Neuromuscular blocking drugs (NMBDs) interrupt transmission of nerve impulses at the neuromuscular junction (NMJ) and thereby produce paresis or paralysis of skeletal muscles. On the basis of electrophysiologic differences in their mechanisms of action and duration of action, these drugs can be classified as depolarizing NMBDs (mimic the actions of acetylcholine [ACh]) and nondepolarizing NMBDs (interfere with the actions of ACh), the latter of which are further subdivided into long-, intermediate-, and short-acting drugs ( Box 11.1 ). Succinylcholine (SCh) is the only depolarizing NMBD used clinically. It is also the only NMBD that has both a rapid onset and ultrashort duration of action. Among the nondepolarizing NMBDs, rocuronium’s rapid onset time most closely resembles that of SCh.
Clinical Uses
The principal clinical uses of NMBDs are to produce skeletal muscle relaxation for facilitation of tracheal intubation and to provide optimal surgical working conditions. NMBDs may also be administered during cardiopulmonary resuscitation (also see Chapter 45 ) and to patients in emergency departments (also see Chapter 42 ) and intensive care units (also see Chapter 41 ) to facilitate mechanical ventilation of the patient’s lungs. Of prime importance is to recognize that NMBDs lack analgesic or anesthetic effects and should not be used to render an inadequately anesthetized patient paralyzed. An inadequately anesthetized but paralyzed patient is a major risk for awareness during general anesthesia (see Chapter 47 ). Ventilation of the lungs must be mechanically provided whenever significant skeletal muscle weakness is produced by NMBDs. Clinically, intraoperative clinical evaluation of neuromuscular blockade is typically provided by visually monitoring the mechanical response (twitch response) produced by electrical stimulation of a peripheral nerve (usually a branch of the ulnar or facial nerve) delivered from a peripheral nerve stimulator (see the section, “Monitoring the Effects of Nondepolarizing Neuromuscular Blocking Drugs” ). This chapter places increased emphasis on the value of monitoring by use of a peripheral nerve stimulator when NMBDs are given. Also, neostigmine has been the standard “reversal” drug for a nondepolarizing neuromuscular blockade; sugammadex is a relatively new reversal drug that has a unique mechanism of action and specifically reverses a rocuronium- and vecuronium-induced neuromuscular blockade.
Choice of Neuromuscular Blocking Drug
The choice of NMBD is influenced by its speed of onset, duration of action, route of elimination, and associated side effects, such as drug-induced changes in systemic arterial blood pressure, heart rate, or both. Rapid onset and brief duration of skeletal muscle paralysis, characteristic of SCh, are useful when tracheal intubation is the reason for administering an NMBD. Because of its rapid onset time, rocuronium is often used to facilitate tracheal intubation, but its duration of action is much longer than that of SCh. However, an approved indication for sugammadex is reversal of a profound neuromuscular blockade specifically from rocuronium or vecuronium. For example, if rocuronium were given to facilitate endotracheal intubation, but the trachea could not be intubated, sugammadex could reverse a profound neuromuscular blockade. Although SCh can be given intermittently, nondepolarizing NMBDs are usually selected when longer periods of neuromuscular blockade (e.g., more than 15 to 45 minutes) are needed. When rapid onset of skeletal muscle paralysis is not necessary, skeletal muscle relaxation can be induced by the administration of other long- or intermediate-acting nondepolarizing NMBDs to facilitate tracheal intubation.
Hypersensitivity Reactions
The overall incidence of life-threatening anesthetic-related hypersensitivity reactions ranges between 1/10,000 and 1/20,000 procedures and varies widely between countries. Although antibiotics are likely the most common cause, NMBDs are the triggering drugs in 11% to 35% of these reactions. Rocuronium and SCh are the most common offenders. Even though it does not release histamine, rocuronium was identified as producing an increased risk for hypersensitivity reactions in France and Norway, with no confirmation from other countries. More recently, a follow-up study from Norway of 83 cases of anaphylaxis during general anesthesia revealed that 77% of these reactions were mediated by immunoglobulin E and 93% were associated with NMBDs, with SCh being the most common drug. In an analysis of all allergic drugs used in anesthesia at the Mayo Clinic, antibiotics were the most common cause, with NMBDs being second at 11% of the reactions. There may be cross-sensitivity among all NMBDs because of the presence of a common antigenic component, the quaternary ammonium group. Anaphylactic reactions after the first exposure to an NMBD may reflect sensitization from previous contact with cosmetics or soaps that also contain antigenic quaternary ammonium groups. Sugammadex (see the section, “Antagonism of Nondepolarizing Neuromuscular Blocking Drugs” later in this chapter) was recently approved by the Food and Drug Administration (FDA). The delay in sugammadex’s approval was partly because of hypersensitivity concerns. The conclusion was that the most common signs of occasional cases of hypersensitivity were nausea and urticaria. However, sugammadex has been approved in Europe and other countries for several years. Treatment of a life-threatening hypersensitivity reaction requires immediate therapy including cardiopulmonary resuscitation and epinephrine (see Chapter 45 for details).
Neuromuscular Junction
The anatomy of the NMJ consists of a prejunctional motor nerve ending separated from the highly folded postjunctional membrane of the skeletal muscle by a synaptic cleft ( Fig. 11.1 ). Nicotinic acetylcholine receptors (nAChRs) are located at pre- and postjunctional sites. Neuromuscular transmission is initiated by arrival of an impulse at the motor nerve terminal with an associated influx of calcium ions and resultant release of the ligand ACh. ACh binds to AChRs (the ligand-gated channel) on postjunctional membranes and thereby causes a change in membrane permeability to ions, principally potassium and sodium. This change in permeability and movement of ions causes a decrease in the transmembrane potential from about −90 mV to −45 mV (threshold potential), at which point a propagated action potential spreads over the surfaces of skeletal muscle fibers and leads to muscular contraction. ACh is rapidly hydrolyzed (within 15 ms) by the enzyme acetylcholinesterase (true cholinesterase), thus restoring membrane permeability (repolarization) and preventing sustained depolarization. Acetylcholinesterase is primarily located in the folds of the end-plate region, which places it in close proximity to the site of action of ACh.

Prejunctional Receptors and Release of Acetylcholine
ACh is synthesized in the motor nerve terminal, and the protein synapsin anchors the ACh vesicle to the release site of the terminal. Some of the ACh is then released, and the rest is held in reserve for response to a stimulus. Presynaptic receptors, aided by calcium, facilitate replenishment of the motor nerve terminal, which can be stimulated by SCh and neostigmine and depressed by small doses of nondepolarizing NMBDs. Inhibition of these presynaptic nAChRs explains the fade in response to high-frequency repetitive stimulation such as tetanic or even train-of-four (TOF) stimulation.
Postjunctional Receptors
Postjunctional receptors are glycoproteins consisting of five subunits ( Fig. 11.2 ). The subunits of the receptor are arranged such that a channel is formed that allows the flow of ions along a concentration gradient across cell membranes. This flow of ions is the basis of normal neuromuscular transmission. Extrajunctional receptors retain the two α-subunits but may have an altered γ- or δ-subunit by the substitution of an ε-unit.

The two α-subunits are the binding sites for ACh and are the sites occupied by NMBDs. For example, occupation of one or both α-subunits by a nondepolarizing NMBD causes the ion channel to remain closed, and ion flow to produce depolarization cannot occur. SCh attaches to α-sites and causes the ion channel to remain open (mimics ACh), thereby resulting in prolonged depolarization. Large doses of nondepolarizing NMBDs (large molecules) may also act to occlude the channel and in this way prevent the normal flow of ions. Neuromuscular blockade secondary to occlusion of the channels is resistant to drug-enhanced antagonism with anticholinesterase drugs. The lipid environment around cholinergic receptors can be altered by drugs such as volatile anesthetics, thus changing the properties of the ion channels. This probably accounts for the augmentation of neuromuscular blockade by volatile anesthetics.
Extrajunctional Receptors
Postjunctional receptors are confined to the area of the end plate precisely opposite the prejunctional receptors, whereas extrajunctional receptors (the ε-unit is replaced by γ-subunits) are present throughout skeletal muscles. Extrajunctional receptor synthesis is normally suppressed by neural activity. Prolonged inactivity, sepsis, and denervation or trauma (burn injury) to skeletal muscles may be associated with a proliferation of extrajunctional receptors. When activated, extrajunctional receptors stay open longer and permit more ions to flow, which in part explains the exaggerated hyperkalemic response when SCh is administered to patients with denervation or burn injury. Proliferation of these receptors also accounts for the resistance or tolerance to nondepolarizing NMBDs, as can occur with burns or prolonged (several days) immobilization (also see discussion under the section, “Hyperkalemia” ).
Structure-Activity Relationships
NMBDs are quaternary ammonium compounds that have at least one positively charged nitrogen atom that binds to the α-subunit of postsynaptic cholinergic receptors ( Fig. 11.3 ). In addition, these drugs have structural similarities to the endogenous neurotransmitter ACh. For example, SCh is two molecules of ACh linked by methyl groups. The long, slender, flexible structure of ACh allows it to bind to and activate cholinergic receptors. The bulky rigid molecules that are characteristic of nondepolarizing NMBDs, though containing portions similar to ACh, do not activate cholinergic receptors.

Nondepolarizing NMBDs are either aminosteroid compounds (pancuronium, vecuronium, rocuronium) or benzylisoquinolinium compounds (atracurium, cisatracurium, mivacurium). Pancuronium is the bisquaternary aminosteroid NMBD most closely related to ACh structurally. The ACh-like fragments of pancuronium give the steroidal molecule its high degree of neuromuscular blocking activity. Vecuronium and rocuronium are monoquaternary analogs of pancuronium. Aminosteroid NMBDs lack hormonal activity. Benzylisoquinolinium derivatives are more likely than aminosteroid derivatives to evoke the release of histamine, presumably reflecting the presence of a tertiary amine.
Depolarizing Neuromuscular Blocking Drugs
SCh is the only depolarizing NMBD used clinically. Furthermore, it is the only NMBD with both a rapid onset and ultrashort duration of action. Typically, doses of 0.5 to 1.5 mg/kg are administered intravenously and produce a rapid onset of skeletal muscle paralysis (30 to 60 seconds) that lasts 5 to 10 minutes because of its unique breakdown ( Fig. 11.4 ). These characteristics make SCh ideal for providing rapid skeletal muscle paralysis to facilitate tracheal intubation. SCh has been used clinically for more than 60 years. Despite consistent industrial efforts, no drug has been developed that is better than SCh for tracheal intubation. Although an intravenous dose of 0.5 mg/kg may be adequate, 1.0 to 1.5 mg/kg is commonly administered to facilitate tracheal intubation. If a subparalyzing dose of a nondepolarizing NMBD (pretreatment with 5% to 10% of its 95% effective dose [ED 95 ]) is administered 2 to 4 minutes before injection of SCh to blunt fasciculations, the dose of SCh should be increased by about 70%. Although ideal for facilitating tracheal intubation, SCh has many adverse effects ( Box 11.2 ). As an alternative, the intermediate-acting nondepolarizing NMBD rocuronium has an onset time as rapid as SCh in doses ranging from 1.0 to 1.2 mg/kg.

Cardiac dysrhythmias
Sinus bradycardia
Junctional rhythm
Sinus arrest
Fasciculations
Hyperkalemia
Myalgia
Myoglobinuria
Increased intraocular pressure
Increased intragastric pressure
Trismus
Characteristics of Blockade
SCh mimics the action of ACh and produces a sustained depolarization of the postjunctional membrane. Skeletal muscle paralysis occurs because a depolarized postjunctional membrane and inactivated sodium channels cannot respond to subsequent release of ACh (hence, the designation depolarizing neuromuscular blockade). Depolarizing neuromuscular blockade is also referred to as phase I blockade . Phase II blockade is present when the postjunctional membrane has become repolarized but still does not respond normally to ACh (desensitization neuromuscular blockade). The mechanism of phase II blockade is unknown but may reflect the development of nonexcitable areas around the end plates that become repolarized but nevertheless prevent the spread of impulses initiated by the action of ACh. With the initial dose of SCh, subtle signs of a phase II blockade begin to appear (fade to tetanic stimulation). Phase II blockade, which resembles the blockade produced by nondepolarizing NMBDs, predominates when the intravenous dose of SCh exceeds 3 to 5 mg/kg ( Table 11.1 ).
Succinylcholine | |||
---|---|---|---|
Feature | Phase I | Phase II | Rocuronium |
Administration of rocuronium | Antagonize | Augment | Augment |
Administration of succinylcholine | Augment | Augment | Antagonize |
Administration of neostigmine | Augment | Antagonize | Antagonize |
Fasciculations | Yes | No | |
Response to single electrical stimulation (single twitch) | Decreased | Decreased | Decreased |
Train-of-four ratio | >0.7 | <0.3 | <0.3 |
Response to continuous (tetanus) electrical stimulation | Sustained | Unsustained | Unsustained |
Post-tetanic facilitation | No | Yes | Yes |
The sustained depolarization produced by the initial administration of SCh is initially manifested as transient generalized skeletal muscle contractions known as fasciculations . Furthermore, the sustained opening of sodium channels produced by SCh is associated with leakage of potassium from the interior of cells sufficient to increase plasma concentrations of potassium by about 0.1 to 0.4 mEq/L. With proliferation of extrajunctional nAChRs and damaged muscle membranes, many more channels will leak potassium and thereby lead to acute hyperkalemia.
Metabolism
Hydrolysis of SCh to inactive metabolites is accomplished by plasma cholinesterase (pseudocholinesterase) produced in the liver (see Fig. 11.4 ). Plasma cholinesterase has an enormous capacity to hydrolyze SCh at a rapid rate (ACh is metabolized even more rapidly by acetylcholinesterase) such that only a small fraction of the original intravenous dose reaches the NMJ. Because plasma cholinesterase is not present at the NMJ, the neuromuscular blockade produced by SCh is terminated by its diffusion away from the NMJ into extracellular fluid. Therefore, plasma cholinesterase influences the duration of action of SCh by controlling the amount of SCh that is hydrolyzed before reaching the NMJ. Liver disease must be severe before decreases in the synthesis of plasma cholinesterase are sufficient to prolong the effects of SCh. Potent anticholinesterases, as used in the treatment of myasthenia gravis, and certain chemotherapeutic drugs (nitrogen mustard, cyclophosphamide) may so decrease plasma cholinesterase activity that prolonged skeletal muscle paralysis follows the administration of SCh.
Atypical Plasma Cholinesterase
Atypical plasma cholinesterase lacks the ability to hydrolyze ester bonds in drugs such as SCh and mivacurium. The presence of this atypical enzyme is often recognized only after an otherwise healthy patient experiences prolonged skeletal muscle paralysis (>1hour) after the administration of a conventional dose of SCh or mivacurium. Subsequent determination of the dibucaine number permits diagnosis of the presence of atypical plasma cholinesterase. Dibucaine is an amide local anesthetic that inhibits normal plasma activity by about 80%, whereas the activity of atypical enzyme is inhibited by about 20% ( Table 11.2 ). The dibucaine number reflects the quality of plasma cholinesterase (ability to metabolize SCh and mivacurium) and not the quantity of enzyme that is circulating in plasma. For example, decreases in plasma cholinesterase activity because of liver disease or anticholinesterases are often associated with a normal dibucaine number.
Variants of Plasma Cholinesterase | Type of Buty butyrylcholinesterase/TG lcholinesterase | Incidence | Dibucaine Number (% Inhibition of Enzyme Activity) | Duration of Succinylcholine-Induced Neuromuscular Blockade (min) |
---|---|---|---|---|
Homozygous, typical (usual, U) | UU | Normal | 70-80 | 5-10 |
Heterozygous | UA | 1/480 | 50-60 | 20 |
Homozygous, atypical (A) | AA | 1/3200 | 20-30 | 60-180 |
Adverse Side Effects
Adverse side effects after the administration of SCh are numerous and may limit or even contraindicate the use of this NMBD in certain patients (see Box 11.2 ). After 60 years of use, SCh continues to cause serious complications. SCh usually should not be given to patients 24 to 72 hours after major burns, trauma, and extensive denervation of skeletal muscles because it may result in acute hyperkalemia and cardiac arrest. Administration of SCh to apparently healthy boys with unrecognized muscular dystrophy has resulted in acute hyperkalemia and cardiac arrest. For this reason, the FDA has issued a warning against the use of SCh in children, except for emergency control of the airway.
Cardiac Dysrhythmias
Sinus bradycardia, junctional rhythm, and even sinus arrest may follow the administration of SCh. These responses reflect the action of SCh at cardiac postganglionic muscarinic receptors, where this drug mimics the normal effects of ACh ( Table 11.3 ). Cardiac dysrhythmias are most likely to occur when a second intravenous dose of SCh is administered about 5 minutes after the first dose. Intravenous administration of atropine 1 to 3 minutes before SCh decreases the likelihood of these cardiac responses. Yet, atropine administered intramuscularly with the preoperative medication does not reliably protect against SCh-induced decreases in heart rate. The effects of SCh at autonomic nervous system ganglia also mimic the actions of the neurotransmitter ACh and may be manifested as ganglionic stimulation with associated increases in systemic blood pressure and heart rate (see Table 11.3 ).
Drug a | Nicotinic Receptors at Autonomic Ganglia | Cardiac Postganglionic Muscarinic Receptors | Histamine Release |
---|---|---|---|
Succinylcholine | Modest stimulation | Modest stimulation | Minimal |
Pancuronium | None | Modest blockade | None |
Vecuronium | None | None | None |
Rocuronium | None | None | None |
Atracurium | None | None | Slight b |
Cisatracurium | None | None | None |
Mivacurium | None | None | Slight b |
a At 95% effective dose (ED 95 ).
Hyperkalemia
Administration of SCh can rapidly result in massive hyperkalemia, serious cardiac arrythmias, and even cardiac arrest. In some patients, potassium levels can exceed 10 mEq/L. The classic conditions that lead to hyperkalemia after SCh include burns, trauma, and spinal cord or other major neurologic damage. Any time prolonged skeletal muscle inactivity (critical care) or extensive muscle damage exists, patients may be susceptible to hyperkalemia 48 hours after injury, and this is dependent on the development of extrajunctional, atypical receptors as previously described. When muscle has returned to its normal state, hyperkalemia will not occur. However, the judgment as to the “normal” state of the muscle is a clinically difficult estimation. In addition, extrajunctional receptors and hyperkalemia will develop in any patient who is immobile (critical care patients) for several days if SCh is given. For example, cardiac arrest has occurred when SCh has been used for emergency endotracheal intubation in the intensive care unit. The use of SCh for urgent tracheal intubation is contraindicated or not allowed in many intensive care units. The duration of susceptibility to the hyperkalemic effects of SCh is unknown, but the risk is probably decreased 3 to 6 months after denervation injury. All factors considered, it might be prudent to avoid administration of SCh to any patient more than 24 hours after a burn injury, extensive trauma, or spinal cord transection or who may become an intensive care patient.
Even though they may have increased potassium levels, patients with renal failure are not susceptible to an exaggerated release of potassium, and SCh can be safely administered to these patients, unless they have uremic neuropathy.
Myalgia
Postoperative skeletal muscle myalgia, manifested particularly in the muscles of the neck, back, and abdomen, may follow the administration of SCh. Myalgia localized to neck muscles may be described as a “sore throat” by the patient and incorrectly attributed to the previous presence of a tracheal tube. Young adults undergoing minor surgical procedures that permit early ambulation seem most likely to complain of myalgia. Unsynchronized contractions of skeletal muscle fibers (fasciculations) associated with generalized depolarization lead to myalgia. Prevention of fasciculations by prior administration of subparalyzing doses of a nondepolarizing NMBD (pretreatment) or lidocaine will decrease the incidence but not totally prevent myalgia. Magnesium will prevent fasciculations but not myalgia. Nonsteroidal antiinflammatory drugs are effective in treating the myalgia.
Increased Intraocular Pressure
SCh causes a maximum increase in intraocular pressure 2 to 4 minutes after its administration. This increase in intraocular pressure is transient and lasts only 5 to 10 minutes. The mechanism by which SCh increases intraocular pressure is unknown, although contraction of extraocular muscles with associated compression of the globe may be involved. The concern that contraction of extraocular muscles could cause extrusion of intraocular contents in the presence of an open eye injury has resulted in the common clinical practice of avoiding the administration of SCh to these patients. This theory has never been substantiated and is challenged by the report of patients with an open eye injury in whom intravenous administration of SCh did not cause extrusion of globe contents. Furthermore, there is evidence that contraction of extraocular muscles does not contribute to the increase in intraocular pressure that accompanies the administration of SCh.
Increased Intracranial Pressure
Increases in intracranial pressure after the administration of SCh can occur but are of little or no concern.
Increased Intragastric Pressure
SCh causes unpredictable increases in intragastric pressure. When intragastric pressure does increase, it seems to be related to the intensity of fasciculations, thus emphasizing the potential value of preventing this skeletal muscle activity by prior administration of a subparalyzing dose of a nondepolarizing NMBD. An unproven hypothesis is that this increased intragastric pressure may cause passage of gastric fluid and contents into the esophagus and pharynx, with a subsequent risk for pulmonary aspiration.
Trismus
Incomplete jaw relaxation with masseter jaw rigidity after a halothane-SCh sequence is not uncommon in children (occurs in about 4.4% of patients) and is considered a normal response. In extreme cases this response may be so severe that the ability to mechanically open the patient’s mouth is limited. The difficulty lies in separating the normal response to SCh from the masseter rigidity that may be associated with malignant hyperthermia. Because SCh is not recommended for use in children, except for emergency airway control, trismus is less of an issue.

Full access? Get Clinical Tree
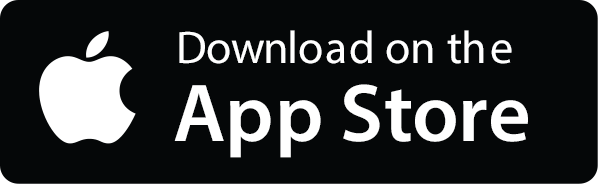
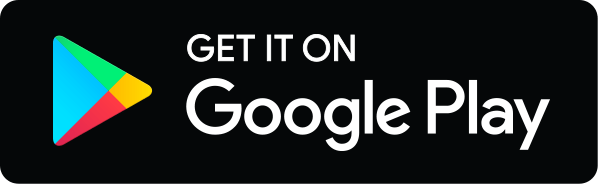