The motor unit is the functional contractile unit and is composed of a single myelinated α motor neuron and all muscle fibers that receive innervation from this single neuron. Motor units vary in size. A large motor nerve innervates more muscle fibers than a smaller motor nerve does. In general, small motor units innervate the “red slow” muscle fibers, whereas large motor units innervate the “white or pale fast” muscle fibers. The slow muscle fibers appear red as a result of high contents of myoglobin, mitochondria, and capillaries compared with white muscle fibers. Unlike the white muscle fibers, the red fibers are resistant to fatigue.6 Muscles contain a varying mixture of motor units depending on their function.
The Neuromuscular Junction
The neuromuscular junction (NMJ) or the endplate is a highly specialized synapse at which presynaptic motor nerve endings meet the postsynaptic membranes of skeletal muscles (motor endplates) (Fig. 11-2). The formation, differentiation, and function of the NMJ requires a proper interaction (cross-talk) between the nerve terminal and muscle cell (for a review, see Naguib et al.1). Failure of this cross-talk will result in a wide spectrum of neuromuscular disorders. It should be noted that in early postnatal period, muscle fibers receive multiple innervation. It has been shown in mice that on the second postnatal day, approximately 75% of muscle fibers are multiply innervated (>95% by two axons).7 However, the transition from multiple to single innervation at the NMJ occurs within few days.7 The motor nerve ending branches to form a complex of nerve terminals that invaginate into the skeletal muscle fiber but lie outside the sarcolemma. As each motor neuron approaches its target muscle fiber, it loses its myelin sheath and makes a contact with a single muscle fiber to form an NMJ. The naked motor nerve terminal that is not in contact with the muscle fiber is capped by Schwann cells. The importance of Schwann cells for development, survival, and repair of several aspects of NMJ is reviewed elsewhere.1

The NMJ is designed to transmit electrical impulses from the nerve terminal to the skeletal muscle via the chemical transmitter, acetylcholine (ACh). Structurally, the NMJ is consisted of a three components: (a) the presynaptic (or prejunctional) nerve terminal containing synaptic vesicles (filled with ACh) and mitochondria; (b) the synaptic cleft that contains basal lamina to which acetylcholinesterase enzyme responsible for hydrolysis of free ACh is attached; and (c) the postsynaptic (or postjunctional) muscle membrane that opposes the nerve terminal, which is highly infolded and these folds are called secondary folds (or secondary postsynaptic cleft). Membrane infoldings increase the surface area of the muscle plasma membrane in the postsynaptic region. Nicotinic acetylcholine receptors (nAChRs) are concentrated at the crests of these folds (directly opposing the active zones of the presynaptic membrane in which synaptic vesicles are clustered) and voltage-gated sodium channels (VGSC) are present in the troughs of the folds.
The plasticity of neuromuscular transmission is dependent on a highly orchestrated mechanism involving (a) synthesis, storage, and release of acetylcholine from the presynaptic region at the NMJ; (b) binding of acetylcholine to nicotinic receptors on the muscle membrane (postsynaptic region) and generation of action potentials; (c) rapid hydrolysis of acetylcholine by the enzyme acetylcholinesterase, which is present in the synaptic cleft; and (d) adaptation of the muscle contractile proteins to functional demands.1 Synaptic plasticity is the “ability of individual synaptic junctions to respond [i.e., to change in strength in response] to either use or disuse.”8
Presynaptic Region
Synaptic Vesicles
Synaptic vesicles (SVs) are specialized secretory organelles (see Fig. 11-2). SVs are synthesized in the neuronal cell body in the endoplasmic reticulum and transported to the nerve terminal via the microtubule system. SVs are then loaded with ACh in the motor nerve endings. Acetylcholine is first synthesized in the cytoplasm of the nerve terminal from acetyl coenzyme A and choline in a reaction catalyzed by the soluble enzyme choline acetyltransferase. An energy-dependent “transporter” then accumulates acetylcholine within vesicles. Each vesicle appears to contain 5,000 to 10,000 molecules of acetylcholine. The acetylcholine contained in a single vesicle is often referred to as a “quantum” of transmitter. The synaptic vesicles possess a diverse set of specialized proteins that can be divided into two functional classes: proteins involved in the uptake of neurotransmitters (transport proteins) and proteins that mediate SV membrane traffic such as docking, fusion, and budding (for review, see Naguib et al.1). Ca2+ signal plays a pivotal role in the process of acetylcholine vesicles exocytosis (see Fig. 11-2). There are two pools of vesicles that differ in the probability of mobilization to the active site: a readily releasable store (active pool) and a reserve store. The active pool is aligned near the active zones.
The miniature endplate potential (MEPP) amplitude represents the depolarization of the postsynaptic membrane produced by the contents of a single vesicle. Endplate potential (EPP) results from summation of MEPP produced by ACh contents of ~50 to 300 SVs. Nearly 50% of the released acetylcholine is rapidly hydrolyzed by the acetylcholinesterase during the time of diffusion across the synaptic cleft before reaching nAChRs. The products of this hydrolysis are choline and acetate. Choline is recycled into the terminal by a high-affinity uptake system, making it available for the resynthesis of acetylcholine. The drug hemicholinium-3 inhibits the later mechanism. After exocytosis, the membrane components of the SVs are recovered by endocytosis and recycled for future use.
Synaptic Cleft
The synaptic cleft is ~20 to 50 nm wide. It separates nerve and muscle fiber plasma membranes and encompasses the synaptic basal lamina and is filled with extracellular fluid. Acetylcholinesterase enzyme is bound to the basal lamina at the cleft (Fig. 11-3).

Acetylcholinesterase ranks as one of the highest catalytic efficiencies known. The efficiency of acetylcholinesterase depends on its fast catalytic activity. It can catalyze acetylcholine hydrolysis (4,000 molecules of acetylcholine hydrolyzed per active site per second) at near diffusion-limited rates.9 Acetylcholinesterase is highly concentrated at the NMJ but present in a lower concentration throughout the length of muscle fibers.10 Acetylcholinesterase is regulated, in part, by muscle activity and by the spontaneous or nerve-evoked depolarization of the plasma membrane.11 After denervation, there is a large decrease in the density of acetylcholinesterase molecules at the NMJ. In addition to hydrolysis of acetylcholine, acetylcholinesterase has other functions such as nerve growth–promoting activities12 and modulation of nAChRs.
The Nicotinic Acetylcholine Receptor at the Neuromuscular Junction
In the adult mammalian skeletal muscle the nicotinic acetylcholine receptor (nAChR) is a pentameric complex of two α subunits in association with a single β, δ, and ε subunit (Fig. 11-4). These subunits are organized to form a transmembrane pore (a channel) as well as the extracellular binding pockets for acetylcholine and other agonists or antagonists.1 Each of the two α subunits has an acetylcholine-binding site. These sites are proteins located in pockets approximately 3.0 nm above the surface membrane at the interfaces of the α-ε and α-δ subunits.13 The fetal nAChR contains a γ subunit instead of an ε adult subunit. The mature nAChR has shorter burst duration and a higher conductance to Na+, K+, and Ca2+ than the fetal nAChR.1,14

The fetal nAChR is a low-conductance channel in contrast to the high-conductance channel of the adult nAChR (Fig. 11-5). Thus acetylcholine release causes brief activation and reduced probability of channel opening.1 Upregulation of nicotinic acetylcholine receptors (nAChRs), found in states of functional or surgical denervation, is characterized by the spreading of predominantly fetal type nAChRs. These receptors are resistant to nondepolarizing neuromuscular blockers and more sensitive to succinylcholine (SCh).15 When depolarized, the immature isoform has a prolonged open channel time that exaggerates the K+ efflux.16


Full access? Get Clinical Tree
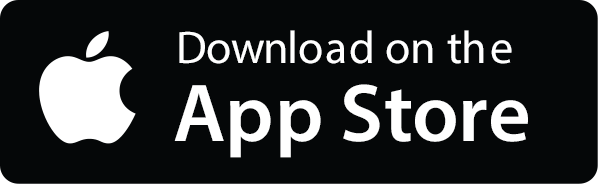
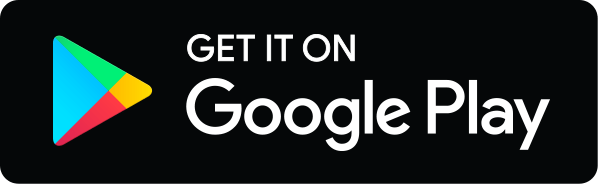