Fig. 34.1
Chest radiographs taken preoperatively of the patient in Case 1 showing the spine instrumentation
After failure of conservative therapy and facet joint injections, she was scheduled for posterior exploration of the original fusion T6–8 and posterior fusion T4–8 with segmental instrumentation using local autograft bone and bone morphogenetic protein. Preoperative evaluation revealed no additional medical problems. General anesthesia was induced after sedation with midazolam (2 mg) using propofol (200 mg), lidocaine (40 mg), fentanyl (100 μg), rocuronium (50 mg), and vancomycin (1 g). General endotracheal anesthesia was maintained using a propofol infusion (160–170 μg/kg/min), sufentanil infusion (0.5–0.6 μg/kg/h), desflurane (2–3 % inspired), with no additional muscle relaxant. In addition, ketamine (50 mg) and decadron (4 mg) were given during the 5-h procedure. The patient was positioned prone with the head in a neutral position head holder and the arms were placed on arm boards with the shoulders abducted at 90°, elbows at 90°, and arms slightly forward from the plane of the chest.
In addition to the usual physiological monitors, processed EEG monitoring was used and blood pressure was monitored by an intra-arterial catheter (mean BP was maintained between 75 and 90 mmHg). Esophageal temperature was maintained between 35.9 and 37.2 °C. Intraoperative neurophysiological monitoring was conducted using SSEPs (median nerve and posterior tibial nerve), transcranial elicited MEPs recorded from the abductor pollicis brevis (APB) and abductor hallicus (AH) muscles, and free-run electromyography from the APB and T4–8 intercostal muscles. Pedicle screw testing was conducted and all responses were elicited at stimulation intensities greater than 20 mA.
The surgical procedure proceeded uneventfully. Pedicle screw placement was difficult owing to the narrow nature of the pedicles. Intraoperative CT scanning and computer-assisted navigation were used to enhance visible landmarks. Decortication of the spinous processes and placement of segmental instrumentation were accomplished and the wound was closed. The intraoperative monitoring was uneventful with no apparent changes due to surgical events. However, the amplitude of the cortical SSEPs from the left arm and the MEPs recorded from the left APB markedly decreased during the procedure. The arm position was adjusted and the blood pressure was raised resulting in resolution of the changes. The tracings are shown in Fig. 34.2. On awakening, the patient had no neurological changes and was discharged on postoperative day 3 with improved back symptoms and pain management. Her postoperative visits demonstrated continued improvement.
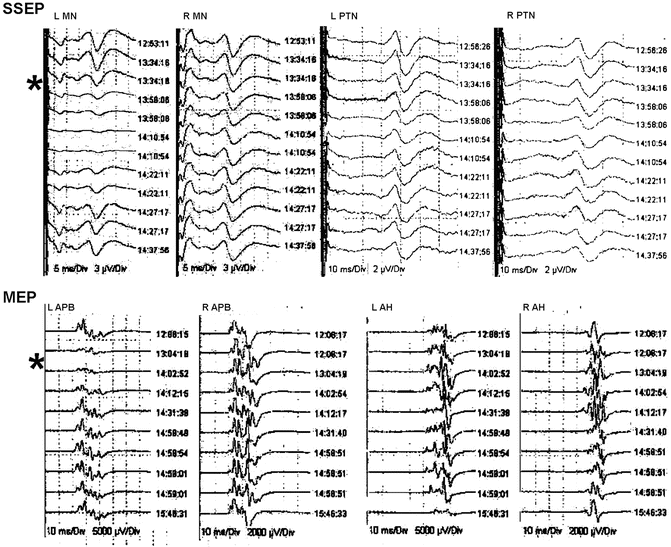
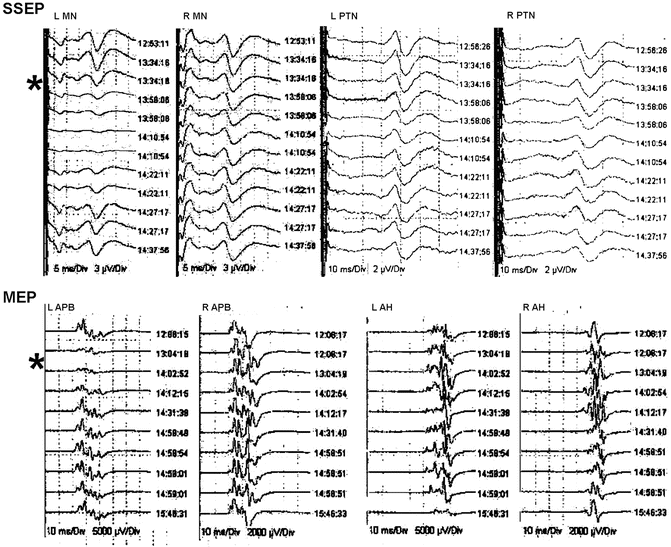
Fig. 34.2
Selected tracings during the surgery in Case 1. Cortical SSEP tracings are shown at various times from the left (L) and right (R) median (MN) and posterior tibial nerves (PTN). MEP tracings are shown at the bottom as recorded from the left and right abductor pollicis brevis (APB) and abductor hallicus (AH). The asterisk marks the onset of the loss of the SSEPs and MEPs recorded from the left arm
Discussion
For this case, the response changes can be assessed as the result of the four etiological categories: Surgical, anesthetic, technical, anatomic/positioning, and physiological. A surgical etiology was thought to be unlikely in this case primarily because the operative site was well below the neural tracts involved in the loss. When the surgeon was informed about the loss, they could not visualize how their procedure could account for this change.
Anesthetic effects would be an unlikely cause of the changes that were observed in this case, where two modalities in only one extremity were affected. In general, anesthetic effects would most likely be bilateral and global (i.e., affecting all four extremities). On the other hand, the effects of anesthesia could result in a differential effect on modalities that are most sensitive to anesthetic agents (e.g., MEPs) as well as possibly a greater effect on lower extremity responses than upper extremity responses. Finally, if the amplitude of the responses is diminished and is near the background noise level, a greater anesthetic effect might be expected on very low amplitude responses as compared to larger amplitude, more robust responses.
Finally, if the arm that lost the responses had some sort of regional anesthetic effect, then anesthesia could have accounted for the loss. Aside from a regional blockade (such as brachial plexus block or Bier block), this would require that the anesthetic agent be selectively trapped in the arm and be of the kind to interfere with axonal conduction of the SSEPs and MEPs or generation of the MEPs. Because most intravenous anesthetic agents interfere with synaptic transmission, the only agent that might be involved would be muscle relaxants. However, this would not account for the SSEP loss. If, however, a local anesthetic (e.g., lidocaine) had been injected and was trapped (e.g., a tight blood pressure cuff), then anesthetic agents could potentially be involved.
A review of the anesthetic record and discussion with the anesthesiologist did not reveal any potential etiologies for the loss. A review of technical causes for the changes was conducted. Nothing in the programming of the machine used for acquiring the IOM modalities could account for the changes. The stimulators and recording amplifiers appeared to function properly. With respect to anatomy and positioning issues, these were thought to possibly be a cause. A cerebral stroke was thought to be unlikely since that would likely have altered at least one of the other extremities. The common nerve roots involved include C8 and T1 (median nerve C6–T1, APB C8–T1) and the patient reportedly had a bulging nucleus pulposus in the cervical spine. For this to have caused the problem, there would likely to have been adverse neck positioning. This late in the case, it was thought to be unlikely since there was no evidence of improper positioning initially and the neck position had not changed. The most likely anatomic/positioning issue was pressure or traction on the brachial plexus from unfavorable arm position. The arm was examined and it appeared to be in an acceptable position. It had not been moved by the surgeons leaning on it nor had it been moved by radiology equipment, but a combination of positioning with the factors noted below was considered possible.
Examination of the forearm revealed a swollen tense arm that appeared to be caused by the infiltration of the intravenous line on that side and not due to a hematoma at the arterial line site. Temporary placement of the pulse oximeter on the fingers of that hand revealed a very poor tracing. In retrospect, the anesthesiologist noted some problems with the arterial line, which had been placed in the radial artery of that arm. Specific examination of the ground, stimulation and recording electrodes revealed marked swelling at the site of the SSEP median nerve stimulation and MEP recording needles. During SSEP stimulation, the hand motion was markedly different than it had been before the swelling. In addition, the blood pressure cuff appeared tighter than usual, perhaps due to the arm swelling.
The arm swelling could have both mechanical and physiological effects contributing to the signal loss. With respect to the SSEP stimulation electrodes, the swelling could have moved the needles away from the median nerve, decreasing the effectiveness of the stimulation and producing local ischemia in the hand impairing the recording of the MEP. Visual inspection of both sets of needles suggested that this might have been the case. With respect to the perfusion of the arm and physiological effects, the tense arm was suggestive of a developing compartment syndrome with resulting ischemia of the muscles and nerves involved in creation of the SSEPs and MEPs. In addition, the tense blood pressure cuff could also have been contributing to ischemia and the possibility of a vascular abnormality resulting in or from the DVT in that arm. Finally, the arm was cold from the infiltration of unwarmed fluids and this may also have contributed to the changes in the monitoring responses. Other physiological changes were also considered, but central changes such as relative hypotension, hypocarbia, and whole body hypothermia were thought to be unlikely since only one extremity was affected and the anesthesia monitors did not reveal these abnormalities.
In response to the change, the arm was repositioned to insure minimal problems at the brachial plexus and elbow. The mean BP was elevated by 10 mmHg. The noninvasive blood pressure cuff was moved to the other arm and the intravenous fluids in that arm were stopped. Warm pads were used to help with the arm temperature and absorption of the infiltrated fluids. The arm could not be elevated since this would increase the stress of the brachial plexus at the shoulder. The pulse in the arm, as assessed by the arterial line and pulse oximeter was observed to insure that a compartment syndrome requiring fasciotomy was not needed. The change in the evoked potentials gradually resolved and the surgery was completed uneventfully. Fortunately, the loss of monitoring capability in the arm did not reduce the monitoring signals most needed for the surgical procedure. By the conclusion of the procedure, the swelling had markedly improved and no new neurological abnormality in the involved arm could be identified.
Case 2
A 35-year-old woman with posttraumatic/postinfectious kyphosis resulting in fusion of the T7 and T8 vertebral bodies presented with severe thoracic kyphosis measuring 137°. This patient was treated nonoperatively for several years, including physical therapy, medications, and alternative treatments without improvement. She endorsed worsening deformity with the inability to maintain forward gaze. After a complete medical evaluation, including preoperative evaluation with endocrine and anesthesia, a posterior PVCR of T7, and posterior instrumentation from T2–L2 was planned (Fig. 34.3).
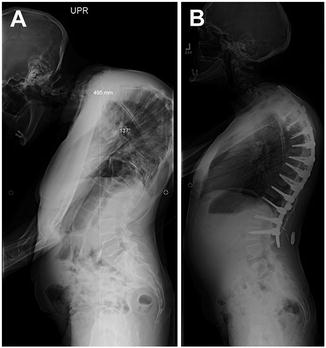
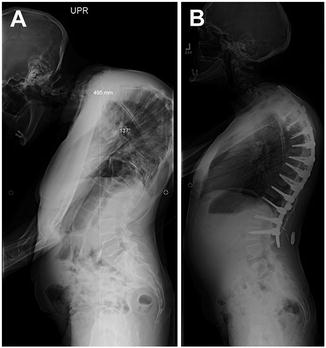
Fig. 34.3
Preoperative lateral radiograph of Case 2 showing the magnitude of thoracic deformity before (a) and after (b) surgery
General anesthesia was induced with propofol and maintained with total intravenous anesthesia. No volatile anesthetic was used at any time. Intravenous infusion of propofol, sufentanil, ketamine, and lidocaine were titrated for the remainder of the case. The patient was positioned prone with the head in a neutral position head holder and the arms were placed on arm boards with the shoulders abducted at 90°, elbows at 90°, and arms slightly forward from the plane of the chest.
In addition to the standard physiological monitors, processed EEG monitoring was used as well as an intra-arterial catheter to monitor blood pressure continuously. The mean arterial pressure was maintained between 85 and 110 mmHg for the case with intermittent use of vasoactive medications. Esophageal temperature was maintained between 35.9 and 37.2 °C. Intraoperative neurophysiological monitoring was conducted using SSEPs (median nerve and posterior tibial nerve), transcranial elicited MEPs recorded from the APB, tibialis anterior (TA) and abductor hallicus (AH) muscles, and free running electromyography from the bilateral rectus abdominus and iliopsoas muscles.
The surgical procedure proceeded uneventfully. During the exposure portion of the case, it was noted that the left lower extremity cortical SSEP signal amplitude attenuated to greater than 50 % of baseline values (Fig. 34.4) and the MEPs were maintained (Fig. 34.5). This was not observed in any other cortical signals for the remaining extremities. Importantly, the subcortical SSEP signals for the left lower extremity remained consistent with baseline values, thus an alert was not called. The left lower extremity cortical SSEP signals fluctuated above and below the 50 % threshold for the remainder of the procedure.
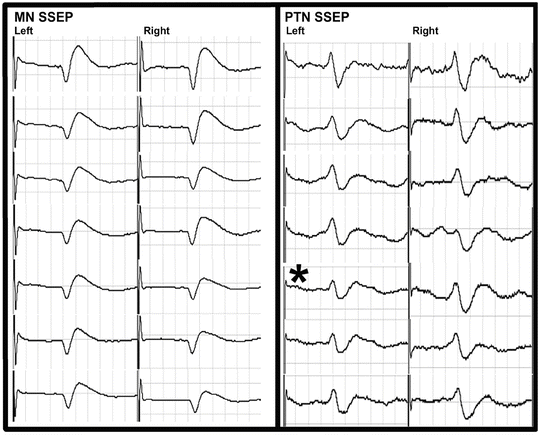
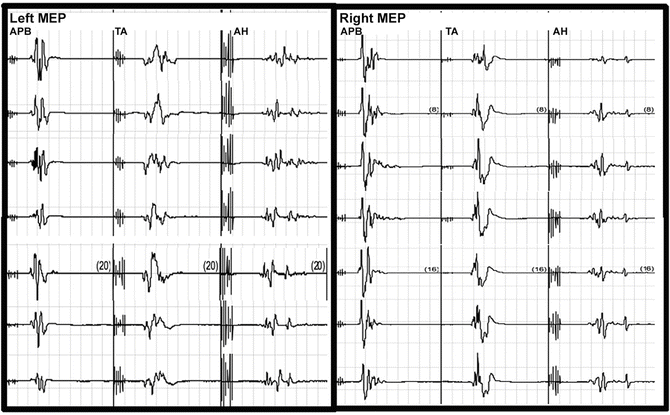
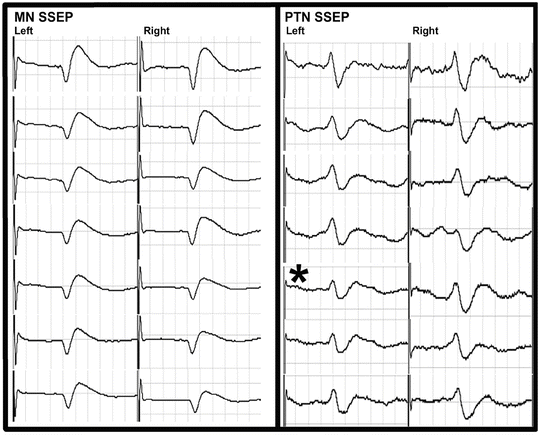
Fig. 34.4
Cortical SSEP tracings are shown at various times from the left (L) and right (R) median (MN) and posterior tibial nerves (PTN ) for Case 2. The asterisk marks the onset of the loss of the SSEPs and MEPs recorded from the left arm
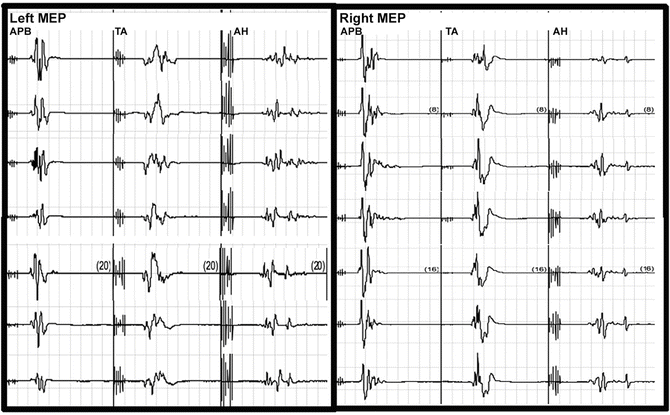
Fig. 34.5
Selected MEP tracings for Case 2 from the left and right abductor pollicis brevis (APB), tibialis anterior (TA), and abductor hallicus (AH). The tracings at the top were from the baseline and the bottom tracings were recorded at closing
Pedicle screw placement was difficult due to the narrow nature of the pedicles. Intraoperative CT scanning and computer-assisted navigation were used to enhance visible landmarks. No IOM perturbations were observed during this portion of the surgical procedure. Decortication of the spinous processes and a Smith-Peterson osteotomy (SPO) were then performed. During this segment of the procedure, acute blood loss and anemia prompted transfusion of two units of packed red blood cells. A decline in upper extremity amplitude was seen, but not to alert levels (>50 % decrease in amplitude). At this time, the surgeons requested a 10-mg dose of dexamethasone. The amplitude decrease resolved; no acute lower extremity amplitude changes or loss of MEPs were seen. Lastly, placement of segmental instrumentation and deformity correction was accomplished. During the osteotomy and spinal correction, MEPs were obtained frequently to ensure that vascular compromise and overcorrection was avoided. No further IOM perturbations were observed during this portion of the procedure. The wound was closed shortly thereafter and no new neurological deficits were noted postoperatively.
Discussion
The change in SSEP amplitudes is better visualized when the amplitude values are plotted from the technical log of the case (Fig. 34.6). Looking at this graph, it is apparent that the amplitude of the upper extremity median SSEP responses (MN) increased their amplitude following baseline acquisition, perhaps due to an influence of residual anesthesia induction drugs at baseline that resolved during further recording. This “baseline drift” reduced the sensitivity of the monitoring for these tracings such that the values of the MN amplitudes did not reach alert thresholds during the SPO when the acute blood loss occurred. As such, monitoring should consider acute changes such as those seen during the osteotomy (C to D) as a basis for concern. For example, if the baseline had been reset to the higher values at the time of x rays prior to the surgery, the amplitudes would have fallen below the alert threshold of 50 % (i.e., the amplitudes would have fallen to 45–44 % [left, right] of the new baseline). Fortunately, correction of the anemia and volume loss corrected the likely cause of the amplitude decline. Because MEPs are more sensitive to spinal cord ischemia, it was good monitoring strategy to frequently follow the MEP values during this time to insure that the transfusion and physiological management were optimal.
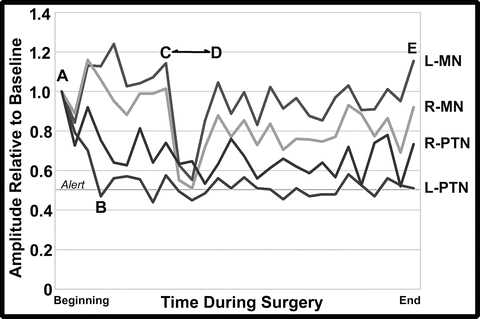
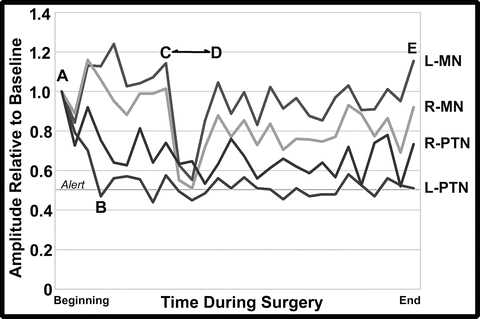
Fig. 34.6
Graph of selected tracings of the cortical SSEP amplitudes from the left (L) and right (R) median (MN) and posterior tibial nerves (PTN) as taken from the technical log of the case. Amplitudes are plotted as a fraction of the baseline values at the beginning of the monitoring (A) through closing (E, end). The amplitude of the left PTN response fell below the 50 % threshold alert criteria value at B. The amplitude of the left and right MN responses fell during the Smith-Peterson osteotomy (C to D) but did not reach the 50 % threshold alert criteria value
In contrast to the responses obtained from the upper extremity, the lower extremity amplitudes declined after the baseline recordings. Although not clear why, it was felt likely that a temperature decline in the lower extremity during positioning and skin preparation may have contributed to that change. A drop in esophageal temperature of 1.4 °C and a 6–7 % increase in latency were consistent with the reduced conduction velocity in the legs from a temperature decline. If the PTN baseline amplitude values had been reset to the amplitudes at the time of x rays prior to the surgery (i.e., 47–74 % [left, right] of the initial values), the amplitude values would have not have declined to less than 70 % of the new baselines. In this case, the team was reassured by the slow steady decline in amplitudes that preceded the surgical intervention and with the fact that MEPs remained present.
Such baseline drift has been seen in previous cases. The term “anesthetic fade” has been used for the increase in stimulation voltage needed to record MEPs as the surgery progresses [43]. In this case, declines and increases in SSEP amplitudes were both seen. Although an anesthetic-related factor may have been associated with the amplitude increase as noted above, it is unlikely that anesthesia contributed to the lower extremity SSEP amplitude decline since a global decrease would have been expected. As such, “anesthetic fade” might perhaps be better considered as “baseline drift” since multiple causes might contribute. As noted, tracking baseline drift so as to reset alert criteria and watching for acute amplitude declines are prudent practices to help optimize monitoring.
Conclusions
The use of intraoperative monitoring has become common place in the correction of spinal deformity. The initial demonstration of improved outcome was in the correction of scoliosis. The value in reducing preventable paralysis with scoliosis was shown by the Scoliosis Research Society (SRS) and European Spinal Deformities Society. They pooled the results of 173 surgeons who performed correction of spinal deformity in 51,263 cases (scoliosis, kyphosis, fractures, and spondylolisthesis) [44]. The overall incidence of neurological injury with SSEP monitoring was 0.55 % (1 in 182 cases), well below the 0.7–4.0 % expected based on historical experience. In 1992, the SRS published a position paper that concluded that “neurophysiological monitoring can assist in the early detection of complications and possibly prevent post-operative morbidity in patients undergoing operations on the spine” [44]. This was echoed in the British literature, saying “it is standard practice to conduct some form of monitoring when performing any spinal operation that is associated with a high risk of neurological injury” [45]. This made the utilization of monitoring a virtual standard of care during axial skeletal and spinal cord procedures [46].
Recently the American Academy of Neurology with the American Clinical Neurophysiology Society published an evidence-based guideline update on spine monitoring with SSEPs and MEPs [47, 48]. They identified class I and class 2 evidence which showed that the detection of adverse IOM changes during monitoring allowed significant reduction in the occurrence of paraparesis, paraplegia, and quadriplegia. All patients with new-onset neurological changes had changes in IOM responses. This finding has been echoed by multiple studies, and the cost-effectiveness in scoliosis has been demonstrated [44, 46, 49–60].

Full access? Get Clinical Tree
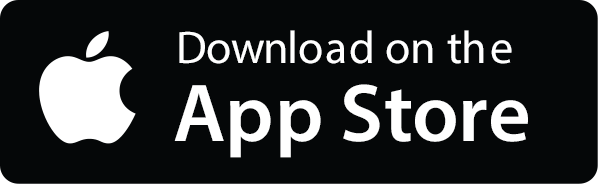
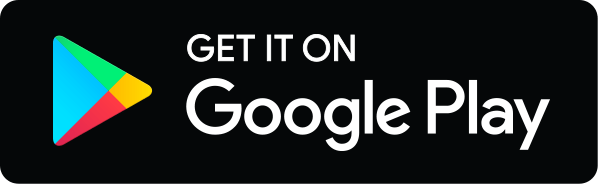