 0.15 mg/kg only after wound closure; and group 3 received placebo. Compared with groups 2 and 3, results demonstrated that group 1, the preincision and preemptive ketamine group, had lower pain scores, had a longer time to first request for rescue analgesia postoperatively, and required a lower overall total morphine consumption in the perioperative period. This was demonstrated with no difference in hemodynamic variables or other adverse effects during the study.12
In 2010, Goldberg et al. investigated the pharmacodynamic responses to racemic ketamine and norketamine in patients with complex regional pain syndrome (CRPS). Ketamine was titrated from 10 to 40 mg/hr in patients with CRPS and maintained at such levels for 5 days. Serum ketamine and norketamine concentrations were determined at several intervals during and after the conclusion of the 5-day infusion. Ketamine concentrations were found to peak at 4 to 5 hours. Norketamine concentrations were lower and peaked on day 2 of the infusion. Significant pain relief was achieved by day 2 of the infusion and correlated with the maximum plasma levels of ketamine and norketamine. Pain continued to improve over the 5-day infusion and was found to peak when serum ketamine concentrations of 200 to 225 ng/mL and norketamine levels of 9220 ng/mL were reached.13
When considering therapy of chronic pain using ketamine, one must take into consideration what little information is available about the clinical effects of long-term ketamine exposure. To investigate the clinical effects of long-term ketamine exposure, there are two major available cohorts. First, there are patients receiving ketamine for legitimate therapeutic reasons, generally in the perioperative setting. Second, there are abusers of ketamine in the community. Studying this second group provides a unique opportunity to gather data about long-term ketamine exposure on relatively larger cohorts. It must be acknowledged that this particular cohort is very heterogeneous, and there is frequently concomitant polysubstance abuse.8 Ketamine is classified as a schedule 3 drug under the Controlled Substances Act; however, very few deaths have been associated with ketamine overdoses. Ketamine is frequently abused using routes of administration such as swallowing, snorting, smoking, or injecting into the muscles and veins.

Full access? Get Clinical Tree
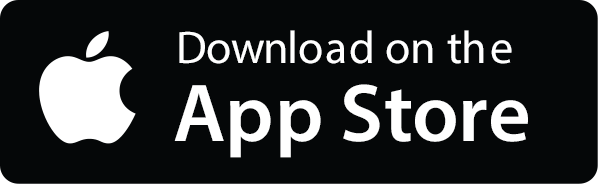
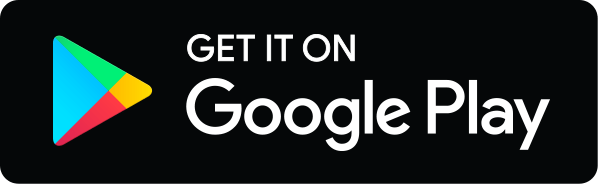