





u, a, s and f are the four commonest of 25 possible gene variants for plasma cholinesterase
Plasma cholinesterase may also exist in excess, as a genetic variant or particularly in the presence of obesity or alcoholism. The result is a shortened duration of action of suxamethonium.
Plasma cholinesterase is also responsible for the metabolism of mivacurium, and the concomitant use of these drugs in susceptible patients may exaggerate problems.
Phase I and phase II blockade
Two distinct types of neuromuscular blockade (termed phase I and II blockade respectively) may result after the administration of suxamethonium. Figure 32.5 lists the features of each type.
Phase I blockade
|
Phase II blockade
|
Non-depolarising blockade
Examples – atracurium, mivacurium, pancuronium, rocuronium, vecuronium
Mechanism of action
Non-depolarising neuromuscular blockade is the result of competitive occupancy of the postjunctional receptors preventing acetylcholine from reaching one or both α subunits of the receptor. This is probably a dynamic process, with both acetylcholine and the blocking agent in equilibrium with the receptors. At least 75% of receptors must be blocked before contraction fails. The process is competitive and reversible. The block can be antagonised by a local increase in acetylcholine, as produced by anticholinesterases. These muscle relaxants have no intrinsic activity at the neuromuscular endplate. Each has one or more quaternary ammonium groups. In the bisquaternary agents, these are usually 140 nm apart.
A graphic illustration of the process by which depolarising and non-depolarising drugs have their effects is shown in Figure 32.6.
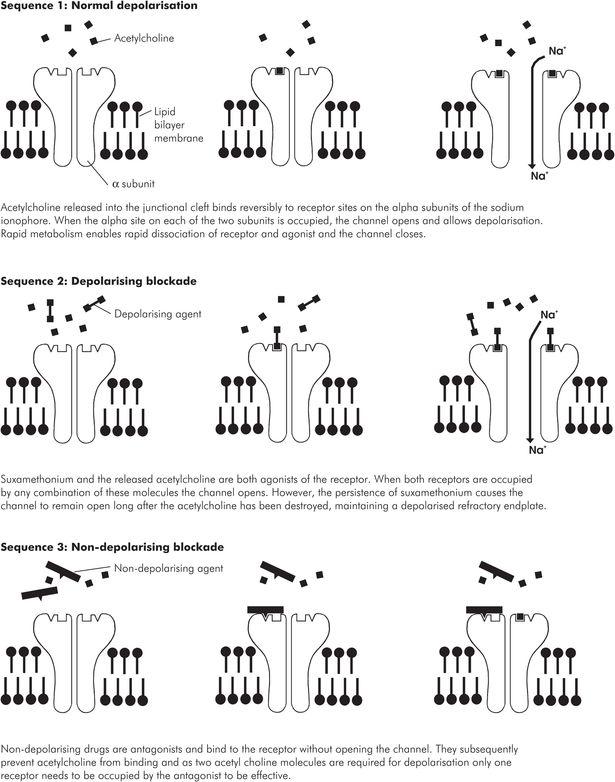
Action of depolarising and non-depolarising muscle relaxants
Clinical features
The predominant effect of neuromuscular blocking agents is a reversible paralysis of skeletal muscle. This reduction in skeletal muscle activity reduces venous return and therefore reduces cardiac output and blood pressure.
Neuromuscular test stimulation results in:
Reduced single-twitch height
Reduced train of four, with twitch amplitude 1 > 2 > 3 > 4
Tetanic fade
Post-tetanic facilitation
The neuromuscular blocking agents also have anticholinesterase activity (Figure 32.7).
Drug | Concentration to produce 50% inhibition of enzymatic acetylcholine breakdown (μmol L–1) in the presence of various neuromuscular blocking drugs | |
---|---|---|
Acetylcholinesterase | Plasma cholinesterase | |
Suxamethonium | 1300 | 640 |
Atracurium | 340 | 420 |
Vecuronium | 66 | 0.62 |
Aminosteroids
The acetylcholine-type fragment associated with the D ring of the steroid nucleus is probably responsible for most of the neuromuscular antagonism. The acetylcholine-type fragment associated with the A ring is probably responsible for the cardiovascular effects, especially the vagolytic aspects. Histamine release is not expected with the aminosteroid structure. In general, the aminosteroids are more slowly metabolised than the benzylisoquinoliniums.
Benzylisoquinolinium compounds
The chemical structure of benzylisoquinolinium is associated with histamine release. In general, the presence of an ester link promotes rapid degradation and metabolism, leading to a short half-life and rapid transition to complete recovery.
Elimination
The non-depolarising agents are usually metabolised in the liver. Other pathways exist, including spontaneous degradation and enzymatic hydrolysis within the plasma. These pathways are covered under Specific pharmacology, below.
The non-depolarising blocking agents may be potentiated by suxamethonium, intravenous and volatile anaesthetic drugs, opioids, aminoglycosides, tetracyclines, metronidazole, lincosamides, polymyxins, magnesium, verapamil and nifedipine, protamine, diuretics and catecholamine antagonists. The blockade is prolonged by hypothermia.
Anticholinesterases
Mechanism of action
The anticholinesterases inhibit the breakdown of acetylcholine by binding to the acetylcholinesterase enzyme in a competitive manner. This raises the background concentration of acetylcholine near the neuromuscular junction, which in turn overcomes the reduced number of functional nicotinic receptors on the muscle endplate, whether due to a reduced number of receptors (myasthenia gravis) or due to blockade of existing receptors (non-depolarising muscle relaxants). The anticholinesterases can be classified as either reversible anticholinesterases or organophosphorus compounds. While they all act on acetyl and plasma cholinesterase, the specific interaction with the enzyme varies between individual drugs.
Reversible anticholinesterases
Examples – distigmine, edrophonium, neostigmine, pyridostigmine
Acetylcholinesterase has an esteratic site and an anionic site in close proximity. Physiologically, the positively charged quaternary amine of acetylcholine binds to the anionic site. The acetyl ester combines with the esteratic site and the acetylcholine is hydrolysed. The anticholinesterases competitively occupy these sites and prevent acetylcholine access. The anticholinesterases have a quaternary amine group that is attracted to the anionic site and a carbamyl ester that binds covalently to the serine amino acid of the esteratic site. The quaternary amine group is not essential for activity, but when present it conveys enhanced potency and stability. The quaternary group also results in poor absorption following oral administration and a minimal transfer of the drug across the blood–brain barrier. Neostigmine is absorbed poorly from the gut as compared with the IV route. When neostigmine is used orally for the treatment of myasthenia gravis larger doses are therefore necessary. Equivalent doses of neostigmine are as follows: IV 0.5 mg; IM 1–1.5 mg; oral 15 mg.
Anticholinesterases also have some direct cholinergic agonist activity.
Physostigmine (no longer available) is an example of an anticholinesterase with several tertiary amine groups rather than the quaternary amine of the others. Consequently it is readily absorbed topically and orally and crosses the blood–brain barrier, but has no cholinergic agonist activity.
Edrophonium is only clinically effective for 5 minutes, and is used in the diagnosis of myasthenia gravis. Longer-acting agents are needed for treatment of the disease. Neostigmine is the only anticholinesterase routinely used to reverse planned neuromuscular blockade in clinical practice. Anticholinesterases have widespread effects subsequent to the stimulation of increased cholinergic muscarinic and nicotinic activity. Heart rate, vasomotor tone and blood pressure are reduced. At high dose levels sympathetic ganglion stimulation may predominate. Excess acetylcholine causes bronchoconstriction and increased bronchial secretion. Increased gastrointestinal tone and secretion occurs. Secretions of saliva, sweat and tears are also stimulated. These problems are prevented by the concomitant use of muscarinic anticholinergic drugs such as atropine or glycopyrrolate. Anticholinesterases can also cause a depolarising neuromuscular blockade when used in excess, or in the absence of non-depolarising blockade.
Neuromuscular blockade is terminated either by endogenous elimination of the drug and diffusion of the blocking agent away from the neuromuscular junction, or, in the case of non-depolarising agents, the effects can be overcome, in part, by inhibiting the metabolism of acetylcholine. If a long-acting muscle relaxant is used, it is possible for the blockade to re-establish if the effects of the anticholinesterase wear off before the neuromuscular blocking agent has left the receptors.
Organophosphorus compounds
Example – ecothiopate
These are primarily used as nerve gases and pesticides, and may feature in cases of poisoning. In general, they have no ionic binding component but bind covalently and irreversibly to the esteratic site of the cholinesterase enzyme by the release of a relatively weakly bound component of the drug. Consequently, the enzyme is not readily reactivated. Organophosphates tend to be highly lipid-soluble and readily cross the blood–brain barrier, causing central nervous system toxicity.
Ecothiopate differs from the other organophosphates in a number of ways. It is the only organophosphate in clinical use, and is used in the treatment of glaucoma. It too binds covalently to the esteratic site of the enzyme, but it also has a positively charged quaternary ammonium group that helps binding. This positive charge means that ecothiopate does not readily cross the blood–brain barrier, in contrast with other organophosphorous compounds. Although it is slowly hydrolysed it may nonetheless prolong the action of suxamethonium and mivacurium.
Selective binding agent
Example – sugammadex
Sugammadex is a selective relaxant binding agent specifically designed to target rocuronium (de Boer et al. 2006). It is a synthetic γ-cyclodextrin derivative (an oligosaccharide) devoid of intrinsic activity. In effect, each molecule forms a tube into which a single relatively small aminosteroid molecule of rocuronium or vecuronium fits. This binding is specific for rocuronium and vecuronium, effectively encapsulating it to rapidly reduce its unbound plasma concentration and also that at the neuromuscular endplate. Cyclodextrins are highly water-soluble, and sugammadex is excreted rapidly in the urine. The half-life is relatively short, and so further administration of rocuronium soon afterwards may be an option. An alternative benzylisoquinolinium non-depolariser could also be used, as these larger molecules will not fit within the cyclodextrin cavity.
In contrast with anticholinesterase reversal, sugammadex:

Full access? Get Clinical Tree
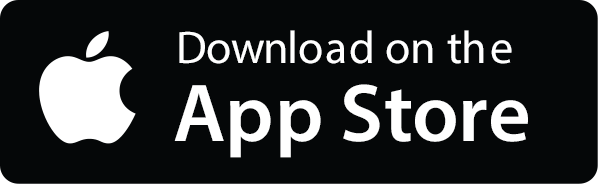
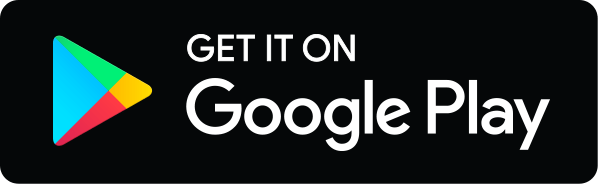