Neuro-Oncological Problems in the Intensive Care Unit
N. Scott Litofsky
Michael C. Muzinich
Introduction
Neuro-oncology encompasses the care of patients with neoplasms affecting the brain, spinal cord, and peripheral nervous system. These tumors may arise either within the nervous system itself or spread from systemic malignancies. Neuro-oncology patients may require care in an intensive care unit (ICU) at a number of different phases of their illnesses. Usually, postoperative patients with brain tumors are admitted to the ICU. Neuro-oncology patients are also admitted to the ICU if they suffer catastrophic or near catastrophic neurologic decline or if they are at high risk to suffer such a change. Lastly, neuro-oncology patients may also suffer from medical processes that require intensive care.
This chapter discusses the intensive care issues that may be encountered in neuro-oncology patients, either following their surgery or as complications of their diseases. These issues include elevated intracranial pressure (ICP), hydrocephalus, seizures, postoperative complications, spinal neoplastic disease, and medical systemic complications.
Elevated Intracranial Pressure
Elevated ICP frequently complicates the course of patients with cerebral neoplasms. Both primary and metastatic tumors in the brain can cause elevated ICP. Patients with aggressive brain
tumors often succumb as a consequence of uncontrollable elevations in ICP.
tumors often succumb as a consequence of uncontrollable elevations in ICP.
Pathophysiology
Normal intracranial pressure ranges between 5 and 15 cm H2O. This pressure is generated by the volumes of the various components contained in the “closed box” of the skull. These components include brain parenchyma, cerebrospinal fluid (CSF), extracellular water, and blood in vascular spaces. A perturbation of any of these components can increase ICP. Any additional tissue not normally present in the brain, such as a primary or metastatic tumor, or a hemorrhage associated with a tumor, can also increase ICP. While the numerical value of the ICP cannot be ascertained by neurodiagnostic images, some of the perturbations are evident on either computed tomography (CT) scan or magnetic resonance imaging (MRI).
Brain tumors can affect each intracranial component. In addition to the volume of the neoplasm itself, cerebral neoplasms can produce vasogenic edema [1], secondary to increased permeability of blood vessels within or adjacent to the tumor, thereby increasing extracellular water [2,3]. Radiographically, this edema corresponds to hypodensity on CT or hyperintensity on T2-weighted MRI around the enhancing bulk of tumor. Tumor mass, or brain parenchyma displaced by tumor, may obstruct CSF pathways, causing hydrocephalus. Hydrocephalus will be discussed in further detail in the section “Hydrocephalus.” Intravascular blood volume also can increase in patients with tumors as a result of hypoventilation. Hypoventilation occurs either related to seizure activity or ICP elevation, both of which can reduce respiratory drive. Hypoventilation increases PCO2, which causes arterial vasodilation, thereby increasing intravascular volume and ICP. This increase can cause a vicious positive feedback loop by further reducing ventilatory drive.
Signs and Symptoms
Patients can experience a variety of symptoms and signs caused by elevated ICP. These findings do not necessarily correlate with the degree of elevated pressure, though generally the higher the ICP, the more significant the neurologic findings.
As ICP increases, compression of the reticular activating system depresses the patient’s level of consciousness. These findings tend to occur sequentially, with the patient progressing from an awake and alert status to progressively more lethargic states and may eventually lead to coma.
Patients may develop a variety of cognitive changes resulting from elevated ICP. Disorientation, short-term memory loss, decreased fund of knowledge, and loss of insight and judgment can occur to varying degrees.
As increasing ICP approaches pressure of the central retinal vein, the patient will usually lose the spontaneous venous pulsations that are seen on routine funduscopic examination. Further elevation of ICP exceeding the central retinal vein pressure causes swelling of the optic disks (papilledema). Papilledema does not usually occur rapidly in the setting of elevated ICP. Usually several days of elevated ICP must ensue before papilledema is evident. A patient with long-standing papilledema may have constriction of his/her visual fields and/or decreased visual acuity.
Brain masses causing elevated ICP can cause brain shifts from one intracranial compartment to another. Usually a brain shift, also known as a “herniation,” is away from the mass causing the elevated ICP. Supratentorial masses may cause the brain to herniate inferiorly through the tentorial incisura. With a resulting central diencephalic herniation syndrome, the patient experiences simultaneous bilateral pupillary dilation from compression of the tectum, containing the Edinger–Westphal nucleus of the oculomotor nerve (CN III). A lateral cerebral mass, particularly if in the temporal lobe, forces the uncus of the temporal lobe to herniate through the incisura. This uncal herniation causes compression of CN III between the posterior cerebral artery and the superior cerebellar artery, resulting in unilateral pupillary dilation. In both herniation syndromes, the constrictive phase of the light reflex can also cease to function (unreactive pupils). Usually, though not always, decrease in the patient’s level of consciousness precedes pupillary dysfunction.
Patients can also have light-near dissociation. Pressure on the tectum can compress the retinotectal fibers that are part of the afferent limb of the pupillary light reflex; the pupil does not constrict to light appropriately. However, those fibers involved in the afferent limb of pupillary accommodation to near vision, which travel to the tectum through other pathways, are not affected. Patients, therefore, can have pupils that constrict to accommodation but not to light. This is often a very subtle sign of elevated ICP.
Double vision may also be present. The abducens nerve (CN VI), which controls abduction of the eye, has the longest intracranial course of the cranial nerves and is at highest risk of dysfunction when ICP is elevated. Diplopia is usually more pronounced with increasing lateral gaze, either unilaterally or bilaterally.
As the dura and blood vessels are stretched by elevated ICP, the patient may experience headache. Headache is frequently described as “band like” or “pressure like.” It tends to occur more commonly in the early morning and may wake the patient from sleep. While the patient is sleeping, the recumbent position decreases venous return to the heart, elevating ICP. In addition, hypoventilation that occurs during sleep will also elevate ICP, increasing the headache.
Not uncommonly, headache is associated with projectile vomiting. Vomiting occurs because of increased pressure on the area postrema.
In addition to the symptoms and signs described earlier, patients with elevated ICP often experience neurologic deficits from the compressive effects of the mass of the tumor on adjacent neural structures. These deficits can include the following: hemiparesis, aphasia, visual field deficits, hearing loss, ataxia (truncal or appendicular), and sensory loss. The presence of these findings is based on the size, location, and rapidity of growth of the mass. Slower growing tumors allow the brain to compensate; focal findings may not be evident until late in the patient’s course.
Management
Mechanical and pharmacologic therapies are available to treat elevated ICP, with expectant reduction or elimination of its signs and symptoms. Some require very minimal intervention, while others are much more intensive or invasive.
Head elevation of 30 to 45 degrees is perhaps the easiest treatment available. It increases venous drainage from the brain, thereby reducing blood volume within its intravascular compartment. Head elevation poses minimal risk to the patient. Theoretically, cerebral perfusion could be diminished, but such a reduction is negligible in a patient with normal blood pressure.
Mannitol, an osmotic diuretic, draws fluid out of the brain and into the vascular system by increasing serum osmolarity. From the vascular spaces, the fluid follows the mannitol as the kidney excretes it. Therefore, mannitol reduces intracellular and extracellular water in the brain. Furthermore, mannitol improves blood rheology; ischemic areas of brain adjacent to the tumor mass are better perfused [4]. Mannitol is
frequently given as an initial resuscitative dose of 1 gm per kg, followed by 0.25 gm per kg every 4 to 6 hours to maintain the diuresis and control ICP. Mannitol is quite effective in lowering ICP and/or reversing early cerebral herniation. It may also be used if patients have significant mass effect identified on neuroimaging studies to stabilize and improve their condition (Fig. 174.1). A number of potential risks are present with long-term mannitol use. Hypotension can occur in already hypovolemic patients. Patients can also become hyperosmolar and hypernatremic. Therefore, mannitol is usually withheld from the patient if serum osmolarity exceeds 320 mOsm per L. Lastly, there is some concern that mannitol may lose effectiveness if used continuously for more than 72 hours.
frequently given as an initial resuscitative dose of 1 gm per kg, followed by 0.25 gm per kg every 4 to 6 hours to maintain the diuresis and control ICP. Mannitol is quite effective in lowering ICP and/or reversing early cerebral herniation. It may also be used if patients have significant mass effect identified on neuroimaging studies to stabilize and improve their condition (Fig. 174.1). A number of potential risks are present with long-term mannitol use. Hypotension can occur in already hypovolemic patients. Patients can also become hyperosmolar and hypernatremic. Therefore, mannitol is usually withheld from the patient if serum osmolarity exceeds 320 mOsm per L. Lastly, there is some concern that mannitol may lose effectiveness if used continuously for more than 72 hours.
Furosemide (Lasix), a loop diuretic, rapidly reduces systemic circulating volume. Extracellular and intracellular water in the brain are drawn into the vascular system and are redistributed. Lasix also promotes venous pooling, leading to similar redistribution of fluids. While mannitol is generally used as the first-line agent, Lasix may be used at an initial resuscitative dose of 1 mg per kg in patients with cerebral herniation. Risks are minimal in this setting, as electrolyte abnormalities are unlikely to occur with only a single dose. In a patient who has had frequent vomiting and is already dehydrated, Lasix can cause hypotension from the additional hypovolemia.
Hypertonic saline enhances cerebral blood flow by increasing intravascular osmolarity that creates a gradient to move free water from the interstitial and intracellular compartments to the intravascular space. This is associated with an acute plasma expansion with hemodilution, increase in arterial blood pressure, and reduced vascular resistance [5]. Hypertonic saline has also been found to have some inotropic effects that appear to be derived from improvement in cardiac microcirculation and contractility [6]. However, significant polyuria has been observed in the acute setting which may lead to excessive diuresis and subsequent dehydration. Patients on hypertonic therapy should also have frequent blood draws every 6 hours to monitor serum osmolality and serum sodium. It is generally recommended to have a target serum sodium from 145 to 155 mmol per L and serum osmolality of less than 320 mOsm per L. Serum sodium should not increase more than 15 mmol per L daily and should not be allowed to drop more than 10 mmol per L daily to decrease the risk of central pontine myelinolysis [7].
Glucocorticosteroids can markedly improve symptoms of elevated ICP and/or mass effect in patients with cerebral neoplasms. They work by stabilizing cell membranes and reducing vasogenic edema [8,9]. Dexamethasone (Decadron) is the most commonly used glucocorticosteroid. An initial dose of 10 to 20 mg is followed by 4 to 6 mg every 4 to 6 hours, depending on the severity of the patient’s clinical condition. Solu-Medrol (100 mg initially, and then 20 to 40 mg every 4 to 6 hours) is another option. Glucocorticosteroids are the medical mainstay of brain tumor care because their effects are sustained over time. A patient with vasogenic edema from tumor may require steroids for a significant period of time. In the short term, steroids can cause hyperglycemia and exacerbate diabetes mellitus, changing the patient’s insulin requirements. Gastrointestinal hemorrhage or ulceration can occur; H2 blockers, such as Pepcid, Nexium, or Zantac, are frequently given prophylactically. The stimulatory effect of steroids frequently disrupts sleep. Long-term use of steroids may be associated with proximal muscle weakness, avascular necrosis of the femoral head, easy bruising, and other findings of Cushing’s syndrome.
In contrast to cerebral vasodilation caused by hypoventilation, hypocarbia from hyperventilation causes cerebral vasoconstriction, which reduces the arterial intravascular blood volume within the brain. Hyperventilation can therefore rapidly reduce ICP and reverse a cerebral herniation syndrome. Although initial hyperventilation can be performed with an AMBU bag valve mask, sustained hyperventilation requires endotracheal intubation and mechanical ventilation of the patient. Moderation of hyperventilation is necessary because at PCO2 less than 25 mm Hg, cerebral ischemia may result from profound vasoconstriction. A vasodilatory rebound from hyperventilation occurs after approximately 24 hours, thereby negating its positive effects if hyperventilation is used chronically [10].
One of the most effective means of rapidly reducing ICP is to drain CSF. Such a maneuver is effective whether or not the patient has hydrocephalus. In a patient with a brain tumor, the safest method of draining CSF is to place a ventriculostomy, a catheter usually passed into the frontal horn of the lateral ventricle via a small hole drilled through the skull. The procedure can be performed by a neurosurgeon at the bedside. After placement of a ventriculostomy, drainage of CSF into a bag at bedside can reduce ICP. The catheter can also be coupled to a pressure transducer so that ICP can be measured. Usually, CSF is drained if ICP exceeds 15 to 20 mm Hg. The risks of the procedure include hemorrhage and infection. Therefore, coagulation studies are appropriate before the procedure is done, especially in patients who have received recent chemotherapy. The risk of infection increases the longer the catheter remains in place. Sometimes, prophylactic antibiotics are used.
Regardless of what means are necessary to stabilize and/or resuscitate the patient, the best means of controlling ICP in long term is to remove the tumor if possible. Unfortunately, some tumors are unresectable. Gliomas or metastases involving the thalamus or basal ganglia are generally not resected, except in unusual circumstances. In these instances, medical management is necessary to control ICP until adjuvant therapy, such as radiation therapy, can shrink the tumor and reduce its edema-producing capabilities. The same rationale applies to patients with multifocal cerebral masses; patients with more than one metastasis do not usually have multiple operations to resect each tumor, especially if symptoms are controllable with steroids. On the other hand, if the tumor is resectable, its removal can relieve the brain of the extra mass, relieve obstruction to the flow of CSF, and reduce vasogenic edema. In addition to relieving the signs and symptoms of elevated ICP, tumor resection can also relieve the effects of compression on the surrounding brain, improving lateralizing findings. Some tumors can be removed completely. These include meningiomas, vestibular schwannomas, craniopharyngiomas, pituitary adenomas, and metastatic tumors. Microscopic disease may still be present in the tumor bed, particularly in the case of metastases or craniopharyngioma, which may require adjuvant therapy, but ICP can be well controlled. Primary glial neoplasms, however, cannot be completely removed in most cases. The bulk of tumor can be resected, and postoperative neurodiagnostic images may show no residual tumor, but most of these tumors have infiltrating fingers of tumor still present. Even so, removing tumor bulk can alleviate elevated ICP; edema can sometimes be exacerbated with only partial resection, so caution is required.
Hydrocephalus
Brain tumors often can cause hydrocephalus, a situation in which the patient has an increased volume of CSF under increased pressure. Hydrocephalus is typically associated with enlargement of the ventricular system (or a portion thereof) and compression of the normal brain parenchyma. A patient with hydrocephalus may require urgent or emergent intensive care monitoring and treatment. Hydrocephalus is a special case of elevated ICP and warrants separate discussion.
Etiology
Hydrocephalus can occur from a variety of mechanisms in patients with brain tumors. It is as important to identify the etiology of the hydrocephalus as its presence because the definitive treatment of hydrocephalus will be based on its mechanism of formation. Some tumors, as discussed later, are more likely to be associated with certain mechanisms of hydrocephalus than others.
Leptomeningeal infiltration by tumor cells in the subarachnoid space can prevent the absorption of CSF by the arachnoid granulations, either by occluding the granulations or preventing the flow of CSF from the outlet foramen of the fourth ventricle around the dorsolateral convexities to the granulations. Metastatic tumors from the lung, breast, lymphoma, and leukemia are the most frequently involved systemic tumors; primary tumors behaving in this fashion include primitive neuroectodermal tumors (i.e., medulloblastoma), ependymoblastoma, and glioblastoma multiforme. A patient with carcinomatous meningitis will frequently have a stiff neck or cranial neuropathy in addition to symptoms and signs of elevated ICP.
Large extra-axial “benign” tumors, usually in the posterior fossa, can cause hydrocephalus (Fig. 174.2). These tumors include those in the cerebellopontine angle, such as meningioma or vestibular schwannoma. These tumors displace the cerebellar hemisphere and obstruct the fourth ventricle to prevent adequate circulation of CSF. Rarely a choroid plexus papilloma can emerge from the foramen of Luschka and similarly compress the cerebellar hemisphere. Meningiomas of the clivus or tentorium can also displace CSF pathways with resulting hydrocephalus.
Some tumors may originate in a ventricle or protrude into a ventricle and occlude CSF pathways, thus producing hydrocephalus. These tumors include medulloblastoma, ependymoma, choroid plexus papilloma, intraventricular meningioma, colloid cyst, giant cell astrocytoma of tuberous sclerosis, and pineal region tumors.
Parenchymal tumors often can occlude CSF pathways. Primary or metastatic tumors in the thalamus or basal ganglia can displace brain parenchyma and occlude the foramen of Monro or the third ventricle [11]. Tumors in the pineal region may occlude the posterior third ventricle or cerebral aqueduct (Fig. 174.3). Brain stem gliomas or tumors in the cerebellar hemispheres can compress the fourth ventricle [12].
Symptoms and Signs
The clinical picture of a patient with hydrocephalus is frequently the same as that of a patient with elevated ICP. In fact, hydrocephalus must be considered in the differential diagnosis for causes of elevated ICP. Patients with midline masses or carcinomatous meningitis usually do not have lateralizing neurologic deficits such as hemiparesis. Those patients with unilateral brain masses may have lateralizing deficits from compression of the previously normally, but marginally, functioning brain by the progressive hydrocephalus.

Full access? Get Clinical Tree
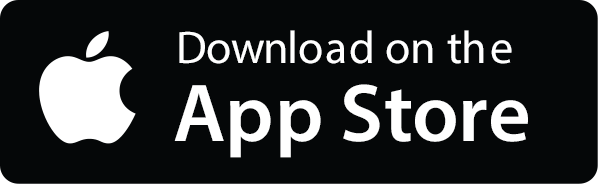
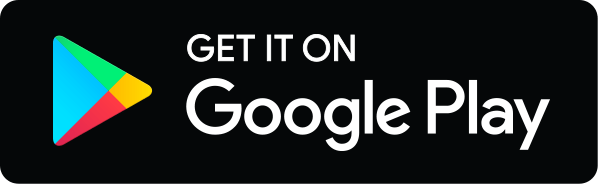