Fig. 15.1
Diagram of the postsynaptic nicotinic acetylcholine receptor at the neuromuscular junction with the five subunits. Each subunit contains four transmembrane domains labelled M1 to M4. Reproduced from Naguib et al. [7] with permission from Wolters Kluwer Health, Inc.
The receptor channel complex is roughly cylindrical in shape with a length of 11 nm and a diameter of 8.5–9.0 nm. The receptors are clustered on the crests of the junctional folds, the receptor; density in this area is 10,000–30,000/μm2. There are about 5 million of them in each junction. Two molecules of acetylcholine must bind to the α subunits to induce the conformational changes, which will allow ionic flows. The ion channels of the end-plate region are chemically sensitive and when open cannot discriminate between sodium and potassium which will flow inward and outward. At resting potential the inward movement of sodium will be favoured because sodium will move along its electrical gradient. Accumulation of intracellular sodium makes the inside of the cell less negative. If a large number of receptors open simultaneously, the inside of the cell is depolarised sufficiently to trigger the action potential. The propagation of the action potential to the whole muscle is then independent of acetylcholine receptors. It produces opening of voltage-sensitive calcium channels. Intracellular calcium increases rapidly and binds with troponin which usually inhibits actin and myosin. The immature receptor has a smaller single-channel conductance and a much longer channel open time than the mature receptor.
Prejunctional Event s
During high-frequency stimulation, under physiologic conditions, the release of acetylcholine decreases, but it is more than enough to depolarise the end plate above threshold. Small concentrations of acetylcholine increase the evoked release of acetylcholine, whereas d-tubocurarine and other nicotinic antagonists reduced the release, suggesting that acetylcholine may facilitate its own further release. The decrease in acetylcholine release during high-frequency stimulation (train-of-four (TOF), tetanus) in the presence of non-depolarising muscle relaxant (NDMR) drugs explains the progressive decrease in muscle response, i.e. fade. It is likely that this effect is mediated through presynaptic receptors. These prejunctional receptors could mediate mobilisation of the reserve store into the readily releasable store in case of high-frequency stimulation [8]. Difference in potencies of nicotinic antagonists at pre- and postjunctional receptors suggests a difference between the two types [9].
Effects of Muscle Relaxants at the Neuromuscular Junction
Depolarising Muscle Relaxants: Succinylcholine
Succinylcholine produces a depolarisation of the end-plate region which is similar to, but more persistent than, that achieved by acetylcholine. This depolarisation induces desensitisation of the nAChR, inactivation of voltage-gated Na+ channels at the neuromuscular junction and increase of the K+ permeability in the surrounding membrane. Onset of neuromuscular blockade is characterised by an excitatory state with fasciculations of muscle fibres thought to represent random repetitive neuronal firing. It could be due to the depolarisation of prejunctional receptors; the abolition of fasciculations by d-tubocurarine may represent an action at the presynaptic receptor. The neuromuscular block is characterised by a twitch response to indirect stimulation which is diminished but sustained with repetitive stimulation. It is associated with end-plate depolarisation and development of a surrounding zone of inexcitability through which a muscle action potential evoked by direct stimulation cannot propagate. During prolonged depolarisation, the muscle may gain sodium and chloride and lose significant amounts of potassium, sufficient to raise serum levels of this ion. Following continuous administration, a “phase II block” characterised by fade and post-tetanic facilitation occurs. One of the mechanisms could be an excessive activation of presynaptic nicotinic receptors, which could lead to reduced transmitter output. “Phase II block” could also be associated with a desensitisation of the end plate, which then becomes refractory to chemical stimulation. Succinylcholine depolarises immature receptors more easily, inducing a significant cation flux. Moreover, once depolarised, the immature receptor will stay open for a longer time.
Non-depolarising Muscle Relaxants (NDMRs)
NDMRs competitively bind at the same site as acetylcholine on the α subunit of the nicotinic postjunctional receptor . The binding of one nicotinic antagonist molecule to one of the two α subunits of the receptor prevents opening of the channel because both α subunits need to be bound by acetylcholine for activation of the channel. In a given situation, the proportion of receptors bound to the agonist depends on the affinity of the binding site for the agonist, on its affinity for the antagonist and on the concentration of agonist and antagonist. Some authors have suggested that NDMR could also block the open receptor because they can have access to the mouth of the open channel but cannot pass through, thus preventing further ionic movement. This type of block is non-competitive but its role is probably marginal. Only a small fraction of receptors need to bind to acetylcholine to produce depolarisation. This has been referred to as the “margin of safety” of the neuromuscular transmission. Non-depolarising neuromuscular block is not apparent until a large number of receptors are occupied by the muscle relaxant. Animal studies suggest that 75 % of receptors must be occupied by a NDMR before twitch height decreases. Blockade is usually complete in peripheral muscles when 92 % of receptors are occupied. Peripheral muscles have been found to have a smaller margin of safety than the diaphragm.
Factors Which Govern the Duration of Action
Onset
There is ample evidence that potent NDMRs have slower onset times than less potent drugs with similar physicochemical properties . These facts can be explained by the concept of the margin of safety. A critical number of receptors at the neuromuscular junction must be occupied before appearance of neuromuscular block, and at least 90 % of the receptors must be occupied before block is complete at the adductor pollicis . When the drug reaches the synaptic cleft, most molecules will bind to receptors which are present with a high density. As the concentration of free drug decreases, more molecules are driven in, and the process will continue until the concentrations of free drug within and outside the synaptic cleft are equal. This process is known as buffered diffusio n [10]. When a potent drug is administered, fewer molecules are given than in a case of a less potent drug, and the onset will be slower compared to onset of weak potency NDMR [11]. This phenomenon is probably what contributes to the slower onset time for cisatracurium than atracurium. Based on theoretical calculations and experimental data, the ED95 to produce a short onset of action might be >0.3–0.5 mg/kg (Fig. 15.2) [9]. However, for very short-acting drugs, the ideal ED95 might be greater (0.5–1.0 mg/kg) because rapid metabolism destroys some of the muscle relaxant given before it reaches the neuromuscular junction [12]. This phenomenon can explain the relatively slow onset time for mivacurium.
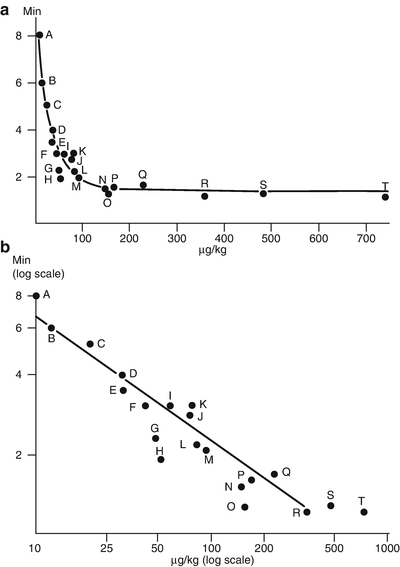
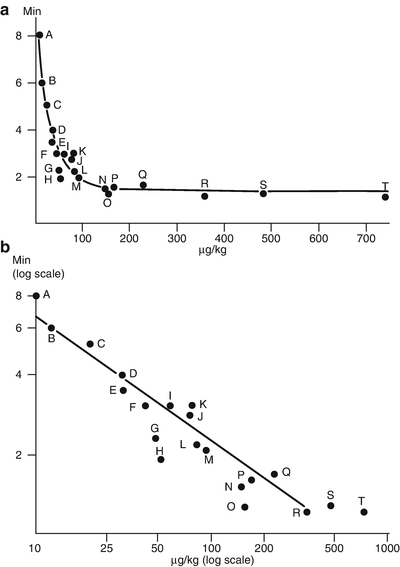
Fig. 15.2
The relationship between effective dose and onset time for 20 chemically related aminosteroidal neuromuscular blocking drugs. The effective dose (abscissa) is the mean dose to produce 50 % twitch depression of the tibialis anterior. Reproduced from Bowman et al. [9] with permission from Wolters Kluwer Health, Inc.
Plasma concentrations have only modest influence on onset time. Arterial plasma concentrations peak 25–35 s after administration thus before onset of neuromuscular block. This paradox can be explained by assuming that the site of action, the neuromuscular junction, is represented by the effect compartment in which the concentration of the NMBA is directly related to the magnitude of neuromuscular blockade [13]. As usual the model will include a rate constant for transfer into the effect compartment and another rate constant for transfer out of this compartment. The obtained ke0 or more appropriately the T1/2ke0 is related to the time required to fill or empty the effect compartment for a constant plasma concentration. The ke0 is similar for most intermediate-duration action NMBAs and corresponds approximately to neuromuscular junction blood flow divided by neuromuscular junction/plasma partition coefficient . Whatever muscle relaxant, the limiting factor appears to be the time required for the drug to reach the neuromuscular junction which in turn depends on cardiac output, the distance of the muscle from the central circulation, and muscle blood flow. Therefore in most cases, the onset time will be dependent on blood flow to muscle. It has been demonstrated that in dogs time to maximum blockade was decreased when blood flow increased, whereas recovery was not affected by blood flow [14]. Under normal circumstances, muscle blood flow increases when cardiac output increases, with a direct relationship between speed of onset and cardiac output. This might explain why infants and children have a faster onset of action of NMB and elderly patients have a slower onset than younger individuals. It has been demonstrated that vecuronium-induced onset of NMB was shorter with etomidate than with either thiopental or propofol and onset time was longer if hypotension was present [15].
It is obvious that the intensity of maximum blockade is affected directly by the administered dose. However when the dose increases in the sub-paralysing range, that is, when maximum blockade is between 0 and 100 %, time to reach maximum effect is dose independent. This is because the time to peak concentration at the effect compartment is independent of the dose. When the administered dose is sufficient to obtain complete disappearance of the neuromuscular response, time to maximum blockade becomes dose dependent. For many years, it was believed that shorter-acting NMBA would have a faster onset time than long-acting NMBA. Many studies have shown that atracurium or vecuronium onset times were comparable to that of pancuronium. The lack of effect of elimination of these drugs on onset time is explained by the fact that these NMBAs are eliminated too slowly to see any difference.
Duration of Action
Although it is commonly believed that the rate of decline of NMBA plasma concentrations during recovery from neuromuscular blockade determines the duration of action and the rate of recovery, further explanations are needed. It has been suggested that muscle blood flow is, to a certain extent, a limiting factor for the termination of action. For long-acting NMBA, the dominant effect for the recovery from neuromuscular blockade is the rate of decrease of plasma concentration because there is a pseudoequilibrium between concentrations at the neuromuscular junction and the plasma. Therefore changing blood flow will not affect the duration of action. For intermediate-duration action NMBA, after a single bolus dose, plasma concentrations decrease at a rate that differs slightly from the equilibrium half-life with muscle. It can induce a significant concentration gradient between neuromuscular junction and plasma during recovery, but provided that recovery rate is constant, the ratio of concentrations between the neuromuscular junction and plasma will remain relatively constant.
The most important issue is that the rate of decline of plasma concentration during recovery is not always related to the NMBA terminal half-life because after initial administration, plasma concentrations will decrease because of redistribution. It is only when redistribution will be complete that the decrease in plasma concentrations will be dependent of the terminal half-life and will decrease more slowly. For long-acting NMBA such as pancuronium, the recovery time will take place during the terminal half-life. In this situation, the duration of action will be dependent on the rate of decrease of plasma concentrations. It is different for intermediate-duration action NMBA. The terminal half-life of atracurium is around 20 min, whereas the elimination half-lives of both vecuronium and atracurium are between 60 and 120 min. Although such differences can be observed, the duration of action and recovery from neuromuscular block of these three drugs is very similar. These apparent discrepancies can be explained by the fact that the distribution phase is the most important factor and extends for a much longer period than for long-acting NMBA (Fig. 15.3) [16]. If their duration of action and recovery rates are almost identical, it is because plasma concentrations decrease to levels compatible with recovery during the redistribution phase.
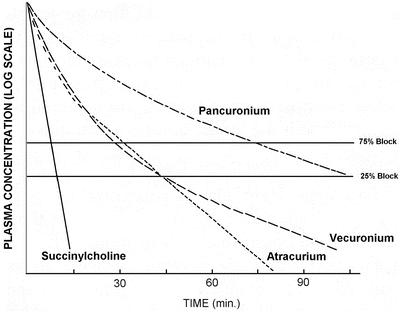
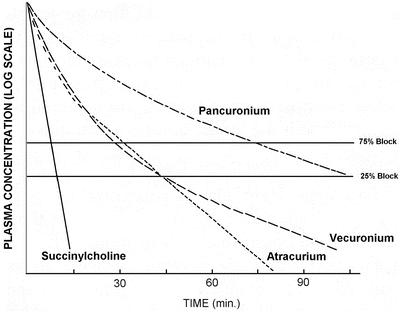
Fig. 15.3
Plasma concentrations after equipotent doses of pancuronium, vecuronium, atracurium and succinylcholine as a function of time. Reproduced from Donati F [16] with permission from Elsevier
Individual Drugs
Succinylcholine (Fig. 15.4)
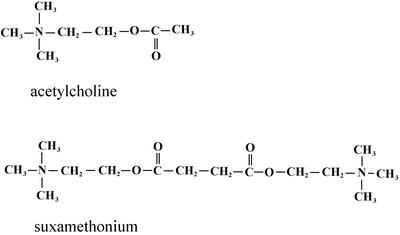
Fig. 15.4
Chemical structures of acetylcholine and succinylcholine
Pharmacolog y
Succinylcholine is the only available NMBA with a rapid onset of effect and ultrashort duration of action. Succinylcholine is rapidly hydrolysed by plasma cholinesterase (Table 15.1) to choline and succinylmonocholine. Its estimated plasma half-life is about 2 min and a return of neuromuscular activity occurs quickly. The mean doses producing 50 % blockade (ED50) and 95 % blockade (ED95) at the adductor pollicis are close to 0.3 and 0.5–0.6 mg/kg, respectively, during oxygen–opioid anaesthesia. Following 0.5 mg/kg succinylcholine, onset time is within 60–90 s at the adductor pollicis and within 60 s at more central muscles such as the laryngeal adductor muscles [17] and the diaphragm. A dose of 1.0 mg/kg succinylcholine produces complete neuromuscular block in about 60 s. Time to 90 % recovery at the adductor pollicis, following 1 mg/kg succinylcholine, is within 6–12 min. When a small dose of NDMR is given before succinylcholine, the onset time is increased by about 30 %, and with the exception of pancuronium, which inhibits cholinesterase, the duration of action is decreased by 30–50 %. The dose of succinylcholine must be increased by 50–100 % to achieve comparable paralysis. Because little or no butyrylcholinesterase is present at the neuromuscular junction, the neuromuscular blockade is terminated by its diffusion away from the neuromuscular junction.
Table 15.1
Metabolism and elimination of neuromuscular blocking agents
Muscle relaxant | Metabolism | Excretion | |
---|---|---|---|
Kidney (%) | Liver (%) | ||
Succinylcholine | Plasma cholinesterases (90 %) | 1–2 | – |
Atracurium | Hofmann elimination + Ester hydrolysis (60–90 %) | 10–40 | |
Cisatracurium | Hofmann elimination (80 %) | 10–15 | – |
Pancuronium | Liver (10–20 %) | 70 | 30 |
Pipecuronium | Liver (10 %) | 70 | 20 |
Rocuronium | Liver (10 %) | 30 | 70 |
Vecuronium | Liver (40 %) | 20–30 | 70–80 |
Butyrylcholinesterase is produced by the liver and is present in the plasma. Butyrylcholinesterase activity may be decreased during pregnancy, hepatic disease, burns, sepsis, malnutrition and treatment by cyclophosphamide, cytotoxic drugs or ecothiopate eye drops. The duration of action of succinylcholine may be increased by no more than 25–30 %. In clinical practice, esmolol can induce a slight and transient prolongation of succinylcholine-induced neuromuscular block.
A small proportion of patients have a genetically determined inability to metabolise succinylcholine. In white populations, 95–97 % of the population will have the normal cholinesterase genotype. The duration of action is slightly increased (10–20 min) in heterozygous patients. Neuromuscular block may be very prolonged (45–360 min) in patients homozygous for either the atypical or the silent gene [18]. Dibucaine is more active in inhibiting normal butyrylcholinesterase (80 %) than the abnormal variant (20 %). In clinical practice, the dibucaine number is recommended to confirm the deficit and the genetic profile [19].
Prolonged administration of succinylcholine leads to a change in the nature of the block from that of a depolarising drug to one resembling that of NDMR and called “phase II block”. Appearance of fade after continuous administration of succinylcholine could be dependent on the affinity for the presynaptic α3β2 neuronal subtype nAChR when the concentrations exceed the normal clinical concentration observed after a single bolus dose [20]. Its occurrence is dependent of the dose, the duration of administration and the patient; the specific contribution of each factor remains discussed.
Neostigmine and pyridostigmine inhibit acetylcholinesterase but may also inhibit butyrylcholinesterase. When succinylcholine is given, after neostigmine administration, the neuromuscular block will be more profound and prolonged.
Side Effects
Succinylcholine can produce a number of side effects , although these are usually of minor clinical importance. The life-threatening complications, malignant hyperthermia (MH), anaphylaxis and extreme hyperkalaemia, are rare but may arise without warning.
The frequency of fasciculations is high, especially in adults. It can be prevented by giving a small dose of NDMR before succinylcholine but care must be taken to avoid partial paralysis in awake patients. The reported incidence of muscle pain varies from 1.5 to 89 %. The importance of early postoperative mobility has been reported to be a factor in determining the severity of muscle pain. A relationship between fasciculations and muscle pain has not been established although it is considered that the unsynchronised contractions of muscle fibres before onset of neuromuscular block could play a significant role. It could also explain the rise of serum creatine kinase following succinylcholine administration [21].
The administration of succinylcholine is followed by a transient increase in serum potassium concentration which is usually less than 0.5 mmol/L. The increase in K+ results from the depolarising action of the drug. It seems to be dependent of the anaesthetic technique used at induction. In children, halothane induction followed by succinylcholine produces an increase of serum potassium of 0.2–0.5 mmol/l, while the thiopental–succinylcholine sequence is associated with a small decrease of about 0.1–0.35 mmol/l. The rise usually occurs within 3–5 min after injection. Severe hyperkalaemia, producing arrhythmias or cardiac arrest, may occur in patients with burns, traumas, sepsis, severe metabolic acidosis and neurological and muscular disorders. The mechanism could be due to the extrajunctional spread of acetylcholine receptors. In burn patients, it has been suggested to avoid succinylcholine at least until several weeks to months after complete healing when the patient reverts to a normal metabolic state. In trauma patients the risk of hyperkalaemia occurs 2–7 days after the injury and can last at least 2 months after trauma. Therefore, the use of succinylcholine is contraindicated until complete healing of the muscular lesions and recovery of the patient [21].
Because of its structure similarity to acetylcholine, succinylcholine can stimulate cholinergic autonomic receptors on both sympathetic and parasympathetic ganglia and muscarinic receptors in the sinus node of the heat (Table 15.2). Therefore, succinylcholine can produce sinus bradycardia with nodal or ventricular escape beats, especially in children and infants when vagal tone is predominant. These changes in cardiac rhythm can be prevented by prior administration of atropine or glycopyrrolate. In adults bradycardia is more common after a second dose of succinylcholine. The mechanism of the bradycardia, in adults, following a second dose, is poorly understood. Succinylcholine increases catecholamine release, giving rise to tachycardia in adults. Ventricular escape beats may occur as a consequence of severe sinus bradycardia and atrioventricular nodal slowing. However a single bolus is not usually associated with any pronounced tachycardia in adults. Ventricular dysrhythmias can also be the consequence of hyperkalaemia.
Table 15.2
Clinical autonomic effects of neuromuscular blocking agents
Muscle relaxant | Autonomic ganglion | Cardiac muscarinic receptors | Histamine release |
---|---|---|---|
Succinylcholine | Stimulation | Modest stimulation | Slight |
Atracurium | 0 | 0 | Moderate |
Cisatracurium | 0 | 0 | None to slight |
Mivacurium | 0 | 0 | Moderate |
Pancuronium | 0 | Weak block | 0 |
Pipecuronium | 0 | 0 | 0 |
Rocuronium | 0 | Weak block | 0 |
Vecuronium | 0 | 0 | 0 |
Following succinylcholine, there is a mean increase in intraocular pressure of about 8 mmHg which peaks 2–4 min after administration and lasts for up to 10 min. This effect may be due to a sustained contraction of the extraocular muscles and is not reliably prevented by pretreatment with NDMR. Succinylcholine traditionally has been avoided in patients with a penetrating eye injury and the opening of the eye anterior chamber. However, there is a greater increase in intraocular pressure during laryngoscopy and intubation in lightly anaesthetised, poorly paralysed patients. When succinylcholine is contraindicated and in case of a patient with a full stomach and a penetrating eye injury, rocuronium can be used to perform a rapid sequence induction of anaesthesia.
Succinylcholine causes an increase in intragastric pressure which may be as high as 30-cm H2O and is apparently related to the severity of the fasciculations of the abdominal wall muscles. It has been observed that the pressure in the lower oesophageal sphincter increases during fasciculations by more than the intragastric pressure, and this increase in pressure may lessen the risk of regurgitation and aspiration. However in case of pregnancy, hiatus hernia or bowel obstruction incompetence of the gastro-oesophageal junction can occur during an increase in intragastric pressure of less than 15-cm H2O. The smaller increase in intragastric pressure in infants and children could be related to the less intense fasciculations in the first years of life [22].
The increase in intracranial pressure is poorly understood although it may be due to an increase in PaCO2 during fasciculations. It can be prevented by pretreatment with NDMR. Masseter muscle rigidity (MMR) is a contracture of the masseter to a degree which interferes with tracheal intubation and despite the presence of adequate concentrations of succinylcholine. Several studies have demonstrated that an increase in masseter muscle tone of up to 500 g lasting 1–2 min is a normal finding [23]. Most cases of the so-called MMR may represent simply the extreme of a spectrum of tension changes in response to succinylcholine. The incidence of MMR is within 0.5–1 %, whereas the incidence of malignant hyperthermia is about 1:12,000 in children and 1:30,000 in adults. Traditionally, MMR has been taken to herald the development of malignant hyperthermia. Occasionally, MMR may precede malignant hyperthermia. Increasingly, an expected approach to the management of MMR is being advocated with monitoring for signs of hypermetabolism (hypercapnia, metabolic acidosis, hyperthermia, tachycardia, increased oxygen consumption, hyperthermia). Some authors have concluded that anaesthesia can be continued safely in isolated cases of MMR when careful monitoring is employed. Anaesthetic vapours will be avoided if the case is continued.
There is some controversy concerning the incidence of anaphylaxis following succinylcholine. Almost all the cases of anaphylaxis have been reported in Europe or Australia. The incidence of anaphylactic reactions may be close to 0.06 %. It has been shown that, of all muscle relaxants, succinylcholine was associated with the greatest number of serious reactions. The anaphylactic mechanism is the most frequent. When the muscle relaxant cross-links with IgE, degranulation and release of histamine, neutrophil chemotactic factor and platelet-activating factor occur. The release of these mediators can induce cardiovascular collapse, bronchospasm and skin reaction. Patients with a history of anaphylactic reaction to succinylcholine may exhibit a cross-reaction, at least in vitro, with other NMBAs in approximately 60–70 %. The cross-reactivity is related to the common structural features of these drugs, all of which contain quaternary ammonium ions [24]. In case of anaphylactic reaction to succinylcholine, it is mandatory to complete investigation for cross-reactivity with the other commercially available NMBAs to identify safe alternative regimens.
Steroidal Compounds (Fig. 15.5)
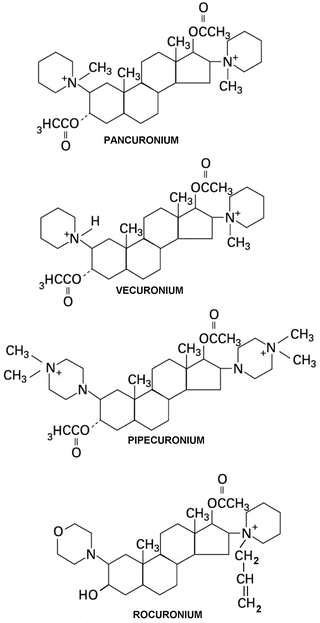
Fig. 15.5
Chemical structures of steroidal NMBAs
Vecuronium
Vecuronium is a synthetic steroid-based molecule which came from the demethylation of pancuronium. It is a monoquaternary compound. Vecuronium undergoes spontaneous deacetylation to 3- and 17-hydroxy metabolites. This process takes place principally in the liver, 40–80 % of the dose being taken up and excreted by the bile (Table 15.1). The most potent metabolite is the 3-OH derivative which is 50 % as potent as vecuronium. Vecuronium and the 3-OH derivative are eliminated through the kidneys [25]. The 17-OH and 3–17-dihydroxy metabolites retain almost no neuromuscular blocking activity.
In contrast to pancuronium , vecuronium has a relatively short duration of action which can be explained by a plasma clearance (3–5 ml/kg/min) double that of pancuronium (Table 15.3). Although the elimination half-life of vecuronium (50–80 min) [26] is larger than atracurium, the duration of action and the rate of recovery are similar. This discrepancy is explained by the fact that vecuronium has a more rapid initial distribution than atracurium. Plasma concentrations decrease rapidly, so that duration of action and recovery for vecuronium depend more on distribution than elimination. This also explains why larger doses are associated with a longer recovery index. In case of repeated dosing, accumulation may occur, especially in the presence of renal failure. The elimination half-life of vecuronium is increased from 55 to 73 min in cirrhotic patients as the result of a decrease in clearance, the volume of distribution being unchanged [27]. Similar findings were observed in patients with cholestasis [26].
Table 15.3
Pharmacokinetic parameters of muscle relaxants
Drug | Vdss (ml/kg) | Clearance (ml/min/kg) | Elimination half-life (min) | Protein bound (%) |
---|---|---|---|---|
Succinylcholine | 6–16 | 200–500 | 2–8 | 30 |
Pancuronium | 260–280 | 1.9 | 110–135 | 30 |
Pipecuronium | 340–425 | 1.6–3.4 | 100 | – |
Vecuronium | 180–250 | 3.6–5.3 | 50–53 | 30–57 |
Rocuronium | 170–210 | 3.4 | 70–80 | 25 |
Atracurium | 180–280 | 5.5–10.8 | 17–20 | 51 |
Cisatracurium | 110–200 | 4–7 | 18–27 | – |
Mivacurium | ||||
cis–trans trans–trans cis–cis | 146–588 123–338 191–346 | 26–147 18–79 2–5 | 1–5 2–8 41–200 | – – – |
The ED50 of vecuronium is 0.025–0.030 mg/kg and the ED95 about 0.04–0.05 mg/kg. The onset of action at one ED95 dose is within 5 and 6 min. It can be reduced considerably by increasing the intubating dose or by priming. A dose of 0.08–0.10 mg/kg will produce maximum block within 2–3 min (Table 15.4). As for other NDMRs, the onset of action is more rapid at the diaphragm and the laryngeal adductor muscles than at the adductor pollicis [28, 29]. Following twice the ED95, the clinical duration of action is about 25–40 min at the adductor pollicis and minutes at the laryngeal muscles. The spontaneous recovery index is close to 12 min. Little accumulation is observed with vecuronium. Recovery from neuromuscular block can be accelerated by neostigmine or edrophonium once the fourth response at the TOF is present. The dose–response of vecuronium is similar in elderly and young adults. Following a bolus of 0.07 mg/kg, the clinical duration of action is shorter in children than in adults and infants.
Table 15.4
ED95 and clinical effects of NDMR at clinical doses (2 × ED95 at the adductor pollicis)
ED95 (mg/kg) | Intubating dose (mg/kg) | Onset time (min) | Total duration of action (min) | Recovery index (min) | |
---|---|---|---|---|---|
Atracurium | 0.230 | 0.5 | 3–4 | 50–60 | 11–12 |
Cisatracurium | 0.048 | 0.150 | 4–5 | 70–80 | 12–15 |
Mivacurium | 0.080 | 0.200 | 3 | 30 | 6–7 |
Pancuronium | 0.050 | 0.070–0.100 | 3.5–4 | 120 | 30–40 |
Pipecuronium | 0.045 | 0.080 | 3.5–4 | 120 | 30–40 |
Rocuronium | 0.300 | 0.600 | 1.5 | 60–70 | 14 |
Vecuronium | 0.040 | 0.08–0.1 | 3 | 50–60 | 12 |
Even at high doses (8 times the ED95), vecuronium does not have significant cardiovascular effects. It does not exhibit action at the autonomic ganglia or sympathetic nervous system exists and does not induce histamine release (Table 15.2). The intermediate duration of action following vecuronium administration makes it useful for most surgical procedures including day-care surgery. An initial dose of 0.07–0.10 mg/kg provides good intubating conditions within 2–3 min. During continuous infusion a dose of 1–2 μg/kg/min is necessary to maintain a 90 % block at the adductor pollicis. Large doses (0.2–0.4 mg/kg) have been used to provide rapid intubating conditions in place of succinylcholine. However the duration of action will be 90–120 min.
Rocuronium
Rocuronium’s main characteristic is a short onset of action when compared with vecuronium, atracurium or cisatracurium.
In humans 33 % of the dose is eliminated unchanged by the kidneys. Metabolites are below the detection limit. There are no human data on biliary excretion; however in cats 50 % of the administered dose of rocuronium is recovered from the bile and only 9 % from the urine within 6 h after administration, indicating that elimination is mainly determined by hepatobiliary excretion [30] (Table 15.1). The pharmacokinetic parameters of rocuronium and vecuronium are of the same order of magnitude; the volume of the central compartment and the volume of distribution at steady state (Vdss) appear to be smaller than those for vecuronium in normal patients (Table 15.3). Pharmacokinetics of rocuronium have been reported in patients with or without renal failure after a single dose of 0.6 mg/kg. There were no significant differences between the two groups with respect to the neuromuscular parameters. The main difference in kinetic parameters was a decreased plasma clearance and an increased mean residence time in patients with renal failure. The duration of action is increased in patients with hepatic dysfunction (114 vs 47 min); this could be due to the larger volume of distribution when compared with healthy patients [31].
The potency of rocuronium is about one-seventh the potency of vecuronium . The ED95 is 0.300 mg/kg at the adductor pollicis. The low potency may explain its rapid onset of action when compared with other NDMRs [19, 20]. After a dose of 0.6 mg/kg, the onset of action is close to 90 s at the adductor pollicis [21] (Table 15.4). A relationship between potency and onset of action has been demonstrated by Bowman. When a less potent NDMR is administered, more molecules are administered than in the case of a more potent NDMR, and the receptors at the neuromuscular junction will be occupied more rapidly. Following equipotent doses, the duration of action of rocuronium is similar to that of atracurium and vecuronium. Minimal cumulative effects have been observed with up to three repeated doses under halogenated anaesthesia. Rocuronium has minimal vagolytic properties; its administration is associated with only small changes in haemodynamic variables (7 % increase in heart rate and 11 % increase in the cardiac index). Like other steroidal NDMRs, it does not cause histamine release (Table 15.2). The main advantage of rocuronium is its short onset of action which provides good intubating conditions 60–90 s after a bolus of 0.6 mg/kg [32]. Rocuronium can be used when rapid tracheal intubation is indicated and succinylcholine is contraindicated . However, it must be remembered that it is an intermediate-duration action NDMR with an onset of action less than 90 s at the laryngeal adductor muscles [33].
Long-Acting Steroidal Compounds
Long-acting NDMRs commercially available include, depending on the country, pancuronium and pipecuronium. Because of their clinical duration of action (time from injection until 25 % recovery of twitch height), the incidence of residual block at the end of the procedure can be important (30–40 %). These compounds are specially designed for long surgical procedures or when mechanical ventilation is necessary in the postoperative period.
Pancuronium
Pancuronium is a synthetic bisquaternary aminosteroid compound. Pancuronium is metabolised mainly by acetylation in the liver to 3- and 17-OH derivatives which are excreted in the urine. The 3-OH metabolite which is 40–50 % as potent as pancuronium is the only one detected in humans. The 17-OH metabolite has about one-fiftieth the potency of pancuronium. Pancuronium is mainly eliminated through the kidney (40–70 % of the initial dose) (Table 15.1).
In normal patients volume of distribution is about 0.3 L/kg, corresponding to the extracellular fluid volume. Total plasma clearance is close to 2.0 ml/kg/min [34] (Table 15.3). In renal failure a decrease in clearance is observed leading to a prolonged duration of action, because biliary excretion cannot compensate for the decrease in glomerular filtration. Plasma clearance is reduced in patients with hepatic disease. However resistance to the initial dose is frequently observed in patients with liver cirrhosis. The resistance is explained by a larger distribution volume. No differences in pharmacokinetics have been observed between adults and children. In the elderly, the plasma clearance is lower than in young adults because glomerular filtration decreases with age.
The ED50 and ED95 at the adductor pollicis are 0.03 mg/kg and 0.05 mg/kg, respectively. The ED50 at the diaphragm is twice the ED50 at the adductor pollicis. Thus complete paralysis of the diaphragm is not expected with a dose that barely blocks the adductor pollicis [35]. Onset time of maximum neuromuscular block is about 3.5 min after administration of 0.10 mg/kg (Table 15.4). The use of a priming dose shortens the onset time. However, priming must be avoided, because of the risk of pulmonary aspiration and paralysis of upper airway muscles, which are more sensitive than the adductor pollicis to small doses of NDMR [36]. Following administration of twice the ED95, the clinical duration of action and total duration of action are close to 70–80 and 120 min, respectively. Recovery at the diaphragm is faster than at the adductor pollicis because it is more resistant to NDMR than the adductor pollicis. Accumulation has been demonstrated with pancuronium inducing a progressive increase in the duration of action after repeated doses. Recovery is accelerated by neostigmine or edrophonium. In clinical practice, when the fourth response at the TOF is detected, neostigmine can be injected safely. Although edrophonium has a shorter duration of action than neostigmine, its use to antagonise residual neuromuscular block induced by pancuronium must be cautious because of its shorter duration of action. The halogenated agents induce a shift to the right of the dose–action curve. This interaction is concentration and duration of administration dependent. The potentiating effects of sevoflurane and desflurane are greater than that induced by other halogenated agents.
Pancuronium increases heart rate, arterial pressure and cardiac output. These cardiovascular effects are related to vagolytic and sympathomimetic effects (Table 15.2). The use of pancuronium in cardiovascular high-risk patients is discussed. Some authors recommend its use to counteract the bradycardia induced by high doses of opioids. Clinical use of pancuronium is now limited since the introduction of new compounds of intermediate duration of action. Due to its long duration of action, residual curarisation in the recovery room is more frequent with pancuronium when compared with vecuronium or atracurium. Its use is limited to long surgical procedures or when mechanical ventilation is needed in the postoperative period.
Pipecuronium
Pipecuronium bromide is a long-acting steroidal NDMR which resembles pancuronium in potency and duration of action. Pipecuronium is metabolised in the liver (Table 15.1) to a 3-OH derivative which has 40–50 % the potency of the parent drug. Renal elimination of this compound affects 37–41 % of the administered dose; biliary excretion is negligible (2 %). Pharmacokinetics of pipecuronium differs from pancuronium by a greater plasma clearance and a larger volume of distribution [37] (Table 15.3). Plasma clearance is decreased from 2.4 to 1.6 mL/min/kg in patients with renal failure, leading to a prolongation of the elimination half-life from 137 to 263 min. In humans, in the presence of cholestasis, neither the pharmacokinetics nor the time course of action was significantly different from those of normal patients.
The ED95 is approximately 0.045 mg/kg. Administration of a 0.070–0.08 mg/kg dose results in a complete block in 3.0–3.5 min, with a clinical duration of action of 70–110 min at the adductor pollicis (Table 15.3). Like other NDMRs, a bolus of pipecuronium exerts a greater effect in adults and infants than in children. In elderly patients (>70 years of age), the onset of action is longer than in younger patients, but the duration of action is not significantly prolonged if renal function is normal. Pipecuronium is comparable to other long-acting relaxants in terms of potentiation of neuromuscular block by halogenated agents and reversal by anticholinesterase drugs. Edrophonium is relatively ineffective against pipecuronium-induced neuromuscular block even when recovery has taken place.
Pipecuronium is devoid of cardiovascular side effects. It lacks the autonomic effects of pancuronium and does not cause histamine release (Table 15.2). Doses up to three times the ED95 do not induce significant changes in heart rate or blood pressure in patients undergoing coronary artery bypass. Because of its long duration of action and interindividual variability in response, the use of pipecuronium is limited to long surgical procedures.
Benzylisoquinoline Compounds (Fig. 15.6)
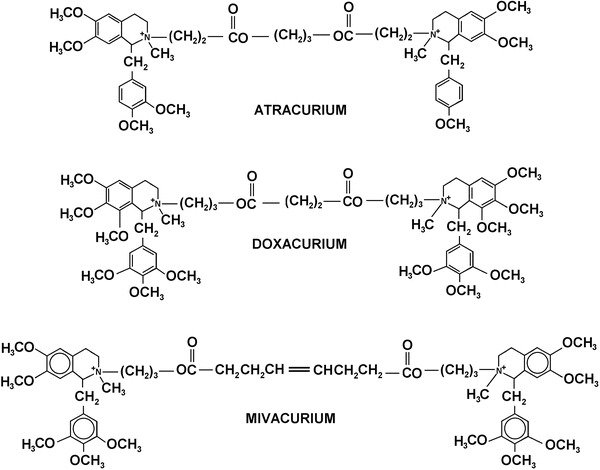
Fig. 15.6
Chemical structures of benzylisoquinoline NMBAs
Atracurium
Atracurium is a bulky diester with two positively charged nitrogen atoms. Atracurium presents four asymmetrical centres so that there are 16 possible isomers. It is metabolised by ester hydrolysis and Hofmann elimination (Table 15.1) which involves the conversion of an amide to an amine under alkaline conditions. Hofmann elimination for atracurium takes place at a pH of 7.4 and a temperature of 37 °C. Breakdown products of atracurium include laudanosine, acrylates and quaternary organic acids [38] (Fig. 15.7). High laudanosine plasma concentrations (17 mg/L) can be associated with convulsions in dogs but have never been implicated in humans. The acrylates are highly reactive substances which may be hepatotoxic although once again no problems have been reported in humans [39]. The ratio of ester hydrolysis to Hofmann elimination differs from species to species, but in humans up to about 60 % probably undergoes ester hydrolysis in the liver, although there is not a uniform agreement. The breakdown of atracurium is independent of plasma cholinesterase. The volume of distribution in normal patients is approximately 0.18 L/kg and clearance 5.5 ml/kg/min. The elimination half-life is 20 min and remains remarkably constant throughout studies (Table 15.3). In patients with renal failure, several pharmacokinetic and pharmacodynamic studies have shown no significant changes [40]. However recovery has been found to be prolonged following repeated doses in patients with renal failure. Laudanosine may accumulate in patients with renal failure, but the concentration attained after a continuous administration is ten times less than the toxic plasma level. In patients with fulminant hepatic failure, the volume of distribution is significantly increased but the elimination half-life (23 min) remains unchanged.
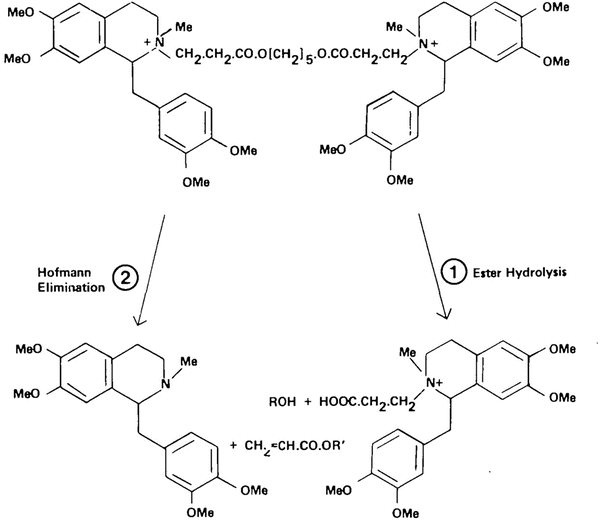
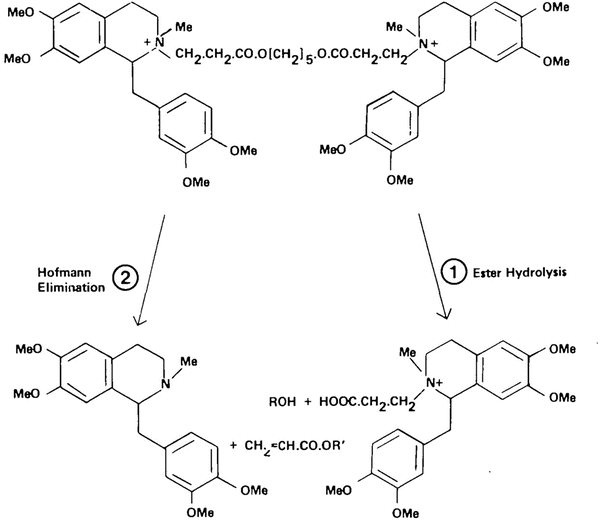
Fig. 15.7
Metabolic pathways for atracurium degradation in humans. Reproduced from Hughes and Chapple [38] with permission from Oxford University Press
The ED50 of atracurium is 0.13 mg/kg, and the ED95 is 0.23 mg/kg (Table 15.3). The onset of action at the adductor pollicis of one ED95 is about 3–5 min. It can be reduced by increasing the dose, but doses above 3 times the ED95 can induce histamine release. The duration of action depends upon the dose administered; an intubating dose (0.50 mg/kg) provides muscular relaxation for 25–40 min in adults. Neuromuscular block is easily antagonised by neostigmine or edrophonium. Atracurium pharmacodynamics are very little affected in the elderly, probably because atracurium elimination is organ independent. The onset time is more rapid and the duration of action slightly shorter in children than in adults. The breakdown of atracurium is decreased by hypothermia, causing a reduction in dose requirements during hypothermic cardiopulmonary bypass. Atracurium can induce histamine release (Table 15.2) and a drop in blood pressure at a dose of 3 times the ED95. Histamine release can be reduced by slow IV injection.
Atracurium is indicated for intermediate to long duration of action procedures and for day case procedures. Due to its lack of accumulation, maintenance can be obtained by a continuous infusion at a rate of 5–10 μg/kg/min.
Cisatracurium
Cisatracurium is one of ten isomers of atracurium. Hofmann elimination is the predominant elimination pathway. Ester hydrolysis plays a limited role in humans (Table 15.1). The two metabolites are laudanosine and a monoquaternary alcohol. Urinary excretion is a minor elimination pathway but a major pathway for the elimination of laudanosine. Because of a greater potency than atracurium, the dose administered is lower, and the production of laudanosine is lower [41]. The mean clearance ranges from 4.5 to 5.7 mL/min/kg and the elimination half-life is 22–30 min [42] (Table 15.4).
The ED95 is 0.048 mg/kg. At twice the ED95, the pharmacodynamic profile of cisatracurium is similar to that of an equipotent dose of atracurium apart from a slower onset of action (Table 15.4). A more rapid onset is produced when increasing the dose (2.7 min at 0.2 mg/kg). The clinical duration of action ranges from 45 min after twice the ED95 to approximately 90 min at eight times the ED95. The recovery index is constant after repeated bolus doses or continuous infusions suggesting a lack of cumulation [43]. When neostigmine is given at 10 % of T1, a complete recovery from neuromuscular block is obtained in 7 min. The intensity of block and the clinical duration of action are not modified by renal failure. The same results are observed in patients with hepatic failure except for the onset of action which is significantly shorter in case of hepatic dysfunction. Contrary to atracurium, cisatracurium does not produce histamine release (Table 15.2) at doses up to 8 times the ED95.
Mivacurium
Mivacurium is a benzylquinolinium diester with three isomers. It is hydrolysed by plasma cholinesterase. Clearance of the cis–trans and trans–trans isomers correlates with plasma pseudocholinesterase activity. The cis–cis isomer is more slowly hydrolysed (Table 15.3), but its potency is only one-tenth that of the two other isomers. Mivacurium is hydrolysed at 70–88 % of the rate of succinylcholine. The ED95 is 0.07–0.08 mg/kg at the adductor pollicis. Onset time is 3 min and the clinical duration of action is 20 min following 0.2 mg/kg (Table 15.4). Mivacurium shows few cumulative effects; the recovery index is 6–7 min and does not change over a wide dose range nor after prolonged infusion up to 5 h. Administration of neostigmine or edrophonium, after the start of spontaneous recovery, reduces the recovery index by approximately 50 %. Prolonged block has been reported following its use in patients with low or abnormal plasma cholinesterase [44]. In patients heterozygous for the atypical and usual gene, the duration of action is about 50 % prolonged [45]. Mivacurium is four to five times more potent in homozygous abnormal patients than in patients with normal phenotype. In these patients the duration of action following 0.2 mg/kg may be very prolonged (6–8 h). Anticholinesterases should not be administered. Patients must be ventilated and sedated until spontaneous recovery occurs. Renal failure may reduce cholinesterase activity by 30–50 %; the duration of action may be prolonged in these patients. There is an inverse correlation between plasma cholinesterase activity and the duration of action of mivacurium in cirrhotic patients. Mivacurium may induce histamine release which is dose and rate of administration dependent (Table 15.2). A dose of 0.2 mg/kg can induce a drop in blood pressure which can be attenuated by slower injection (30 s).
The main advantage of mivacurium is a short duration of action which makes it suitable for short surgical procedures requiring tracheal intubation. A dose of 0.2 mg/kg is necessary to obtain good intubating conditions. Maintenance of neuromuscular block can be obtained by a continuous infusion at a rate of 5–10 μg/kg/min. Monitoring is mandatory during continuous administration to detect recovery or prolonged duration of action in patients with abnormal pseudocholinesterases.
Fumarate Compounds (Fig. 15.8)
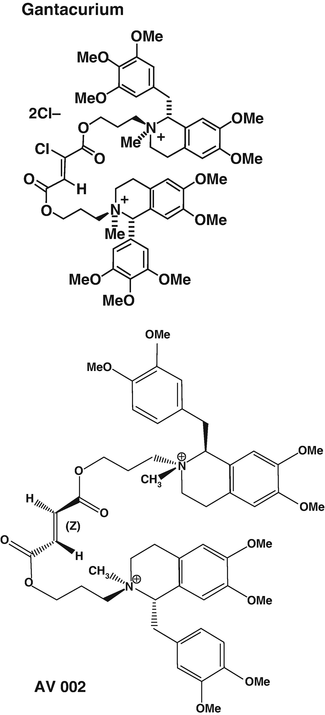
Fig. 15.8
Chemical formulae of gantacurium and CW002 also named AV002. Reproduced from Lien et al. [46] with permission from Elsevier
These compounds share some structural properties with mivacurium. Gantacurium underwent clinical testing in humans a few years ago. It was shown that following 2–3 times the ED95 at the adductor pollicis, onset time at both the laryngeal muscles and the adductor pollicis was comparable to onset of block following succinylcholine administration. Gantacurium does not exhibit ganglionic blockade or vagolytic properties. However histamine release at 4 times the ED95 and a 17 % reduction in blood pressure in humans has hampered its clinical development [46]. Interestingly, gantacurium is metabolised by a slow pH-sensitive hydrolysis and also by adduction of the naturally occurring amino acid cysteine transforming gantacurium into an inactive compound. Administration of l-cysteine (10 mg/kg), 1 min after administration of gantacurium , results in return of complete neuromuscular function within 1–2 min [47].
CW002 is a gantacurium derivative of intermediate duration of action. Initial dose-finding studies in volunteers did not show any evidence of histamine release or bronchoconstriction. The resulting adduction of cysteine product retains some very low affinity for neuromuscular nAChR with potency for neuromuscular blockade decreased 70–100-fold [47]. CW002 is currently under clinical investigation.
Action of Relaxants on Different Muscle Groups
In man, several studies have reported some discrepancies between the level of peripheral paralysis and respiratory depression or intubating conditions. Paton and Zaimis demonstrated in 1951 that some of the muscles of respiration, such as the diaphragm, were more resistant to curare than others. The dose of NDMR needed to block the diaphragm is 1.5–2 times that of the adductor pollicis. Thus, complete paralysis of the diaphragm is not expected with doses of neuromuscular blocking agents used to block neuromuscular transmission at the adductor pollicis [28]. Similarly, the laryngeal adductor muscles are more resistant to NDMR than the more peripheral muscles such as the adductor pollicis, for which all dosing recommendations for neuromuscular blocking agents and their antagonists have been made [29]. The sparing effect on the laryngeal adductor muscles has been documented with vecuronium, rocuronium (Fig. 15.9) [33], cisatracurium and mivacurium. Plaud et al. studied the pharmacokinetic–pharmacodynamic relationship of blocking agents at the adductor pollicis and the laryngeal adductors. They found that the concentration in the effect compartment producing 50 % of the maximum block was significantly greater at the laryngeal adductor muscles (1.5 μg/mL) than that at the adductor pollicis (0.8 μg/ml) (Fig. 15.10) [48]. There is convincing evidence that the EC50 for almost all drugs is 50–100 % higher at the diaphragm or larynx than it is at the adductor pollicis. These differences may be due to any of several factors. Waud and Waud found that following curare administration, neuromuscular transmission occurs when approximately 18 % of the receptors are free at the diaphragm, while it does not occur at the peripheral muscles unless 29 % of receptors are free [49]. This may be due to higher receptor density, greater release of acetylcholine or less acetylcholinesterase activity [50]. The lower density of acetylcholine receptors in slow muscle fibres, such as found in the peripheral muscles, explains, at least in part, the lower margin of safety for neuromuscular transmission when compared to that in the faster muscle fibres in the laryngeal adductors. Interestingly, muscle sensitivity to succinylcholine is different than with other neuromuscular blocking agents [17]. It is the only muscle relaxant that, at equipotent doses, causes greater neuromuscular block at the vocal cords than at the adductor pollicis. Some data suggest that in contrast to NDMR, succinylcholine is more effective in blocking the muscles composed of primarily fast-contracting fibres [51].
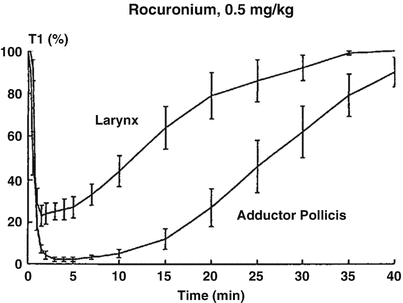
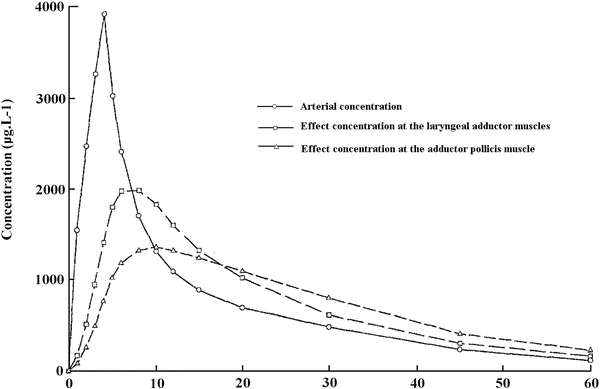
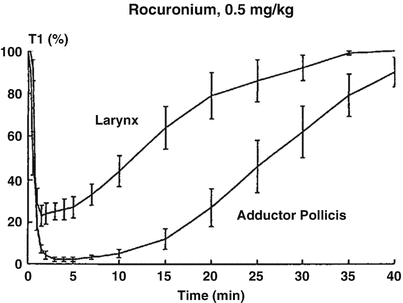
Fig. 15.9
Onset and recovery of rocuronium-induced neuromuscular block at the laryngeal adductor muscles and the adductor pollicis. Reproduced from Meistelman et al. [33] with permission from Springer
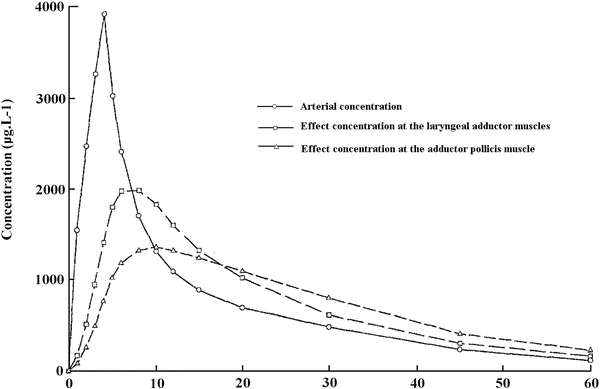
Fig. 15.10
Rocuronium plasma concentration and calculated concentration in the effect compartment at the laryngeal adductor muscles and the adductor pollicis versus time. Reproduced from Plaud et al. [48] with permission from John Wiley and Sons
In spite of their relative resistance to neuromuscular blockers, the onset of neuromuscular block is significantly faster at the diaphragm and the laryngeal adductors than at the adductor pollicis. Fisher et al. [52] postulated that a more rapid equilibration (shorter t ½ke0) of the neuromuscular blocking agent between plasma and the effect compartment at these more centrally located muscles was the explanation for this observation. The accelerated rate of equilibrium probably represents little more than differences in regional blood flow. Therefore, muscle blood flow rather than a drug’s intrinsic potency may be more important in determining the onset and offset time of NDMR. Greater blood flow per gram of muscle at the diaphragm or larynx results in them receiving a higher peak plasma concentration of drug in the brief period of time before rapid redistribution occurs. Plaud et al. have confirmed this hypothesis by demonstrating a faster transfer rate constant (t ½ke0) at the laryngeal adductors (2.7 min) than at the adductor pollicis (4.4 min). Greater resistance to neuromuscular blockade accounts for the more rapid recovery of the respiratory muscles and the muscles of the abdominal wall than at the adductor pollicis. Recovery occurs more rapidly because blood concentration of NMBA has to decrease more in the muscles of respiration than in the adductor pollicis for recovery of neuromuscular function to begin.

Full access? Get Clinical Tree
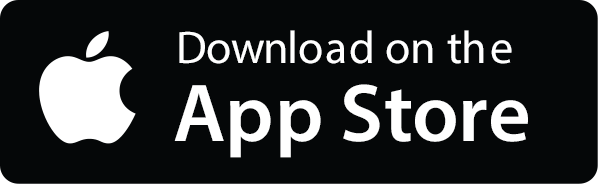
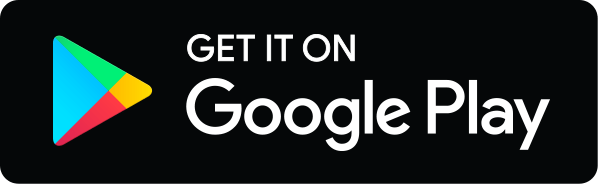