html xmlns=”http://www.w3.org/1999/xhtml” xml:lang=”en”>
Translational Neuroscience in Pediatric Critical Care
Courtney L. Robertson, MD, FAAP, FCCM
Objectives
- Define translational neuroscience research
- Highlight potential barriers to successful translational neuroscience research in pediatric critical care
- Describe the biological process of brain development as it relates to pediatric brain injury research
- Discuss examples of specific therapies for pediatric acute brain injury with potential for translation to clinical use
Key words: developmental brain injury, neuroprotection, progesterone, cyclosporin A
The definition of translational research has evolved significantly over the last 2 decades of medical research. The early description of “translation” involved the concept of bench-to-bedside research, bringing new therapies or techniques from preclinical animal studies into clinical trials and ultimately improving disease outcomes in humans.1 More recently, investigators have recognized that effective translation also involves ensuring that new therapies are actually applied clinically to the intended patient population. Effective delivery of new therapies poses a significant challenge in modern healthcare. It is estimated that adults in the United States receive only about 50% of recommended services for preventive health and chronic disease care.2 In this study, even therapies with proven clinical benefit and relatively low cost, such as receiving aspirin therapy after a myocardial infarction,3 had a low success rate (~60% of patients surveyed). The evolution of the definitions of translational research has challenged investigators and led to some confusion, especially in terms of priorities for public funding. Some scientists have suggested that these 2 fields of translational research are “alike in name only.”1 The Institute of Medicine’s Clinical Research Roundtable was one of the first groups to describe these 2 distinct aspects of translational research,4 depicting them as “translational blocks” and ultimately challenging the US clinical research enterprise. In recent years, these translational research approaches have been labeled as T1 and T2, with some further subdividing T2 research into T2, T3, and T4 components.5 T1 research involves the transfer of fundamental basic science into clinical evaluation and clinical trials. T2 research involves dissemination of T1 results into daily clinical practice. The US National Institutes of Health (NIH) has placed a high priority on translational research by highlighting its importance in the NIH Roadmap for Medical Research in 20036 and by funding NIH Clinical and Translational Science Awards (CTSAs) to more than 50 academic institutions since this time.7
The Neuroscience Field and Pediatrics
In the field of neuroscience, translational research is often similarly defined, encompassing experimental and clinical studies of nervous system disorders. The mission of the National Institute of Neurological Disorders and Stroke (NINDS) Office of Translational Research is to “facilitate the preclinical discovery and development of new therapeutic interventions for neurological disorders.”8 The NINDS and its Office of Translational Research have recognized both T1 and T2 challenges in their mission. Specific challenges emerge when considering translational neuroscience research in the pediatric population. These challenges are summarized in a recent review by Szilagyi5 (p. 77) and include limited patient populations with fewer investigators, insufficient leadership positions held by pediatric researchers within clinical research centers, and the regulatory and institutional review board challenges of studying a “vulnerable population.” Some of these challenges have been addressed by support for pediatric research within the CTSA program.
In 2007, the CTSA Pediatric Oversight Committee was formed, allowing for coordination of pediatric translational research on a national level.7 Despite this and other national efforts, the percentage of public research funding (NIH, the Agency for Healthcare Research and Quality) devoted to pediatrics has decreased from 13.1% in 1993 to 10.8% in 2008.9 An excellent series of recommendations for enhancing pediatric translational research can be found in the review by Szilagyi,5 who concluded that these changes can “bridge the promise of scientific discovery with the reality of improved child health.”
What Is Unique About the Developing Brain?
Although there are many T2-related challenges to translational neuroscience in pediatrics, the rest of this chapter is devoted primarily to T1 aspects. One of the most important considerations in the field of pediatric neuro-science is that the maturing brain has many features different from those of the adult brain. Some of these unique features confer specific vulnerabilities to acute brain injury, while other features can result in resistance to injury or improved capacity for long-term recovery. Many of the pathological cascades that are triggered following acute brain injury have developmental regulation.10 For example, the balance of excitatory and inhibitory neurotransmission is different in the young brain. The very young brain has a heightened sensitivity to excitotoxicity after hypoxic–ischemic insult,11,12 which may be due in part to the age-dependent regional expression of glutamate receptors (NMDA, AMPA, kainite).13,14 Furthermore, the γ-aminobutyric acid (GABA) receptor, which is responsible for inhibitory neurotransmission in the adult brain, can be excitatory in early development.14,15 Another important consideration in developmental brain injury is that the antioxidant capacity and the ability to up-regulate antioxidant enzymes following injury are reduced in the young versus mature brain.16,17 Finally, cerebral energy metabolism has important developmental features. For instance, cerebral glucose utilization is low postnatally and increases throughout maturation, and this correlates with maturational increases in cerebral mitochondrial enzyme content and activity as well as cerebral blood flow.18-22 The young brain may have improved potential for use of alternative fuels for energy metabolism, such as ketones and lactate,21,23-25 which may be especially important after brain injury, allowing for metabolic rescue and preservation of adenosine triphosphate production.26-28 A more in-depth discussion of developmental brain injury and neuroprotection can be found in a recent review.10
There are many practical considerations when designing pediatric neuroscience studies that translate therapies into multicenter clinical trials. These considerations are nicely summarized in a review written by Natale et al.29 Although this review was focused on experimental trials involving pediatric traumatic brain injury (TBI), the concepts are relevant for most clinical trials in pediatric neurocritical care. One of the main points of this review was that the patient population in pediatric acute brain injury is quite small, relative to the adult population, which may necessitate large, multicenter approaches. The authors highlight the fact that fewer than 900 children were enrolled in experimental studies of severe TBI in the last 25 years and that the average enrollment in most trials was 1 child or fewer per month per center. The authors also discuss other key features, and challenges, of pediatric brain injury studies, such as the family perspective when informed consent is being obtained shortly after they have been told of their child’s injury.
What Neuroprotective Therapies Are Ripe for Translation to the Pediatric ICU?
In February 2008, a group of scientist from many biomedical disciplines met at a NIH-sponsored workshop titled “Combination Therapies for Traumatic Brain Injury.” The objective of this workshop was to identify possibilities for neuroprotection after severe TBI using combination therapies. The organizers cited the failure of more than 20 late phase 2 or phase 3 clinical trials for adult TBI in the last 2 decades. Several reasons for this failure were suggested, including inadequate preclinical models and inadequate translation of important issues, such as therapeutic windows and plasma levels between animals and humans. In the published proceedings from this workshop, Margulies et al suggested critical aspects of animal models that are necessary for meaningful clinical translation.30

Full access? Get Clinical Tree
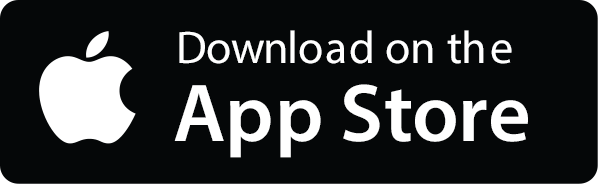
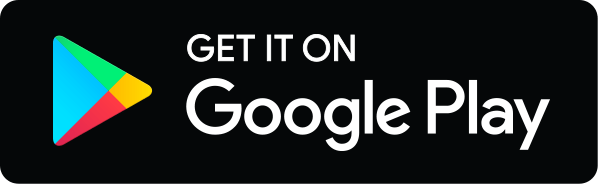