Abstract
Intensive care unit (ICU)–acquired weakness is one of the most frequent complications after ICU admission. The resulting sequelae may occur even years after discharge from the ICU; unfortunately, there are scanty data about effectively treatment that can counteract the muscle deconditioning. Neuromuscular electrical stimulation (NMES), widely used for several purposes, aims to maintain muscle trophism and function in critically ill patients. NMES may also be used as a diagnostic tool that can provide important information on the muscle state. This chapter provides the basic concepts, physiological mechanisms of NMES, and the latest evidence in the treatment of sepsis and acute respiratory failure, proposing an algorithm, and focusing on the proper execution of this physical therapy.
Keywords
Intensive care unit–acquired weakness, Motor point, Muscle strength, Neuromuscular electrical stimulation, Sepsis, Strength–duration curve
Outline
Introduction 771
Neuromuscular Electrical Stimulation: Basic Concepts and Practical Considerations 772
Fundamental Principles of Neuromuscular Electrical Stimulation 772
Neuromuscular Electrical Stimulation for Muscle Strengthening 773
Neuromuscular Electrical Stimulation for Testing Purposes 774
Neuromuscular Electrical Stimulation in the Intensive Care Unit 775
Contraindications and Adverse Effects 776
Recommendations for the Use of Neuromuscular Electrical Stimulation in the Intensive Care Unit 777
References 780
Introduction
In the last 20 years, survival after admission to the intensive care unit (ICU) has increased significantly, with approximately 75% of patients surviving to hospital discharge. Many survivors, however, have persisting physical, cognitive, and mental impairments that are the consequence of the critical illness and its treatment as well as comorbid conditions prior to the ICU admission. This post–intensive care syndrome reduces patients’ quality of life and has a great impact on families and health services. Muscle weakness acquired during the ICU stay (intensive care unit–acquired weakness, ICU-AW) is a common complication, “as common as arterial hypotension.” ICU-AW affects 25–100% of patients in the ICU. Sepsis, persistent systemic inflammation, multiorgan system failure, hyperglycemia, and possibly glucocorticoid therapy are important risk factors. ICU-AW is characterized by diffuse, symmetric weakness involving the limbs and respiratory muscles. Muscle disuse associated with muscle weakness, wasting, and deconditioning commonly afflicts patients and can seriously affect their outcome. Muscle deconditioning affects the muscle size, with a reduction in volume, but not in the number of muscle fibers. Muscle mass reduction is an acute event appearing in the first days of critical illness and is related to marked degradation of proteins and reduction in myofibrillar synthesis. Nerve conduction studies remain normal in muscle deconditioning, which constitutes an important criterion to identify different phenotypes of patients with ICU-AW, and thereby improve prognostication and guide individualized treatments. The other causes of ICU-AW are the critical illness myopathy (CIM) and critical illness polyneuropathy (CIP). Thus, muscle deconditioning can be dramatically worsened if CIP, CIM, or both complicate the clinical course. CIP manifests as a distal axonal sensorimotor polyneuropathy and CIM as a primary myopathy (not secondary to muscle denervation). CIP is diagnosed by means of electroneurography-electromyography and is characterized by a reduction in the amplitude of the compound muscle and sensory action potential with normal nerve conduction velocity. Neuropathologic investigations on nerve biopsies or postmortem show loss and degeneration of axons with the most severe involvement of distal segments. Surviving nerve fibers show a preserved myelin sheath. In CIM, major electrophysiological features are abnormal reduction in the amplitude of the compound muscle action potential and an increase in their duration, normal sensory nerve action potential, reduced muscle excitability on direct stimulation, and myopathic motor unit potentials on needle electromyography. Muscle biopsy is needed to define the structural alteration: the selective loss of thick myosin filaments and muscle fiber necrosis are typical features; contrarily, in muscle deconditioning, the necrosis is always absent.
No nutritional, antioxidant, or hormonal therapy or immunoglobulins have been shown to reduce the incidence or severity of ICU-AW. However, major advances have been made in supportive treatment. Early physical and occupational therapy in the ICU improves functional independence of patients in the ICU. In this context, neuromuscular electrical stimulation (NMES) has been proposed as a noninvasive, easily applicable technique that can be used at bedside to stimulate nerve-muscle function and to improve muscle wasting and weakness. NMES is widely used in the rehabilitation of patients who need to maintain or increase their muscle mass, strength, and function and in several diseases. Currently, it is encouragingly used to mitigate the effects of the prolonged bed rest and of the increased muscle catabolism due to the systemic inflammation. In this chapter, we will focus on sepsis and acute respiratory failure as clinical models in which NMES can be applied in the ICU.
Neuromuscular Electrical Stimulation: Basic Concepts and Practical Considerations
Electrical stimulation is widely used in clinical settings for several treatment purposes (e.g., decreasing pain, improving muscle strength, assisting human movement, reducing edema, wound healing) as well as for electrophysiological testing. Each purpose requires a specific modality of current delivery. The most common forms of electrical stimulation adopted in clinical settings are: transcutaneous electrical stimulation (TENS), NMES, functional electrical stimulation, peripheral nerve stimulation, and direct current stimulation.
When dealing with muscle strengthening, the most appropriate modality is NMES. NMES can also be used to assess the neuromuscular function, particularly to test the motor nerve excitability and muscle contractile properties and fatigability.
Fundamental Principles of Neuromuscular Electrical Stimulation
NMES is intended to generate contractions of skeletal muscles by activating the intramuscular motor nerve branches.
NMES is delivered at current intensities high enough to evoke visible or palpable muscle contractions, and hence, beyond the so-called motor threshold . This type of motor-level stimulation is in contrast with TENS, which, instead, typically acts in the domain of the sensory-level stimulation , thus with intensities below the motor threshold .
NMES can be administered with two modalities: noninvasively (transcutaneous stimulation with electrodes located on the skin surface) or invasively (percutaneous stimulation with intramuscular needle electrodes). For practicality and safety reasons, NMES is typically applied transcutaneously, with the cathode electrode placed over the belly of the target muscle. The positive electrode may be placed either on the muscle belly (close to the cathode) or more distally, namely, on the tendon or on the opposite side of the limb. Electrodes may have the same size (bipolar configuration) or different size with the anode usually larger than the cathode (monopolar configuration) ( Fig. 46.1 ).

The stimulator generates an electric field between the two surface electrodes, creating a current flow from the anode to the cathode into the tissue. The depth of the electric field and the current route will depend on the stimulation intensity and electrode configuration. As a consequence, the diverse excitable elements lying in the target tissue (e.g., motor nerve fibers, sensory nerve fibers, muscle fibers) are activated depending on the specific stimulation settings.
For normally innervated muscles, as the intensity of stimulation is progressively increased, the activation threshold of the alpha motor neuron axons belonging to the terminal motor nerve branches is reached. Motor axon depolarization will in turn cause the contraction of muscle fibers, which are inherently less excitable than motor nerve fibers. For this reason, NMES generally excites the nerve endings more effectively than the muscle, i.e., before a muscle action potential is evoked. Only in case of denervated muscles a direct muscle stimulation may occur if proper stimulus parameters are selected ( Fig. 46.2 ).

Electrode positioning may affect profoundly the selective activation of the underlying excitable structures. Indeed, the skin surface overlying a superficial muscle presents sites where the motor nerve branches are more easily excited with respect to adjacent regions. These locations have been termed motor points ( Fig. 46.1 ), which are defined as the superficial points of stimulation with the lowest motor threshold for a given electrical input, i.e., the points on the skin over the muscle belly at which the smallest amount of current is required to produce muscle contraction.
Since current density is greatest in the vicinity of the electrodes, placing the cathode on the motor point will preferentially activate the motor nerve trunk with respect to the mixed peripheral nerve containing also sensory axons ( Fig. 46.3 ). For this reason, the motor points have been advocated as the optimal sites for NMES to maximize the evoked tension, while minimizing the dose of the injected current (thus limiting spreading of current to adjacent regions) and the level of discomfort related to sensory axons stimulation. This latter aspect improves significantly the subject tolerance to NMES.

Neuromuscular Electrical Stimulation for Muscle Strengthening
NMES has received increasing attention in the past decades for its potential to serve as a muscle strength training modality, although results on actual effectiveness are conflicting. This is mainly due to methodological limitations of published studies, especially concerning the lack of detailed descriptions of the stimulation parameters, the treatment regime, and the electrode size and configuration.
Compelling evidence, however, exists that NMES can be used as a complement to voluntary resistance training for muscle strength improvement of healthy subjects as well as for the preservation of muscle mass and function in several disease conditions.
This paragraph is not intended to provide a comprehensive dissertation on the validity of specific stimulation patterns and training protocols designed for muscle reinforcement, but rather to focus on the adjustable external factors that are strictly user dependent. As a matter of fact, NMES end-users are not engaged primarily in the design of patterns of stimulation (e.g., the selection of the frequency of stimulation and duty cycle, the organization of the different phases within a protocol, the definition of the number of repetitions for each phase) because marketed electrical stimulators are provided with preprogrammed protocols. Conversely, the actual possibility of manipulation by end-users mostly concerns the stimulation amplitude settings and the electrode positioning. These operator-dependent, intertwined aspects are critical for NMES effectiveness. In analogy with voluntary contractions, the beneficial effects of NMES are obtained by an adequate intensity of contraction able to provide a proper workload (mechanical stress) and an increased local oxygen consumption (metabolic stress). The amount of the evoked muscle contractile work is therefore crucial to assure effective strength conditioning in light of the fact that muscles strengthen only in response to mechanical and metabolic stress that are sufficiently high to promote transcriptional induction and induce new myofibrillar protein synthesis. It is known that muscle mass increases after working at intensities exceeding 40% of the maximal voluntary contraction, with better results when muscles perform contractions at 60–70% of their force generating capacity. Thus, the higher the evoked muscle tension, i.e., the mechanical and metabolic stress achieved during the stimulation protocol, the greater the chance to exceed the threshold levels of activity required to induce muscle strengthening adaptation. In this view, for its ability to maximize the evoked contractile activity and increase the metabolic solicitation and energy expenditure during the stimulation regime, NMES applied via the motor point is highly recommended to heighten training intensities and improve strengthening outcomes.
Neuromuscular Electrical Stimulation for Testing Purposes
Modern clinical electromyography and nerve conduction techniques have largely replaced NMES as a method to test the function of the peripheral nerves and muscle. Nevertheless, NMES may prove to be useful when considering threshold-tracking studies of motor axons and muscle fibers and the related strength–duration curves (S–D curves), allowing the clinician to gain more insight into the nature of a neuromuscular disorder by complementing other electrophysiological techniques or in situations in which modern examination procedures are not readily available.
The stimulus amplitude (“strength”) and duration (also known as “pulse width”) are a traditional pair of electrical stimulation parameters. The S–D curves are obtained by combining these two parameters and plotting their relationship. Together, the amplitude and duration determine the pulse charge, i.e., the quantity of electricity that passes through the tissue. The stimulation of a cell depends on the charge rather than on the current. Consequently, stimulation can be achieved either by passing a large current for a short period of time or a small current for a longer period of time ( Fig. 46.2 ). However, there is a lower limit of current amplitude below which stimulation will not occur, no matter how long the duration of stimulus is. For any given excitable fiber, an S–D curve may be drawn, as shown in Fig. 46.2 , by evaluating the minimum stimulus required to activate the fiber (activation threshold). NMES, in particular, is used for motor threshold tracking. The S–D curves are obtained by placing the cathode over the motor point of the muscle and by measuring, for each pulse duration, the just-threshold pulse amplitude, i.e., the minimum strength needed to depolarize the motor nerve and evoke a visible/palpable muscle contraction. From the methodological point of view, a low stimulation frequency (1–2 Hz) is typically used, resulting in evoked muscle twitches (a twitch is the mechanical response elicited by a single pulse). The cathode over the motor point is recommended to attain a fairly consistent amount of motor axon activation from one stimulus to the next, allowing for constant amplitude of the twitch responses.
The excitability characteristics expressed by S–D curves allow to assess the degree of muscle innervation and to differentiate a normally innervated muscle from a partially or totally denervated one ( Fig. 46.2 ). Moreover, these curves can be of primary value for monitoring the progression of denervation or reinnervation after a nerve lesion.
Interestingly, the threshold measurements provide information on the membrane properties of a nerve at the point of stimulation, thereby complementing the conventional nerve conduction study that measures the conduction characteristics of the motor axon along its length. The threshold-tracking technique is therefore better suited for studying diffuse axonal properties (e.g., in metabolic neuropathies) than focal abnormalities (e.g., in segmental demyelination). Finally, if coupled with other techniques such as dynamometry or mechanomyography, NMES allows more extended possibilities for neuromuscular testing. Specifically, ramp stimulations starting from low frequencies (1–2 Hz) to tetanic stimulation (30–50 Hz) provide the so-called force–frequency relationship, which is one of the most acknowledged procedures to assess in vivo the contractile properties of skeletal muscles using electrophysiological techniques. Repetitive neuromuscular stimulation has also been extensively applied to evaluate the degree of fatigability (fatigue index) of the target muscles, allowing to retrieve specific information on peripheral fatigue by avoiding the influence of individual motivation or other central factors contributing to fatigue and/or effort intolerance.
Neuromuscular Electrical Stimulation in the Intensive Care Unit
NMES promotes the synthesis of muscle proteins and improves muscle microcirculation in diverse acute diseases [sepsis, trauma, chronic obstructive pulmonary disease (COPD), neurological diseases, etc.]. As such, NMES has been introduced in the ICU as a therapeutic option to prevent muscle weakness and, to a lesser extent, muscle wasting. NMES evokes a muscle contraction through an electrical impulse to the motor nerve via surface electrodes. Therefore, no patient cooperation is required and NMES can be usefully implemented at an early stage of acute disease in patients under continuous intravenous sedation or in those with delirium or severe reduction of consciousness. This aspect can be important to deliver treatment at an early stage of acute disease, because muscle wasting starts early and has a rapid progression, with the largest loss of mass and function occurring during the first 2 weeks of ICU stay. Systematic reviews have concluded that NMES is effective in preventing the muscle weakness in ICU. Moreover, it also has positive effects on lung function, accelerating weaning from mechanical ventilation, and possibly on limb muscle strength. Evidence is more conflicting concerning the efficacy of NMES on preventing muscle wasting, which may occur without a decrease in specific muscle strength.
Early rehabilitation has been proved to be safe and effective in critically ill patients and is widely used in patients in the ICU, but active physical therapy requires that patients be awake and collaborative. Therefore, NMES is particularly useful in patients who are not cooperative.
Sepsis
The majority of randomized controlled clinical trials have assessed the effects of NMES on heterogeneous populations of critically ill patients with percentages of patients with sepsis varying between 18% and 38%. Two small randomized controlled clinical trials including 14 and 8 patients have selectively enrolled patients with sepsis. The main outcome was muscle strength measured by means of the Medical Research Council sum score (quadriceps femoris and biceps brachii) in one trial and quadriceps muscle volume measured by means of computed tomography in the other. Both studies started NMES within 48 h after ICU admission, but only one found improvement in muscle strength in the side treated with NMES compared to the other. In an observational study in 32 patients, Stefanou et al. found an increased mobilization of endothelial progenitor cells (EPCs) in critically ill patients with sepsis treated with NMES started about 1 week after ICU admission compared to untreated patients. EPCs are precursors of endothelial cells with the potential capacity to proliferate, migrate, differentiate, or exert paracrine action, hence the results of this study can have important implications for future research. However, functional outcomes were not assessed, which leaves unsettled the impact of NMES on clinically relevant outcomes. In another observational study in a mixed population of critically ill patients that included 23 patients with sepsis, Segers et al. found that only 50% had an effective muscle contraction in at least 75% of the NMES sessions and were classified as responders. Sepsis was an independent predictor of NMES unresponsiveness together with edema and use of vasopressor. Muscle protein degradation caused by the hypercatabolic state of sepsis, mitochondrial dysfunction, and increased level of cytokines affecting muscle excitability might explain this result. According to the theory of bioenergetics failure, excitable tissues like peripheral nerves and muscles, which spend much of their energy to maintain the function, are involved early in the process ultimately leading to multiple organ dysfunctions and failure theory, thus explaining the high prevalence of ICU-acquired neuromuscular alteration. Likewise, NMES, which is based on active nerve stimulation promoting metabolic activation and energy consumption, may fail during the sepsis-related low-energy state. Gruther et al. found that NMES was beneficial in patients who were started on NMES 2 weeks after ICU admission compared to patients who were started earlier.
Explanation of these results is not easy. Although early treatment is advocated for preventive strategies to be effective, it is possible that in some subgroup of patients with sepsis treatments should be started only after a steady circulatory and metabolic state is reached. In mice, the anabolic response of septic muscle to a mechanical stimulus is suppressed in the early stage of acute illness, which is dominated by systemic inflammation, and detrimental effects due to production of proinflammatory cytokines can also be amplified by muscle activation. Since CIP and CIM are often present at an early stage of sepsis, it is also possible that NMES is not effective in the presence of an ongoing acute neuropathic or myopathic process.
In conclusion, NMES is likely to be effective in patients with sepsis, but the optimal timing for its application is undetermined. It is also unknown if ongoing CIP and CIM reduce NMES efficacy.
Acute Respiratory Failure
In patients with acute exacerbation of COPD, early studies showed that NMES can increase muscle strength and reduce the time needed for patients to transfer from bed to chair. More modern studies confirm the increase in muscle strength measured with maximal voluntary contractions, or evaluated manually after use of NMES, either alone or associated with physical rehabilitation. The distance covered during a 6-min walking test is also significantly increased in patients with COPD treated with NMES. Other authors, in a pilot study, did not find significant differences at hospital discharge in lower limb muscle strength between NMES-treated patients and controls, but those treated with NMES walked farther than controls. Dyspnea, measured with a specific scale, was reduced after NMES treatment. In a study with muscle biopsy, the use of NMES was associated with an increased number of slow muscle fibers, which is probably a consequence of transition from fast-to-slow fiber type.
In conclusion, NMES is probably effective in patients with COPD admitted to the ICU because of acute exacerbation of respiratory failure, but the optimal timing for starting NMES remains uncertain. There are no studies evaluating the efficacy of NMES in patients with acute respiratory distress syndrome.

Full access? Get Clinical Tree
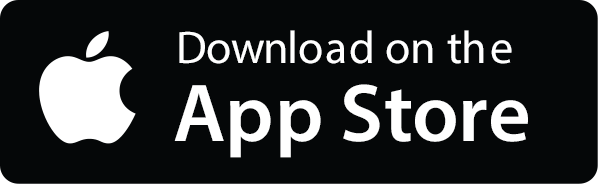
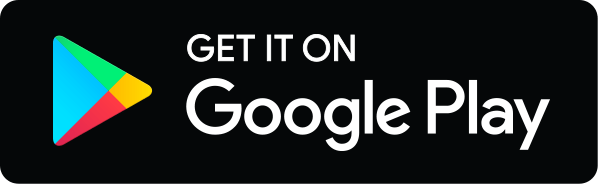