After uncomplicated neurosurgical procedures, patients are often admitted to an intensive care unit (ICU) for close observation. In the large majority of cases, this level of care is warranted as a means to monitor the patient’s neurologic function carefully and to expedite appropriate intervention if necessary. Further, observation and evaluation of postoperative neurosurgical patients may be complicated by the residual effects of the prior anesthetic and an ongoing need for analgesia and sedation.
The Leapfrog Group has shown that the implementation of basic intensive care practices can vastly improve patient safety. One of these practices is choosing a hospital with ICUs that are staffed at least 8 hours daily by specially trained critical care physicians. Mirski and colleagues showed that treatment in a dedicated specialty neurologic-neurosurgical ICU (NSICU) improved mortality and disposition at discharge for patients with intracerebral hemorrhage, compared with a similar cohort treated 2 years earlier in a general ICU setting. Furthermore, critically ill postoperative neurosurgical and neurologic patients who are treated in the NSICU had shorter hospital stays and lower total costs of care than a national benchmark. The data suggest that a neuroscience specialty ICU staffed by specialty-trained intensivists and nurses is beneficial. Only a small fraction of patients require prolonged ICU stay after craniotomy for tumor resection. A patient’s risk of prolonged stay can be predicted by certain radiologic findings, large intraoperative blood loss, fluid requirements, and the decision to keep the patient intubated at the end of surgery. For those patients requiring ICU resources beyond the first 4 hours, the interventions can be critical. It is, therefore, crucial to have appropriate skills and resources readily available.
In this chapter, we describe the methods we have implemented in many NSICUs to improve outcome after neurosurgical procedures ( Fig. 37.1 ). In basic terms, most improvement in postoperative outcome is attained by recognizing acute reversible neurologic injury and distinguishing it from expanding intracompartmental mass lesions or edema, and by preventing secondary neurologic insults from decreased ventilation or cerebral hypoperfusion. We summarize the care given to postoperative patients who have undergone major neurosurgical procedures that carry a higher risk for neurologic impairment.

Neurologic Support
Focused Neurologic Examination
Postoperative neurosurgical patients require rapid initial neurologic assessment. The Glasgow Coma Scale (GCS) score is the most widely recognized assessment of cortical function, consisting of motor, verbal, and eye opening (arousal) responses ( Box 37.1 ) The GCS offers simplicity and reproducibility, but it is affected by other factors causing cortical impairment such as sedatives, narcotics, temperature, glucose levels, and electrolyte imbalances; it also lacks compensatory scores for intubated or aphasic patients. Regardless of a patient’s level of consciousness, an examiner must evaluate cranial nerve and brainstem reflexes, including pupil size and light reflex, eye deviation and extraocular muscle movements, oculovestibular and oculocochlear reflexes, facial symmetry/movement, gag reflex, and midline tongue protrusion (see Box 37.1 ).
Cortical function: Glasgow Coma Score (GCS)
Eye opening
Spontaneous 4
Speech 3
Pain 2
None 1
Motor response
Obeys commands 6
Localizes 5
Withdraws 4
Flexes 3
Extends 2
No response 1
Verbal response
Oriented 5
Confused 4
Inappropriate words 3
Incomprehensible sounds 2
No response 1
Eye opening + motor response + verbal response = GCS
Brainstem function
Reflexes
Pupillary light reflex
Ocular movements
Corneal reflex
Facial grimace
Gag
Cough
Spinal cord function
Deep tendon reflexes
Brachial
Achilles
Motor response
Hands
Toes
Sensory
Light touch
Spinal cord function can be assessed using reflexes, muscle strength, and peripheral sensation. Triceps, biceps, brachioradialis, patellar, and ankle reflexes are graded and compared with the contralateral side. The standardized motor strength grading is based on a scale of 1 to 5. Typically, flexor and extensor muscle groups in the proximal and distal portion of each extremity are tested as an initial screen, with further and more detailed testing of individual muscles as warranted by the history or initial screen. If a patient is unable to comply fully with the motor examination because of an alteration in level of consciousness, an examiner may still observe some measure of muscle strength based on spontaneous movement or withdrawal from stimuli. Passively flexing and extending the extremities can be used to assess the patient’s muscle tone.
A screening examination consists of light touch and pain sensation attained proximally and distally in each extremity, as well as proprioceptive sense in the thumbs and great toes. Stimulating the lateral sole assesses plantar responses. Testing finger-to-nose and heel-to-shin maneuvers is used to assess cerebellar function.
The results of the focused neurologic examination are used as the basis for further monitoring, which requires coordination between examiners as they assess neurologic recovery. With the exception of some simple reflexes (which are either present or not present), the neurologic examination is subjective, and variability can be expected to exist between examiners; however, repeated examinations by the same health-care professional are consistent and this consistency is bolstered by knowledge of the clinical history. In summary, the intensive and intermediate nursing care of postoperative neurosurgical patients is based on the repeated observations of responses to the neurologic examination.
Postoperative Imaging
Central nervous system imaging studies are often an extension of the neurologic examination, because they can be used to assess anatomic lesions, which may then be related to neurologic dysfunction uncovered by the examination. In the postoperative neurosurgical population, imaging is useful for any of three reasons: (1) because the patient is unable to arouse fully in a reasonable amount of time after anesthesia, (2) because a neurologic deficit is seen postoperatively that was not seen preoperatively, or (3) because a preexisting deficit is worse postoperatively than it was preoperatively.
Computed tomography (CT) scanning, which provides fast, detailed images by computerized analysis of circumferential radiographs, is the mainstay for urgent or emergent evaluation of ventricular size, edema, hemorrhage, and bony structures. The main advantage of CT is the speed with which the scans can be obtained because patient compliance may be limited in this population. Spiral CT scans provide three-dimensional (3-D) images (especially useful in examining vascular anatomy and cerebrospinal fluid [CSF] flow), and perfusion CT scanning may give some measure of regional cerebral blood flow. Additionally, with the improved availability of portable CT scanners, reductions in transportation-related morbidity and time to clinical decision may be facilitated without compromise of image quality.
Magnetic resonance imaging (MRI) may be used in addition to or instead of CT to provide the highest anatomic detail available. MRI uses pulse sequences of magnetic radio waves to orient cellular nuclei in a particular direction; computer analysis then converts the differences in radio energy imparted by the subsequent relaxation of these nuclei into excellent images that provide information on gray and white matter structures, edema, CSF, hemorrhage, blood–brain barrier integrity (with the use of gadolinium contrast), and tumors. Newer pulse sequences allow 3-D images of intracranial vasculature and perfusion studies. The main disadvantage of MRI is the length of time it takes to perform a study: complete imaging of the brain may take up to 1 hour. Also, acquiring the images can be difficult in the noncompliant or critically ill patient. Another disadvantage is that the noise and closeness of the MRI machine result in many patients’ needing to be sedated because of claustrophobia and anxiety. For these reasons, MRI is usually reserved for situations in which CT is inadequate (e.g., for imaging in the posterior fossa), for non emergent detailed imaging after intracranial tumor surgery, and for spinal cord imaging.
Finally, conventional angiography remains the gold standard for vascular imaging. In the postoperative period, it is mainly reserved for use after intracranial vascular surgery when MR angiography or CT angiography is contraindicated or of questionable efficacy.
Postoperative Intracranial Pressure Monitoring
Because the central nervous system is relatively incompressible, postoperative intracranial pressure (ICP) may be directly related to mean arterial pressure (MAP) after compensatory mechanisms have been exhausted. Cerebral perfusion pressure (CPP) equals MAP minus the highest downstream pressure, usually the ICP. If cortical function is normal, CPP may be approximated by measuring MAP when ICP is unknown. However, the central nervous system is somewhat compartmentalized because of the separation of the cerebral hemispheres and posterior fossa by the dural reflections, and thus raising or lowering the MAP may not directly raise or lower ICP in a linear manner. Therefore, it is often necessary to monitor the ICP invasively.
ICP should be monitored directly when its manipulation involves prolonged attempts or when a degree of hydrocephalus might result in depressed neurologic function. Historically, this has been done by ventriculostomy or cranial bolt placement. However, newer ICP monitors can evaluate regional oxygenation, temperature, and pressure. These catheters may be used to guide management of intracranial pressure, oxygenation, and cerebral metabolic rate (CMR) but are largely not validated with outcome data.
Management of Postoperative Complications
Immediately after a neurosurgical procedure, complications include cerebral edema, hematoma, and seizures. Global cerebral edema, as seen after head trauma, with associated increases in ICP has been shown to have high mortality, thought to be largely the result of decreased cerebral blood flow (CBF). Although the regional edema seen at the surgical site is not associated with the same risks or mortality, postsurgical edema with neurologic compromise may be caused by local or diffuse decrements in CBF and thus may benefit from ICP management. The goals are to supply the metabolic demands of the brain by maintaining perfusion, oxygenation, and glucose. The treatment for elevated ICP is first directed at the cause, which in this patient population is typically postsurgical edema, but it may include intracerebral hemorrhage, cerebral venous occlusion, focal ischemia or infarction, infection, or unresected tumor.
Management of ICP begins with supporting the viable neuronal tissue until definitive therapy for the underlying cause can be achieved. Maneuvers intended to decrease ICP in the setting of cerebral edema range from hyperventilation to calvarium removal; however, in the immediate postoperative period, most interventions are meant to reduce the volume of CSF or blood in the cranial vault.
Hyperventilation, routinely used to reduce CBF via cerebral vasoconstriction, can be achieved through increases in tidal volume, respiratory rate, or both. Its disadvantages include reducing CBF in concurrently ischemic brain, presumably because of associated vasoconstriction, decreasing seizure threshold, rebound increase in ICP, and CSF acidosis after cessation. Osmotherapy, aimed at shifting intracranial fluid into the vascular compartment, utilizes hyperosmolar solutes such as mannitol or hypertonic saline to reduce ICP. Although mannitol acts to promote osmotic diuresis, it may also diffuse into areas of the brain where the blood–brain barrier is no longer intact and theoretically could cause worsening of regional edema. Likewise, infusion of hypertonic saline with subsequent rapid increases in serum sodium have been associated with irreversible myelinolysis. Intravenous corticosteroids can be used to actively treat the cause of cerebral vasogenic edema, as seen in malignancies. High dosages may be necessary and may be difficult to wean quickly. Finally, barbiturates have been used to decrease ICP through lowering CBF and CMR. In general, high dosages are required to induce burst-suppression patterns on electroencephalogram. Therefore, the blood pressure may need pharmacologic augmentation with inotropes and/or vasoconstrictors.
Postoperative hematoma formation is another major concern. The location of surgery best dictates how to monitor the patient for this. Epidural hematoma formation occurring after spinal surgery is usually indicated by new and progressive neurologic symptoms in the extremities and demands immediate diagnosis with CT and with surgical evacuation and decompression. Although the postoperative development of intracerebral or epidural hemorrhages is not unheard of, subdural hematoma formation is more common after intracranial procedures and may be signified by alteration in mental status, unilateral neurologic signs, or failing to recover fully from anesthesia. Again, immediate imaging with CT and consideration of evacuation is indicated.
Finally, seizures may complicate the early postoperative course. The largest incidence study indicated that early postoperative seizures may occur in as many as 17% of cases, allowing for wide variation between inciting pathologies. Although many studies have found differing results regarding seizure prophylaxis utilizing a myriad of antiepileptic drugs (AEDs) including phenytoin, carbamazepine, valproic acid, phenytoin, phenobarbital, zonisamide, and levetiracetam, a recent systematic review suggests that there is inconsistent, low-quality evidence to suggest that AEDs are effective in the reduction of early or late seizures postcraniotomy. Current recommendations include the use of perioperative antiepileptic drugs in patients with a history of seizures or considerable preoperative cerebral damage; these drugs should be continued indefinitely if postoperative seizures occur or for several months if they do not. The use of any medication should be weighed against its side-effect profile and the patient’s comorbidities.
Respiratory Support
Airway and breathing are the initial concern in preventing secondary neurologic injury and should therefore be integral to the initial assessment of any postoperative patient. In the neurosurgical patient, control of both oxygen and carbon dioxide is critical.
Hypoxia and Hypercarbia in Brain Injury
Hypoxia can be detrimental by two mechanisms. First, in areas of the brain with cerebrovascular compromise, hypoxemia may be poorly tolerated, with subsequent neuronal infarction if not corrected. Second, hypoxemia may exacerbate intracranial hypertension. The normal compensatory mechanism for hypoxia in the brain is vasodilation. This seems to be a regional phenomenon and may be controlled by regional factors. Therefore, it is reasonable to assume that areas of maximal vasodilatation and decremental blood flow will not tolerate additional hypoxemia, because there may be few, if any, further compensatory mechanisms available to the neuronal tissue. If further vasodilatation is possible, however, subsequent cellular damage may occur because of increases in intracranial pressure, with consequent decrease in regional or global blood flow and altered cellular structure, leading to further edema and increases in ICP. Hyperoxia, on the other hand, is also theoretically detrimental because of the abundance of free radicals created as a result of ischemia and reperfusion. However, with the data available, it still seems most reasonable to err on the side of too much oxygen rather than too little.
Carbon dioxide has an even more profound effect on cerebral vasculature. CBF is linearly related to PaCO 2 when it is between 20 and 60 mmHg. Therefore, decrements or increments in PaCO 2 will either decrease or increase CBF, respectively. This change in CBF has a direct effect on blood volume and therefore on ICP. It would then seem that when an increased ICP was detrimental, a lower PaCO 2 would be beneficial, as it would directly lower the ICP; however, the lower ICP is at the direct expense of blood flow, which is exactly the parameter that the clinician is attempting to maintain. The relation between ICP and PaCO 2 is even more complicated in the injured brain. When injured, the cerebral vasculature loses autoregulation and increased PaCO 2 may not increase blood flow to injured areas because arterioles in that area may be maximally dilated. There is also the theoretical issue of steal, as noninjured areas may increase their blood flow with increases in PaCO 2 at the expense of maximally dilated areas of injured brain. On the other hand, lowering ICP through decreases in PaCO 2 may counterintuitively cause hyperperfusion of injured areas because vasoconstriction does not occur as readily there as it does in areas of normal brain where ischemia may otherwise result.
Airway Assessment and Management
Decreased Level of Consciousness
Reduced level of consciousness (GCS < 10) correlates with the need to protect the airway by endotracheal intubation to prevent passive aspiration and intermittent airway obstruction. This was demonstrated in studies that used ICP monitoring after acute hemispheric stroke, in which the development of decreased level of consciousness was not correlated with a rise in ICP but was more closely correlated with edema and tissue shifts.
Raised Intracranial Pressure
Patients presenting with acutely raised ICP often require control of the airway as the initial therapeutic intervention. Preventing hypoxemia, hypercarbia, and acidosis can minimize secondary neurologic damage from raised ICP. A decreased level of consciousness (GCS < 9) is associated with a decreased ability to protect the airway and suggests additional potential benefit from mechanical hyperventilation to control raised ICP. Potential cerebral herniation is associated with raised intracranial pressure and brainstem injury, which impairs airway reflexes, coughing, and ventilatory drive. However, laryngoscopy, hypoventilation, struggling, and the use of succinylcholine without using a small dose of nondepolarizing muscle relaxant to prevent muscle fasciculation have been shown to raise ICP.
Brainstem Lesions
Brainstem disease may lead to several well-defined disorders of breathing and require endotracheal intubation. Patients with basilar artery infarction may suffer from obstructive or mixed apnea or impaired airway reflexes, which lead to positional airway obstruction or repeated aspiration. The dorsolateral medulla is primarily responsible for the integration of effective rhythmic breathing and as long as this area, which includes the nucleus ambiguous and solitarius, is not affected by the ischemic infarct, central respiratory control may be relatively normal. Thus, even locked-in patients with rostral brainstem infarction may be left with a relatively normal respiratory drive if infarction spares the dorsal lateral medulla. Disruption of automatic breathing may result from damage to the lateral medulla and pontine tegmentum caudal to trigeminal outflow. Lateral medullary stroke is most commonly the result of occlusion of the distal vertebral or posterior inferior cerebellar artery, and large infarcts involving the dorsolateral medulla may be associated with fatal apnea. These patients may suffer from mild hypoventilation while awake, which can be reversed voluntarily. Respirations may cease entirely during sleep.
Spinal Cord Lesions
Priority is given to simultaneous airway assessment and management. Immobilization of the spine must be ensured until definitive radiographic studies are obtained. Listening for stridor caused by partial airway obstruction and feeling for air movement can determine adequacy of the airway. Patients who can speak without stridor or hoarseness usually have unobstructed airways. Some patients who demonstrate airway obstruction will respond to a jaw-thrust maneuver; however, during this process, spine manipulation must be avoided. Many patients with cervical cord lesions will require at least temporary endotracheal intubation. A significant proportion of spinal cord injured patients have associated head injuries and depressed levels of consciousness. Patients who are stuporous or unconscious are at increased risk of developing aspiration pneumonitis. In the patient with spinal cord injury, this risk is compounded by gastrointestinal paresis that develops soon after the injury. Patients with significant chance for regurgitation and aspiration require intubation or tracheotomy to protect their airway. Gastric atony should be suspected and managed with nasogastric tube suction until it resolves.
Sedation Strategies
Once a decision has been made to reintubate or to continue mechanical ventilation, the role of sedation becomes an issue because agitation, increased physiologic stress, and inadvertent airway extubation may impair neurologic recovery. However, sedation hinders the neurologic examination and impairs functional monitoring. The perfect sedative would allow complete cooperation from an alert, awake, yet passive patient, would take effect immediately, and would dissipate immediately when infusion is stopped. The oldest agents are the barbiturates, such as thiopental. Although thiopental’s efficacy is limited because tissue redistribution causes prolonged sedation after cessation of drug infusion, it has cerebral-protective properties by lowering metabolic rate and decreasing ICP.
Benzodiazepines (such as midazolam) are commonly used. These gamma-aminobutyric acid (GABA)ergic drugs cause sedation and amnesia. They retain some antiepileptic properties, decrease cerebral metabolic rate, and can be relatively short acting. They have the added benefit of being reversible with flumazenil but at the expense of potentially lowering the seizure threshold. The main disadvantages are that benzodiazepine use is associated with delirium and hypotension.
Short-acting opioids (such as fentanyl, alfentanil, or remifentanil) may also be used for their sedative properties. They have the added bonus of conveying analgesia (they do not, however, consistently confer amnesia) and are also reversible with naloxone. Patients remain fairly cardiostable after dosing and opioids blunt respiratory responses such as dyspnea, coughing, or gagging in ventilated patients. These drugs become more problematic with lengthier infusion because all have context-sensitive half-lives (except remifentanil). Remifentanil, however, can be quite expensive, which, coupled with its propensity to reduce MAP and thus CPP, may limit its use in the setting of prolonged postoperative sedation. Nevertheless, neurosurgical patients may benefit from its utility secondary to its rapid elimination and similar safety profile; this affords a predictable time to sedation elimination for rapid neurological assessment.
Newer agents include propofol and dexmedetomidine. Propofol is another GABAergic agent with both a very short half-life and sedative-hypnotic properties. Its main benefit is quick arousal times even with prolonged infusions. It may, however, have detrimental hemodynamic effects and can be rather expensive. Finally, dexmedetomidine, an alpha-2 agonist, grants both sedative and analgesic properties with minimal amnesia. Some hemodynamic depression can be seen (especially with bolus loading) and it also has a relatively long half-life when compared with propofol. Nevertheless dexmedetomidine, unlike the other sedatives, allows patients to be aroused from sedation with minimal stimulation and there is little to no respiratory depression.
Cardiovascular Support
Cerebral Perfusion and Arterial Blood Pressure
Under normal circumstances, cerebral blood flow is tightly autoregulated on the basis of regional tissue oxygenation, arterial carbon dioxide, cerebral glucose metabolism; therefore, oxygen delivery, glucose delivery, and carbon dioxide elimination are maintained despite wide changes in arterial pressure. However, after neuronal or vascular injury, as seen in postsurgical changes, cerebral perfusion may be uncoupled from autoregulatory mechanisms and, therefore, will be tied directly to arterial blood pressure. In these circumstances, the arterial–intracranial pressure curve will change to reflect linear increases in ICP because arterial pressure increases until compensatory mechanisms are overwhelmed, at which point ICP increases exponentially. For these reasons, increased systemic arterial pressure may exacerbate, or even cause, cerebral edema or hemorrhage just as decreased systemic pressure may worsen ischemia. It may be necessary, then, to control systemic arterial pressure within tightly defined parameters until neuronal or vascular repair has been accomplished sufficiently to reestablish cerebral blood flow autoregulation.
Hypertension and Brain Injury
Postoperative neurosurgical patients often display varying degrees of hypertension as they recover from anesthesia and postsurgical changes to the brain. The essential question is whether the elevated systemic arterial pressure is detrimental to (or, on the contrary, sustains) cerebral perfusion. When the brain undergoes periods of relative hypoperfusion, systemic arterial pressure may be elevated to maintain adequate cerebral perfusion pressure. At some point along this perfusion–pressure curve, additional systemic pressure no longer increases cerebral perfusion but instead begins to reduce blood flow because the surrounding cranium is not compressible. It is often very difficult to determine immediately and empirically where along the pressure–perfusion curve any individual patient is lying. Therefore, it may be necessary to judiciously manipulate the systemic arterial pressure while vigilantly monitoring the patient for neurologic changes and for indications of improvement or deterioration. Additionally, an estimation of the potential for postsurgical complications (e.g., hemorrhage, edema) should be made to determine whether to lower or to increase a patient’s arterial blood pressure. These factors can make blood pressure manipulation precarious, and clinical decisions are best made for individual patients after considering all significant patient and surgical factors.
Inotropes and Vasodilators
A variety of pharmacologic agents are at the disposal of the intensivist when systemic arterial blood pressure must be maximized in attempts to maximize cerebral perfusion. Inotropes such as epinephrine, norepinephrine, dopamine, dobutamine, and phenylephrine demonstrate varying degrees of alpha- and/or beta-adrenergic agonism; a full discussion of all inotropes commonly employed is beyond the scope of this chapter. As such, the selection of blood pressure-augmenting agent is best made in the specific clinical context in which it is to be used while weighing the drug’s intended benefits with its expected side effects. General considerations include the patient’s comorbidities, particularly cardiac and renal function, as well as whether the patient’s arterial pressure and cardiac output are best maintained or enhanced through alpha (i.e., peripheral) or beta (i.e., cardiac) effects.
When induced hypotension is required, the clinician can choose from vasodilators, angiotensin-converting enzyme (ACE) inhibitors, and sympatholytics. Commonly utilized vasodilators include nitroglycerin, sodium nitroprusside, hydralazine, and nicardipine; however, in general, nitroglycerin and nitroprusside are avoided for the postoperative neurosurgical patient because of their tendency to dilate cerebral vasculature, which can raise intracranial pressure and thus decrease cerebral blood flow. Nicardipine, a calcium-channel blocker (CCB), can theoretically worsen intracranial pressure, but it has been found to decrease systemic arterial pressure reliably without clinically significant intracranial pressure changes and it has the additional benefit of potential cerebral protective properties. Similarly, clevidipine, a newer third-generation CCB, has found favor both intraoperatively and in the NSICUs because of its more rapid onset and shorter half-life than nicardipine; in addition to its rapid titratability, it may also be advantageous because of the lower drug volume required to achieve an effect, which may be particularly useful in critically ill, hypervolemic patients.
ACE inhibitors have also been shown to decrease systemic blood pressure and have additional benefits in patients with cardiac comorbidities. The sympatholytics include beta-adrenergic blockers (i.e., labetalol and metoprolol) and centrally acting alpha-2 agonists (i.e., clonidine). Clonidine is not routinely used in the immediate postoperative phase because of its long-acting and sedative effects. However, it has potential uses in patients with refractory or poorly controlled hypertension, ongoing drug abuse, or signs and symptoms of narcotic withdrawal.
Fluid and Electrolyte Support
Hypovolemia and Cerebral Perfusion
Hypovolemia may lead to hypotension and thereby worsen ischemia because of decreased perfusion of organs. This is also true in the brain. With regional brain injury and ischemia, cerebral blood vessels may be maximally dilated in an attempt to maintain perfusion and lose autoregulation, which ties perfusion directly to mean arterial pressure. In states of hypovolemia, hypotension may be induced despite compensatory mechanisms (decreased blood flow to peripheral tissues and nonessential organs, increased movement of water intravascularly), thereby worsening ischemia in poorly perfused neuronal tissue. Therefore, patients with hypovolemia, whatever its cause, warrant aggressive fluid resuscitation.
In the immediate postoperative period, hypovolemia is most likely related to blood loss, intraoperative diuresis with mannitol and/or furosemide, or inadequate resuscitation. Blood loss, whether it occurred intraoperatively or is ongoing postoperatively, should be replaced with crystalloid solution until a lowest limit of allowable hemoglobin is reached (see later), at which point blood transfusion should begin. Intraoperative fluid manipulation to decrease brain water generally consists of osmotic diuresis with mannitol and/or loop diuresis with furosemide. Use of either of these agents can result in depletion of total body water in the postoperative patient and potentially hypovolemia with resultant hypotension. It then becomes necessary to replace body water without worsening cerebral edema. In general, this is done through the use of iso-osmolar fluid solutions, such as normal saline, and 5% albumin.
The use of hyperosmolar saline solutions has been evaluated during resuscitation. These solutions expand intravascular volume with the benefit of a favorable osmotic pressure gradient in the cerebral vasculature. However, a potential disadvantage is the propensity to cause hypernatremia with even modest amounts of volume. Thus, they should be carefully titrated with frequent serum sodium assessments.
Finally, colloids have long been used to increase intravascular volume while attempting to avoid third-space loss. These include both natural colloids (fractionated plasma protein, albumin, and blood products) as well as synthetic colloids (hetastarch). Although there is little evidence in neurosurgical patients, the synthetic colloids are largely avoided because of antiplatelet effects in vivo and concerns about clinical bleeding. In general, fractionated plasma protein colloids and albumin are chosen when there is concern for excessive crystalloid use without indication for the use of blood products.
Hyponatremia, Edema, and Seizures
Hyponatremia has been associated with delirium, cerebral edema, and seizures. Usually, sodium levels below 120 mEq/L are required for hyponatremia alone to cause a significant decline in neurologic status. However, all cases of hyponatremia merit attention and being evaluated for a cause. The three major reasons for the development of new-onset hyponatremia in the postoperative neurosurgical patient are syndrome of inappropriate antidiuretic hormone (SIADH), cerebral salt wasting, and inappropriate free water administration.
It is helpful to describe hyponatremia as it relates to intravascular volume. SIADH most commonly falls in the hypervolemic category, cerebral salt wasting in the hypovolemic category, and dilutional in the euvolemic category. An assessment of volume status should be made from change in weight, the fluid balance record, or measurement of ventricular filling pressure with central venous or pulmonary capillary wedge pressure. It may also be helpful to check the osmolarity of both serum and urine and to check the urine sodium to make a distinction between the causes. Both SIADH and cerebral salt wasting increase urine sodium, differentiating them from dilutional causes. SIADH is associated with a high (often very high) urine osmolarity, while the urine osmolarity is typically normal in cerebral salt wasting.
It is, of course, important to rule out other causes of hyponatremia (e.g., medication, renal failure, excess loss from fever, diarrhea, or vomiting, or endocrine disorder such as hypothyroidism or cortisol deficiency) because the treatment is dictated by the cause. If free water is being given, the practitioner should switch the intravenous fluid to an iso-osmolar or a hyperosmolar salt solution, depending on the degree of hyponatremia. SIADH is generally treated by fluid restriction; however, this may be inappropriate in the hemodynamically unstable patient. In this case, administration of hyperosmolar saline or the addition of extra sodium to the patient’s diet may be more desirable. Cerebral salt wasting is treated by replacing urine sodium lost with normal or hypertonic saline. It is important to begin therapy before reaching critically low serum sodium levels, as correction of hyponatremia should be performed gradually to avoid the complication of central pontine myelinolysis. Usually, correction of 1 to 2 mEq/L/h is recommended, up to 12 mEq/L/day.
Routine Fluid Management
Unlike other tissues, which respond to both hydrostatic and oncotic pressures, the brain’s water content is regulated most by serum osmolarity, in large part because of the blood–brain barrier. Therefore, to avoid the possibility of producing or worsening cerebral edema, it is important to avoid hypo-osmolarity in the serum. This is accomplished by the administration of an isotonic intravenous fluid such as normal saline or a balanced salt solution such as Normosol. The use of colloid solutions is not recommended because oncotic pressure is not as useful in regulating brain water. Maintenance of euvolemia is imperative in the typical postoperative neurosurgical patient because both hypovolemia and hypervolemia may have detrimental side effects. Hypovolemia can lead to hypotension and, therefore, hypoperfusion. Hypervolemia may increase intracranial pressure, leading to hypertension or an edematous state. Finally, dextrose-containing fluids are not recommended for routine use because the presence of hyperglycemia has been shown to worsen outcomes in cerebral injury and in general they add to the amount of hypo-osmolar fluid administered.
Diabetes Insipidus (DI)
DI results from dysfunction of the pituitary gland through primary causes or, more likely in the postoperative neurosurgical patient, from ischemia, tumor, or surgical manipulation. It should be suspected when urine output is higher than expected and serum sodium appears to be rising. DI is the most common cause, behind iatrogenic causes, of hypernatremia in the neurosurgical patient. The diagnosis is suggested by a large hypotonic urine volume in the presence of hyperosmolar hypernatremia leading to a hypovolemic state. Treatment is aimed at replacing total body water with hypo-osmolar salt solutions. This deficit should be replaced over 24 hours at a rate no faster than 2 mEq/L/h because too-rapid correction may result in seizures, delirium, or cerebral edema, presumably from what the brain perceives to be a hyponatremic state. Additionally, low dosages of arginine vasopressin given by continuous infusion are very effective in correcting both hypovolemia and hyponatremia in patients with diabetes insipidus, brain-injured patients, and children. Common protocols call for administering 1 or 2 IU/L of arginine vasopressin in hyponatremic fluid at hourly urine output rates plus 10% to correct hypovolemia and hyponatremia gradually. Similarly, a titratable infusion of 0.01–0.03 IU/h vasopressin may be utilized while replacing urine output in a 1:1 ratio with hyponatremic fluid.
Hematologic Support
Role of Anemia in Cerebral Ischemia
Normovolemic anemia has been shown to increase cerebral blood flow by increasing cardiac output and cerebral vasodilation to ensure an adequate oxygen supply to the brain. Decreased blood viscosity may also contribute to the increase in cerebral blood flow. The compensatory increase cerebral hemodynamics may be impaired by hypovolemia associated with perioperative blood loss. However, if adequate intravascular volume is maintained through the use of intravenous crystalloids, even moderate anemia has little effect on cerebral ischemia. In fact, it has not been shown that even severe anemia (hemoglobin level < 6 g/dL) can cause long-term or permanent cerebral injury alone. Higher hemoglobin levels may, in fact, be detrimental in some pathologic processes, as in subarachnoid hemorrhage–induced vasospasm and diffuse axonal injury. It is also possible, at least in theory, that normovolemic anemia may cause detrimental increases in cerebral blood flow in patients with raised intracranial pressure, but this has not been studied. Finally, only one human study has demonstrated adverse effects of severe anemia (hemoglobin level < 6 g/dL), consisting of mild cognitive changes in healthy volunteers that was readily reversible by increasing the amount of inspired oxygen.
The American Society of Anesthesiologists has published guidelines for the administration of blood products, with emphasis on patient-specific transfusion criteria in the setting of prior blood transfusions, history of a known coagulopathy or thrombotic event, and risk factors for organ ischemia that might alter thresholds for transfusion. They recommend first maintaining normovolemia by the use of crystalloids and colloids; additionally, the application of available pharmacologic therapies such as erythropoietin, prothrombin complex concentrates, and antifibrinolytic agents should be considered when appropriate to minimize transfusions and their subsequent risks. Red blood cell transfusions are recommended for hemoglobin levels 6–10 g/dL based upon an ongoing assessment of blood loss, intravascular volume, end-organ perfusion imbalances, and cardiac reserve. Fresh frozen plasma transfusions are recommended for urgent reversal of anticoagulation, known coagulation factor deficiencies (when specific concentrates are unavailable), microvascular bleeding in the presence of an elevated prothrombin time (an international normalized ratio [INR] >2, in the absence of heparin), and microvascular bleeding after the replacement of more than one blood volume when PT or PTT cannot be obtained. Platelet transfusions are recommended in surgical patients with platelet counts of less than 50,000/μL or despite a theoretically adequate platelet count in the setting of known of suspected platelet dysfunction; this might be appropriate following cardiopulmonary bypass or when undergoing surgery where minimal bleeding may cause major damage, such as in neurosurgery. Aggressive correction of clotting abnormalities in actively bleeding patients with fresh frozen plasma, platelets, and/or cryoprecipitate, as indicated by clotting studies, seems good clinical practice.
Thromboprophylaxis
Deep venous thrombosis (DVT) has three classic antecedents: venous injury, stasis, and hypercoagulability. The postoperative neurosurgical patient is prone to many risk factors for development of DVT, including trauma, immobilization, tumors, acute stroke, estrogen use, obesity, age greater than 40 years, and acquired coagulopathy. Several mechanical and chemical methods are used to prevent the development of DVT. Pneumatic compression devices and compression stockings have few contraindications and have proven efficacy after a number of types of surgery, including neurosurgery. Additionally, mobility may be of benefit: for mobile patients, an early ambulation strategy may be used and for those in a nonambulatory state, passive motion exercise may be utilized. Chemoprophylaxis with enoxaparin or low-dose subcutaneous heparin have been proved valuable in reducing the burden of thromboembolic events without significant increases in bleeding occurrences; current meta-analyses suggest that the benefits of chemoprophylaxis in neurosurgical patients outweigh the risks. It is our practice to use pneumatic compression devices with elastic compression stockings for every patient not on subcutaneous heparin. Physical therapy and ambulation are encouraged in every patient. Heparin is used in those patients with minimal risk of intracranial hemorrhage formation.
Gastrointestinal and Endocrine Support
Glucose and Cerebral Injury
Glucose is the main substrate of the brain and is actively transported across the blood–brain barrier by a carrier-mediated mechanism and by diffusional mechanisms that can be affected by blood glucose levels. Hypoglycemia is disadvantageous to ischemic brain, but hyperglycemia also worsens cerebral ischemia and neuronal damage after both head trauma and stroke. It is less clear how, or at what level of hyperglycemia, global cerebral ischemia becomes worse, and the data are even less clear when applied to focal injury. It is thought that organic acid accumulation in nonischemic areas may lead to cellular injury. Another theory is that lactate production from anaerobic metabolism in areas of ischemia worsens existing damage. Some data suggest that any amount of hyperglycemia (serum glucose greater than 120 mg/dL) may be detrimental. It is not clear whether maintenance of normal glucose levels through insulin therapy improves outcome, neurologic or otherwise. However, it is common practice to keep serum glucose below 200 mg/dL in patients with known, or at risk of, cerebral ischemia. It is not uncommon to attempt to keep blood glucose in the range of normal or less than 140 mg/dL, usually by sliding-scale regular insulin injections or regular insulin infusion protocols.
Management of Diabetes Mellitus
Patients with premorbid diabetes mellitus may have serum glucose levels that are quite difficult to control. These patients are very likely to benefit most from tight glycemic control in the setting of cerebral ischemia, but studies suggesting how to maintain the serum glucose, and to what level, are scant. In our own practice, we routinely check every patient’s serum glucose level every 6 hours after admission for at least the first 24 hours, and patients are placed in an insulin infusion nomogram after two consecutive blood glucose readings greater than 200 mg/dL. It has also become our practice to check glucose levels every 3 hours for the first 24 hours after admission or after beginning feeding for patients who are thought to be at high risk of glucose intolerance.
Nutrition
Neuronal tissue undergoes very high rates of oxidative metabolism with little or no stored energy, relying almost solely on blood-delivered glucose. Glucose undergoes active transport from the capillaries in areas with an intact blood–brain barrier. Throughout the brain, glucose also enters neuronal tissue through diffusion mechanisms. Areas of the brain where the blood–brain barrier has been disrupted (e.g., by ischemia) may be totally reliant on diffusion mechanisms for their glucose supply. It is critical that nutritional demands be continually met in the critically ill. In the immediate postoperative period, nearly all neurosurgical patients receive nothing by mouth until they have been fully assessed and allowed to recover from the effects of anesthesia and postsurgical changes. If they are then able to self-feed, they are allowed a trial period, during which it is determined whether their nutritional demands can be adequately met. If self-feeding is not feasible, nasogastric tube feedings are initiated and a determination is made about the long-term prognosis for adequate self-nutrition. If enteral nutrition is not successful, (i.e., if there are persistent large residual volumes after feeding or continuing lack of bowel sounds), parenteral nutrition via central venous catheters should be considered.
Postoperative Nausea and Vomiting
It is common for neurosurgical patients, especially those who underwent intracranial surgery, to have significant nausea and vomiting. This may result from the effects of anesthesia centrally or peripherally or from central effects of the surgery itself. Nausea can be uncomfortable for the patient and vomiting can be catastrophic in cases of craniotomy; the act of vomiting may lead to transient increases in intracranial pressure and place the patient with potentially already decreased airway reflexes at an increased risk of aspiration. Nearly all antiemetics, including promethazine, corticosteroids, droperidol, and serotonergic drugs (e.g., ondansetron), have some effect on postoperative nausea and vomiting. Ondansetron has been shown to be particularly efficacious with respect to postoperative nausea following craniotomy, if given as a prophylactic. Aprepitant, a substance P antagonist, has also shown great effect as a premedication in the neurosurgical population and may be more efficacious than ondansetron when either drug is combined with dexamethasone.
Infectious Disease
Infectious Risks of Neuraxial Devices
Several neuraxial catheters may be encountered in the postoperative neurosurgical patient, the most common of which is placed via a ventriculostomy. The predominant concern of any externalized neuraxial device is infection. Historically, available literature has suggested that infection rates rise quickly at approximately 1 week after placement ; however, a recent meta-analysis has suggested that this rate is lower than previously thought and may not be associated with frequency of manipulation or duration of catheter dwelling. In fact, according to Ramanan et al., studies prior to 2000 show a remarkably increased ventriculostomy-associated infection rate; perhaps this can be attributed to emphasis placed on infection prevention modalities since that time. In the interim, a number of different strategies have been used to combat the ongoing concern of infection associated with ventriculostomy, including intrathecal antibiotic infusion, tunneling of the catheter, and changing the catheter weekly. However, there are limited data to suggest that any method actually improves infection rates or patient morbidity or mortality, and each approach has its disadvantages, including microbial resistance and the risk of having to repeat the procedure.
References

Full access? Get Clinical Tree
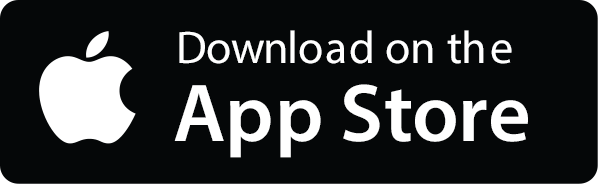
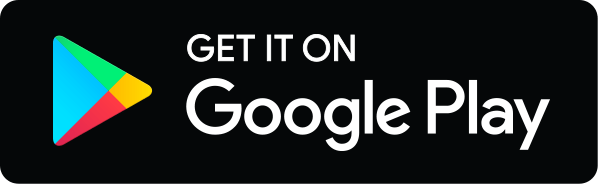
