Neurophysiology and Autonomic Dysfunction in Cluster Headaches
Peter D. Drummond
Massimo Alessandri
NEUROPHYSIOLOGY OF CLUSTER HEADACHE
Neurophysiologic tools have been used to explore the function of central and peripheral neural pathways that may be involved in cluster headache (CH). Techniques include electroencephalography, evoked potentials, studies of threshold for nociceptive responses, and assessment of pain perception. In general, the neurophysiologic approach has shown that CH is associated with altered pain processing, not only in trigeminal circuits but also in other parts of the body.
ELECTROENCEPHALOGRAPHY AND EVOKED POTENTIALS
In an early electroencephalographic investigation, abnormal activity, generally focal activity, was identified between attacks in about 50% of cases with no further changes during the attack (24). More recently, evoked potential data have supported the notion of central nervous system involvement in CH. For example, the asymmetry of brainstem auditory-evoked potentials was greater in CH patients both during attacks and between attacks than in controls, presumably due to asymmetrical conduction in central acoustic pathways. In contrast, brainstem auditory-evoked potentials did not differ between controls and CH patients on lithium therapy (4). The amplitude of visual-evoked potentials was found to be reduced in the pain-involved hemisphere during the pain-free period (2), although another study failed to replicate this result (44). Somatosensory-evoked potentials to median nerve stimulation were recorded in CH patients during and between severe attacks induced by intravenous administration of 40 μg of histamine. During the attack, the amplitude of the N1-P2 complex was lower than in patients outside the attack and lower than in normal subjects (16), presumably because powerful pain modulation mechanisms were triggered during the attack.
Van Vliet et al. (63) recently investigated trigeminal somatosensory-evoked potentials in patients with episodic CH. Electrical stimuli were delivered to the corner of the mouth at three times the sensory threshold. Evoked potentials were delayed during bouts, particularly on the symptomatic side, consistent with a deficit in processing trigeminal sensations.
Blink Reflexes
The blink reflex is a trigeminofacial reflex obtained by electrical or mechanical stimulation of the supraorbital nerve. The reflex consists of two responses: an early ipsilateral response (R1) and a late bilateral response (R2). The R2 is a nociceptive reflex mediated by a polysynaptic circuit through the pons and lateral medulla (26). The amplitude of the R2 response to contralateral stimulation was found to be significantly lower on the symptomatic than asymptomatic side during bouts of CH (46).
The R2 component is modulated both by segmental and heterosegmental influences and can be inhibited by prior stimulation of the supraorbital nerve or a peripheral nerve (47). The recovery of the inhibited R2 response can be investigated by increasing the delay between the conditioning stimulus responsible for the inhibition and the supraorbital stimulus producing the R2 response. The recovery curve of R2 after paired supraorbital stimuli depends on the excitability of trigeminofacial circuits (43), whereas limb stimulation inhibits R2 by activating reticular pathways (62). In CH patients, the R2 recovered more rapidly on the symptomatic side after paired supraorbital stimuli (34), possibly reflecting sensitization in the spinal trigeminal complex. When index finger stimulation was used to condition the supraorbital stimulus, R2 recovery was more
rapid bilaterally in CH patients than controls. The bilateral deficit in R2 inhibition may reflect hypoactivity of reticular nuclei due to reduced central opioid activity (34).
rapid bilaterally in CH patients than controls. The bilateral deficit in R2 inhibition may reflect hypoactivity of reticular nuclei due to reduced central opioid activity (34).
Corneal Reflex
To elicit the corneal reflex, constant current stimuli are delivered from a thin cotton thread soaked in saline solution, which floats in tear fluid on the cornea. The response is recorded from the orbicularis oculi muscles. The latency, amplitude, and duration of the corneal reflex were found to be normal in CH patients both during the active phase and in remission (51). However, during the active phase, the threshold of the corneal reflex was significantly reduced, suggesting an increased excitability of trigeminal nociceptive neurones or facial motor neurones. These findings support the notion of a reversible impairment in activity of the trigeminal pain control system during the active phase of CH (51).
Infratrochlear Nerve Stimulation
High-intensity transcutaneous electrical stimulation applied to the emergence of the infratrochlear nerve induces a constriction of the ipsilateral pupil that is slow in onset and long-lasting. Miosis, which is homatropine resistant, has been attributed to an antidromic activation of the iris sensory fibers (17). In CH sufferers during the active period, transcutaneous electrical stimulation of the infratrochlear nerve elicits normal pupillary constriction in the asymptomatic eye, whereas the response in the symptomatic eye is minimal (15). This observation suggests that repeated attacks of CH deplete neuropeptide stores in trigeminal nerve terminals. If so, miosis during CH attacks is unlikely to be due solely to local release of neuropeptides.
Nociceptive Flexion Reflex
The nociceptive flexion (RIII) reflex has been used to investigate the integrity of antinociceptive mechanisms in several pain conditions, including primary headaches (53). The RIII reflex is obtained by stimulating the sural nerve at the retromalleolar level and recording the response from the biceps femoris. The RIII reflex threshold was found to be lower on the symptomatic than nonsymptomatic side both during (52) and between bouts of CH (40), implying a persistent impairment of the pain control system on the symptomatic side of the body. This impairment was associated with a phase shift in the circadian rhythm of the RIII threshold during episodic bouts of CH and with an absence of circadian variation in patients with chronic CH (40). Thus, impairment of the pain control system in CH may be associated with periodic failure of the mechanisms involved in regulating biologic rhythms (40).
Pain Perception
The pain threshold to electrical stimulation of the cornea was found to be lower than normal during the active phase of CH, particularly on the symptomatic side (51). Pain perception is also altered in other parts of the body. For example, Procacci et al. (45) detected cutaneous and deep hyperalgesia to electrical and mechanical stimuli on the symptomatic side of the body in patients with episodic CH. Moreover, pain or paresthesiae during 10 minutes of limb ischemia appeared earlier, was more intense, and lasted longer on the symptomatic than nonsymptomatic side. Pain perception thresholds at symmetrical points on each side of the head and over the deltoid muscle were assessed with a pressure algometer both in episodic and chronic CH (3). Patients had lower pain pressure thresholds than controls at all sites during bouts and in remission, consistent with the hypothesis of altered pain processing in CH. In addition, pressure pain thresholds were lower on the symptomatic than nonsymptomatic side during bouts of episodic CH, possibly due to inflammation in symptomatic scalp tissues or to trigeminal sensitization.
AUTONOMIC DISTURBANCES IN CLUSTER HEADACHE
CH is associated with a range of local and more general autonomic disturbances. Local signs of parasympathetic overactivity such as lacrimation and nasal discharge often develop during attacks. Signs of ocular sympathetic paralysis (miosis and ptosis) also develop in some patients during attacks and persist interictally together with vasomotor and sudomotor signs of sympathetic deficit. Paradoxically, however, sweating increases on the painful side of the face during attacks, particularly in areas of sympathetic deficit. In patients with ocular and thermoregulatory signs of sympathetic deficit, the symptomatic parts of the face are supersensitive to adrenergic and cholinergic drugs. Furthermore, changes in blood pressure and heart rate suggest that disturbances in cardiovascular control may develop during attacks.
Opinions diverge over the source of these autonomic disturbances. According to some, autonomic dysfunction originates centrally in association with a hypothalamic disturbance that compromises inhibitory pain control mechanisms. Others hold that venous stasis or an inflammatory process in the cavernous sinus accounts for the cyclical pain and associated autonomic disturbances. Another possibility is that many of the autonomic disturbances are secondary to trigeminal discharge during attacks of CH.
Disturbances in Blood Pressure and Heart Rate
Blood pressure typically increases whereas heart rate decreases during CH attacks (6). In addition, the variability of beat-to-beat changes in heart rate alters as the attack progresses. Two recent studies present a similar picture of the time course of these heart rate fluctuations. Tubani et al. (61) monitored heart rate continuously over 24 hours to capture one or more spontaneous attacks in seven patients. In each case, the high-frequency component of heart rate variability (mediated by parasympathetic activity) increased dramatically at the onset of the attack and returned toward baseline as the attack subsided. Conversely, the low-frequency component of heart rate variability (related to blood pressure and baroreflex control and mediated both by sympathetic and parasympathetic activity) dropped slightly at the onset of the attack, then rose toward the end of the attack. De Marinis et al. (6) investigated changes in heart rate variability during attacks in eight CH patients. The low-frequency component of heart rate fluctuations increased before the onset of headache (in parallel with a mild tachycardia) but then fell during the attack, whereas the high-frequency component increased as the attack progressed.
The mechanism of these disturbances in cardiovascular control is uncertain. Perhaps an increase in parasympathetic activity at the onset of the attack forms part of a normal baroreflex mechanism that attempts to reduce pain-evoked increases in blood pressure. Alternatively, activation of trigeminal depressor or oculocardiac reflexes might contribute to bradycardia. A sustained fall in arterial oxygen saturation precedes attacks of CH (32). Since heart rate increases in CH patients during oxygen deprivation (56), hypoxia before attacks could contribute to tachycardia before the onset of the headache.
To investigate whether a disturbance in sympathetic vasoconstrictor outflow might contribute to cardiovascular instability, Nordin et al. (42) recorded sympathetic traffic in the peroneal nerve during attacks of CH. Increases in blood pressure that corresponded with pulse-synchronous bursts of sympathetic activity indicated that an increase in sympathetic vasoconstrictor outflow to muscles contributed to the rise in blood pressure. This response is almost certainly secondary to pain, because the same relationship between bursts of sympathetic activity in the peroneal nerve and blood pressure was observed during eye pain induced by the instillation of soapy water (41). Pain or the anticipation of pain might also trigger transient tachycardia at the onset of attacks.
Cardiac arrhythmias develop during CH attacks in some patients (37), possibly in association with changes in autonomic balance (48). Alternatively, a change in breathing patterns during intense pain could evoke cardiac arrhythmias and contribute to changes in heart rate variability. Respiratory sinus arrhythmia during deep breathing, an index of vagal modulation of heart rate, does not change consistently during attacks of CH (30).

Full access? Get Clinical Tree
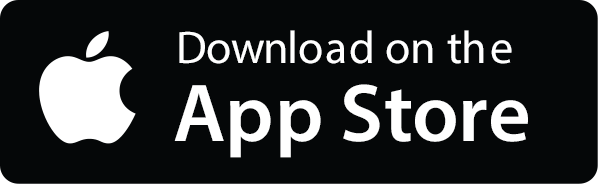
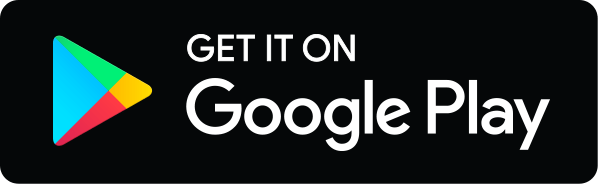